Abstract
Context: Medicinal plants are well known for their use in traditional folk medicine as treatments for many diseases including infectious diseases.
Objective: Six Brazilian medicinal plant species were subjected to an antiviral screening bioassay to investigate and evaluate their biological activities against five viruses: bovine herpesvirus type 5 (BHV-5), avian metapneumovirus (aMPV), murine hepatitis virus type 3, porcine parvovirus and bovine respiratory syncytial virus.
Materials and methods: The antiviral activity was determined by a titration technique that depends on the ability of plant extract dilutions (25 or 2.5 µg/mL) to inhibit the viral induced cytopathic effect and the extracts’ inhibition percentage (IP).
Results: Two medicinal plant species showed potential antiviral activity. The Aniba rosaeodora Ducke (Lauraceae) extract had the best results, with 90% inhibition of viral growth at 2.5 µg/mL when the extract was added during the replication period of the aMPV infection cycle. The Maytenus ilicifolia (Schrad.) Planch. (Celastraceae) extracts at a concentration of 2.5 µg/mL exhibited antiviral activity during the attachment phase of BHV-5 (IP = 100%).
Discussion and conclusion: The biomonitored fractionation of the active extracts from M. ilicifolia and A. rosaeodora could be a potential tool for identifying their active compounds and determining the exact mechanism of action.
Introduction
An increasing number of studies are currently focused on natural sources as means of solving human problems including those in the medical field. Ethnomedicine is a science that studies the ancient practice of the medical use of plants. We have records of ethnomedicine from as early as 2600 BC when the oils of cedar [Cedrus spp. (Pinaceae)], cypress [Cupressus sempervirens L. (Cupressaceae)], licorice liqueur ([Glycyrrhiza glabra L. (Leguminosae)]) and poppy [Papaver somniferum L. (Papaveraceae)] were used to treat coughing due to parasitic infection. Over 80% of the postgenomically produced drugs have a natural origin or were inspired by natural products. Natural plant products have traditionally provided 30–40% of the modern antimicrobial or anticancer drugs for the pharmaceutical industry, but science has analysed only a small fraction of potentially useful species in its efforts to design more antimicrobial drugs from plants (CitationHarvey, 2007).
Polysaccharides, flavonoids, terpenes, alkaloids, phenols and amino acids are among the plant-derived antiviral compounds that are being used in traditional medicine, and these have been shown to inhibit the replication of some viruses such as herpes simplex, human immunodeficiency virus, hepatitis B and severe acute respiratory syndrome (CitationCos et al., 2006; CitationMukhtar et al., 2008; Bufalo et al., 2009).
In modern medicine, there are approximately 37 licensed antiviral drugs on the market (CitationClerq, 2004), but many diseases caused by viruses are untreatable; viral resistance, latency and other recurring difficulties in treatment are also of concern (CitationChattopadhyay & Naik, 2007). The search for new antimicrobial drugs should therefore be intensified and all possible strategies should be deployed (CitationCos et al., 2006; CitationGautam et al., 2007).
New substances can be screened to discover new antiviral agents, as is widely done for cancer drug discovery. To ensure the efficiency of screening, it is necessary to improve the methodology used so that screening is rapid, easy, low-cost, reproducible and capable of standardization and adaptation to various situations. Additionally, the experimental model should mimic the physiological characteristics of the process in the human body, reducing the number of false positives (CitationSmee et al., 2002; CitationCos et al., 2006).
The visual microscopic analysis of cytopathic effects (CPE), colorimetric assays (crystal violet, MTT and neutral red) and fluorometric assays (AlamarBlue®, Bisbenzidine Rhodamine 6G) can be used to screen antiviral compounds (CitationAbou-Karam & Shier, 1990; CitationSmee et al., 2002; CitationJassim & Naji, 2003).
To screen for antiviral substances in plant extracts, it is necessary to choose animal viruses that contain the main differentiating features of viral morphology. There are two main groups for basic viral structure: viruses with no envelope and those with an envelope. In addition, there are viruses with RNA and DNA genomes. Viruses representing all of these structural differences were chosen for screening in the present study to assess the activities of the extracts toward most viral enzymes. Important animal viruses with RNA and DNA genomes that are enveloped and non-enveloped were used: bovine herpesvirus type 5 (BHV-5), avian metapneumovirus (aMPV), murine hepatitis virus type 3 (MHV-3), porcine parvovirus (PPV) and bovine respiratory syncytial virus (BRSV).
BHV-5 is the causal agent of necrotizing meningoencephalitis in young cattle. This virus has a marked neurotropism, frequently leading to fatal disease. BHV-5 has a limited geographic distribution with a high incidence of neurologic disease in South American countries, mainly Argentina and Brazil (CitationDel Médico Zajac et al., 2010).
Avian metapneumovirus (aMPV) is responsible for turkey rhinotracheitis. In chickens, aMPV is involved in the etiology of multifactorial diseases such as Swollen Head Syndrome. This virus causes serious economic losses in the poultry industry (CitationBroor & Bharaj, 2007). BRSV is a major cause of respiratory disease and is a major contributor to the BRD complex, which has caused more than one billion dollars in losses. BRSV infects the upper and lower respiratory tract and is shed in nasal secretions. These two viruses are closely related to the human respiratory syncytial virus (CitationBrodersen, 2010).
Mouse hepatitis viruses (MHVs) are a group of coronaviruses that cause unapparent infection or diseases in mice. The symptoms of these viruses include diarrhea, hepatitis, splenolysis, immunological dysfunction, and acute and chronic neurological disorders. MHV spreads effectively from mouse to mouse, causing frequent enzootics in colonies of laboratory mice (CitationKim et al., 2010).
PPV is one of the most important pathogens responsible for reproductive failure in swine, leading to stillbirth, mummification, embryonic death and infertility. PPV occurs worldwide, with variable prevalence rates reported (CitationWilhelm et al., 2006).
Materials and methods
Plant material
Baccharis dracunculifolia D. C. (Asteraceae), Pfaffia glomerata (Sprengel) Pedersen (Amaranthaceae) and Maytenus ilicifolia (Schrad.) Planch. (Celastraceae) were harvested from the Chemical, Biological and Agricultural Pluridisciplinary Research Center of the State University of Campinas, Brazil (CPQBA-UNICAMP). The samples were deposited in the herbarium of CPQBA under voucher number (). Bursera aloexylon (Shiede ex. Schlecht) Engler (Burseraceae) leaves were harvested from the Medicinal and Aromatic Plant Collection of CPQBA-UNICAMP. The plant materials were collected in January 2006. Dr. Glyn Mara Figueira was responsible for the herbarium.
Table 1. Popular use of medicinal plants.
Dried and ground plant materials from P. glomerata (roots) and M. ilicifolia (leaves) (100 g) were extracted by maceration with 1000 mL of 70% ethanol P.A. for 72 h (CitationQueiroga et al., 2000). The volatile oils from the leaves of B. dracunculifolia and B. aloexylon were obtained by hydrodistillation in a Clevenger system for 1 h (CitationQueiroga et al., 2007). Copaifera langsdorffi Desf. (Fabaceae) oil resin was obtained directly from tree trunks in Bahia State as an exudate. The Aniba rosaeodora Ducke (Lauraceae) (pau Rosa) was collected from a specimen growing in the Zoobotanical Park of the Emilio Goeldi Museum in the state of Pará, Brazil. A voucher is deposited in the herbarium of the same institution. The trunk wood sample was dried at room temperature (5 days), ground and submitted to hydrodistillation using a Clevenger type apparatus (3 h) (CitationSampaio et al., 2012). The quality was confirmed by GC/EM analysis.
Viruses and cell lines
All cell lines () were propagated in monolayer cultures using minimal essential medium with Earle’s salts supplemented with 10% fetal bovine serum (FBS). The cells were passaged at 1:2 to 1:10 dilutions using 0.05% trypsin with 0.02% EDTA according to conventional procedures. It was necessary to use medium without FBS when the viruses were incubated together with the cells.
Table 2. Viruses and cells used in the antiviral screening.
Cytotoxic effects on cells
The cell suspension (100 µL) was seeded in 96-well culture plates at a density of 1 × 105 cells/mL. The microtiter plates containing cells were pre-incubated for 24 h at 37°C to allow for stabilization prior to the addition (100 µL) of the extract samples at four concentrations (0.25, 2.5, 25, and 250 µg/mL) (CitationKohn et al., 2006). The maximum nontoxic concentration (MNTC) for each sample was determined microscopically by observing the morphological changes of the cells at 24, 48 and 72 h of incubation. The results were obtained with the sulforhodamine B (SRB) assay after 72 h.
Sulforhodamine B assay
The SRB assay is used to determine cellular density by measuring the amount of cellular protein. The method described here has been optimized for the toxicity screening of compounds to adherent cells in a 96-well format. The SRB assay is therefore an efficient and highly cost-effective method for screening. The SRB assay was performed as described by CitationKohn et al. (2006). Briefly, the cells were fixed with 50% trichloroacetic acid at 4°C (50 µL per well, 10% final concentration) for 1 h. The supernatant was then discarded, and the plates were washed five times with tap water. The cells were stained with 0.4% SRB in 1% acetic acid (50 µL per well) for 30 min and subsequently washed four times with 1% acetic acid to remove unbound dye. The plates were air-dried, and the protein-bound dye was solubilized with 150 µL (100 mM) of Trizma buffer. The resulting optical density was read in a multiwell plate reader at 540 nm (CitationKohn et al., 2006).
Titration of viruses
The cells were seeded in 96-well culture plates at a density of 1 × 105 cells/mL and incubated at 37°C in a humidified atmosphere containing CO2 for 24 h. Serial dilutions of the viral stocks were prepared, and the cells were infected with the diluted virus. After an additional incubation (1–2 days), the CPE were recorded. The 50% tissue-culture infective dose (TCID50) per mL was calculated as described by CitationReed and Munch (1938).
Antiviral activity
The antiviral activity of the extracts was determined by their inhibition of CPE. All of the experiments were performed in triplicate. Briefly, to evaluate the inhibition, the cells were seeded in 96-well culture plates. After 24 h of incubation, the medium was replaced with 100 µL of DMEM containing the MNTC of the extracts, 50 µL of the logarithmic viral dilutions were added in quadruplicate and the plates were incubated for 3 days. The controls consisted of untreated infected (viral titer), treated non-infected (extract control), and untreated non-infected cells. The viral titers were determined by the 50% infective dose in tissue culture (TCID50 µL) assay (CitationReed & Muench, 1938). The antiviral activity of each extract was determined by comparing the logarithmic reduction factor (log10) of the viral titer with the untreated infected controls. The values were expressed as titer (TCID50 µL) and inhibition percentage (IP) as described in CitationKoseki et al. (1990). The IP was calculated by the formula: (IP) = (1 – T/C) × 100, where T is the antilog of the extract-treated viral titers and C is the antilog of the control (without extract) viral titers. IP was considered significant when the percentage was greater than or equal to 98%.
The antiviral activity test was initially evaluated with a single MNTC extract and different viral concentrations. The extract was considered active if there was a 1.5 log decrease in the viral titer. To confirm activity, a concentration-response curve using different concentrations of the extract in the presence of 100 TCID/mL was plotted using the MTT assay, and the antiviral concentration 50% (EC50) was calculated from this. Briefly, 20 µL of an MTT solution at 5 mg/mL in PBS was added to each well of the 96-well culture plates at each time point. Following 4 h of incubation, 100 µL of DMSO was added to each well and mixed thoroughly to dissolve the dark-blue formazan crystals formed by the surviving cells. Thereafter, the plates were read on an ELISA reader (Molecular Devices SpectraMax Plus) at 540 nm (CitationMosmann, 1983; CitationScudiero et al., 1988).
Data analysis
The 50% cytotoxic (CC50) and 50% inhibition concentrations (IC50) were calculated from the concentration-response curves. The results were obtained from triplicate assays with at least five extract concentrations. The cytotoxic percentages were calculated as [(A – B)/A] × 100, where A and B are the OD 540 nm of untreated and treated cells, respectively. The protection percentages were calculated as [(A − B) × 100/(C − B)], where A, B and C indicate the absorbance of the extracts/fractions, the virus and the cell controls, respectively. Each EC50 value obtained was defined as the effective concentration that reduced the absorbance of the infected cells to 50% when compared with the cell and virus controls. The CC50 and IC50 for each compound were obtained from dose-response curves (not shown). The CC50 and IC50 are the average of three assays that contained five concentrations within the inhibitory range of the compounds. The therapeutic index (i.e., selective index) was defined as CC50/IC50.
The potential stage in the viral infection cycle
The cells and viruses were incubated with active extracts at different stages of the viral infection cycle to determine the mode of antiviral action. The cells were pretreated with samples before viral infection, the viruses were incubated with samples before infecting the cells, and the cells were infected with the virus before the addition of samples. The extracts were used at their maximum non-cytotoxic concentrations.
Virucidal action
To evaluate possible extracellular viral inactivation by the extracts, equal volumes (100 µL) of the 10-fold serially diluted viral suspension and the MNTC of the extracts were mixed and incubated for 1 h at 37°C. Each mixture was then added to the cell monolayer, and the infectious titers were compared with controls.
Statistical analysis
The results were expressed as the mean ± S.E.M. The selectivity index (SI) was calculated as the ratio of CC50 to EC50. The ability of the tested extracts to inhibit viral replication was compared with the control group using the Student’s t-test. A p ≤ 0.05 was considered a significant result.
Results
Cytotoxic effects on cells
The MNTCs of the extracts were determined. The concentrations used in the antiviral assays were 25 µg/mL for M. ilicifolia, P. glomerata, B. aloexylon, C. langsdorffi, and B. dracunculifolia and 2.5 µg/mL for A. rosaeodora.
Antiviral activity of extracts
The antiviral activity of each extract listed in was initially evaluated in a standard titer assay where the nontoxic extract concentrations were added at the same time as the virus. This initial analysis identified which of the many extracts tested have antiviral activity against different viruses. The antiviral activity of each extract was determined as the logarithmic reduction factor (log10) of the viral titer compared with the untreated infected controls. The values were expressed as titer (TCID50 µL) and IP, as described previously. The results were considered active at IPs higher than 97%. Two medicinal plant species showed potential antiviral activity. The best results were obtained from the A. rosaeodora extract (2.5 µg/mL), which exhibited a 98% IP for aMPV. The Maytenus extract (25 µg/mL) exhibited 99% inhibitory activity toward herpesvirus type 5.
These results show that the extracts exhibit increasing antiviral activity against the aMPV virus sample 668 isolated in Brazil and HBV-5. None of the plants studied were active against parvovirus, MHV-3 (DNA-genome virus) or BRSV (RNA-genome virus).
The potential stage in the viral infection cycle
To identify the stage of viral replication during which the active extracts could be exerting their effects, three different treatments were carried out. The cells were infected with virus, and the extract was added after 1 h to evaluate the viral replication phase. The viruses were pretreated with the extract before infecting the cells (virus inactivation), and the extract was added to the cells before viral infection to evaluate any effects on viral adsorption. The extracts were used at their MNTC in all experiments, and the results are shown in .
Figure 1. Mode of inhibitory effect of Maytenus ilicifolia against HBV-5. Virus and cells were treated with the MNTC of extract. Values were presented as mean ± S.E.M. of three independent experiments.
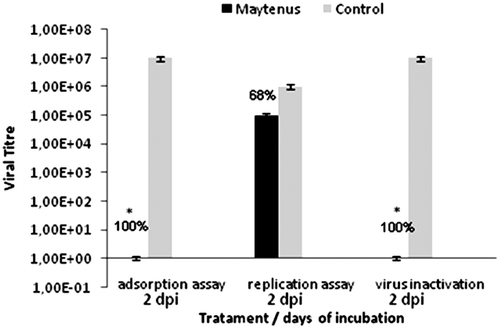
The analysis of this assay showed that A. rosaeodora oil inhibited 90% of the viral growth after treatment, suggesting that this extract exerts its effects during the viral replication. This effect should be studied further to determine its mechanism. Maytenus antiviral activity was not observed at this stage, but rather during the adsorption phase, where it showed 90% inhibition of viral adsorption.
The 50% cytotoxic concentrations (CC50) of the two species are close to 100 µg/mL, and the selectivity index was higher for A. rosaeodora oil ().
Table 3. The CC50, IC50 and SI extracts active.
Discussion
In vitro assays usually exploit a virus’s ability to infect and replicate in specific cell lines in culture systems. The cell culture system provides a rapid and less cumbersome method to grow viruses at higher titers, produce vaccine strain cultures, study reverse genetics and test antiviral compounds. An example of the cell lines that are characterized and routinely used for common viruses is provided in .
Indirect assays are usually the first step in screening a large number of compounds to evaluate their antiviral activity. The inhibition of CPE in the presence of a test compound could be due to the inhibition of viral replication. One of the inherent drawbacks of in vitro antiviral testing is the environmental sensitivity of the cell lines in culture (CitationSmee et al., 2002). Preparations that exert antiviral effects in vivo may not be detected in vitro because of the extremely low concentrations of extract tolerated by cells in the artificial system (CitationMcCutcheon et al., 1995). Even with this limitation, two of the six extracts screened exhibited some antiviral activity.
The analysis of the methodology used to screen the different extracts was effective because it revealed extracts with activities toward different types of viruses. Our results showed two plants with potential antiviral activity (M. ilicifolia for HBV-5 and A. rosaeodora for aMPV) and specific activities for one phase of the viral replication cycle. Further studies are necessary to identify the substances responsible for these activities.
The first stage of screening is necessary to determine the extract’s MNTC, or MNTC with regard to the cells. This step is critical because the virus is an intracellular parasite that uses the cell’s machinery to replicate, and it must be ensured that the virus has the ideal conditions for growth. Because of this, each extract’s cytotoxicity was tested on different kinds of cells. The results of this assay revealed that M. ilicifolia had the lowest cytotoxicity and A. rosaeodora had the highest cytotoxicity of the extracts tested; however, the cytotoxic range of all the extracts was in the same order of magnitude.
The plants studied were effective against two of the five types of virus tested. M. ilicifolia and A. rosaeodora oil (Lin) showed the best antiviral potential, exhibiting 99% antiviral activity against HBV-5 and 98% antiviral activity against aMPV, respectively.
None of the plants in the study yielded extracts that were active against the parvovirus (DNA-genome virus), BRSV (RNA-genome virus) or MHV-3 (mouse hepatitis). Therefore, further studies with other plants that have different chemical compositions should be conducted, with the goal of finding a chemical class that can act against these viruses. The Lin aMPV antiviral activity tests, in which the extracts were added following infection, were unable to detect any activity and suggested that the inhibition of viral replication may occur during the replication phase of the infection (). To test this hypothesis, the cells were treated with the extracts at the MNTC and incubated for 1 h after infection with the virus. The antiviral activity observed in this assay indicated that the A. rosaeodora oil was active in the replication phase, perhaps due to the presence of monoterpene linalool in this oil.
Figure 2. Mode of inhibitory effect of Aniba rosaeodora oil against aMPV. Virus and cells were treated with the MNTC of oil. Values were presented as mean ± S.E.M. of three independent experiments.
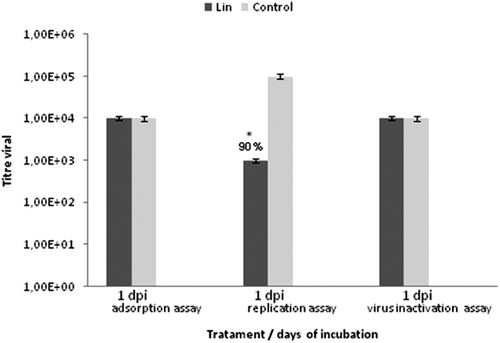
In the assay to evaluate the Maytenus extract activity against bovine herpes virus after different types of treatment (), activity was only observed when the virus was outside of the cell (adsorption phase). No activity was observed in the replication phase (pretreatment of the cell with the virus), suggesting that this extract could be acting during adsorption by inhibiting the receptor responsible for adhesion. The fact that the extracts retained activity under these conditions indicates that the extracts are stably bound to viral receptors on the cell surface. However, these results could also be a result of other inhibitory mechanisms; for example, the relatively high levels of flavonoid glycosides present in this extract may be responsible for this activity.
Pretreating the cells with the Lin extract had no effect on the production of infectious aMPV virus or the viral titer. The best results (IP = 90%) were found when the extract was added during the replication period of the aMPV infection cycle. The antiviral activity of Maytenus extracts was observed during the herpesvirus attachment phase (IP = 100%). The same IP was observed during viral inactivation but not during the replication period (IP = 68%). These results suggest that the M. ilicifolia extract interferes with the structure of the virus in ways that impede its adsorption, as extract activity is lost when added after cellular infection. The A. rosaeodora extracts must be acting on some enzyme during the transcription of viral proteins, as activity was only exhibited in the assay targeting the viral replication phase.
Conclusion
Despite continuous advances in antiviral therapy, viral diseases have become the leading cause of death globally. As intracellular parasites, viruses are dependent on the metabolic pathways of the host cell for their replication. Because viruses and hosts are intimately connected, designing effective antivirals that will attack viral enzymes or replication without affecting the host cell has proven to be difficult.
The results of the present study provide further support for the potential of medicinal plants, which possibly represent a reservoir of pharmacologically active substances. The biomonitored fractionation of the M.ilicifolia and A. rosaeodora active extracts could be a potential tool for identifying their active compounds and determining the exact mechanisms of action for these extracts.
Declaration of interest
The authors would like to thank the funding organizations Fundação de Amparo à Pesquisa do Estado de São Paulo (FAPESP) for the financial support of this work and the others. The authors declare no conflicts of interest.
References
- Abou-Karam M, Shier WT. (1990). A simplified plaque reduction assay for antiviral agents from plants. Demonstration of frequent occurrence of antiviral activity in higher plants. J Nat Prod, 53, 340–344.
- Arns CW, Campalans J, Costa SC, Domingues HG, D’Arce RC, Almeida RS, Coswig LT. (2003). Characterization of bovine respiratory syncytial virus isolated in Brazil. Braz J Med Biol Res, 36, 213–218.
- Brodersen BW. (2010). Bovine respiratory syncytial virus. Vet Clin North Am Food Anim Pract, 26, 323–333.
- Broor S, Bharaj P. (2007). Avian and human metapneumovirus. Ann N Y Acad Sci, 1102, 66–85.
- Búfalo MC, Figueiredo AS, de Sousa JP, Candeias JM, Bastos JK, Sforcin JM. (2009). Poliovirus activity of Baccharis dracunculifolia and propolis by cell viability determination and real-time PCR. J Appl Microbiol, 107, 1669–1680.
- Calgaroto NS, Castro GY, Cargnelutti D, Pereira LB, Gonçalves JF, Rossato LV, Antes FG, Dressler VL, Flores EM, Schetinger MR, Nicoloso FT. (2010). Antioxidant system activation by mercury in Pfaffia glomerata plantlets. Biometals, 23, 295–305.
- Chattopadhyay D, Naik TN. (2007). Antivirals of ethnomedicinal origin: Structure-activity relationship and scope. Mini Rev Med Chem, 7, 275–301.
- Clerq E. (2004). Antiviral drugs in current clinical use. J Clin Virol, 30, 115–133.
- Cos P, Vlietinck AJ, Berghe DV, Maes L. (2006). Anti-infective potential of natural products: How to develop a stronger in vitro ‘proof-of-concept’. J Ethnopharmacol, 106, 290–302.
- Costa-Lotufo LV, Cunha GM, Farias PA, Viana GS, Cunha KM, Pessoa C, Moraes MO, Silveira ER, Gramosa NV, Rao VS. (2002). The cytotoxic and embryotoxic effects of kaurenoic acid, a diterpene isolated from Copaifera langsdorffii oleo-resin. Toxicon, 40, 1231–1234.
- D’Arce RC, Coswig LT, Almeida RS, Trevisol IM, Monteiro MC, Rossini LI, Di Fabio J, Hafez HM, Arns CW. (2005). Subtyping of new Brazilian avian metapneumovirus isolates from chickens and turkeys by reverse transcriptase-nested-polymerase chain reaction. Avian Pathol, 34, 133–136.
- de Almeida RN, Araújo DA, Gonçalves JC, Montenegro FC, de Sousa DP, Leite JR, Mattei R, Benedito MA, de Carvalho JG, Cruz JS, Maia JG. (2009). Rosewood oil induces sedation and inhibits compound action potential in rodents. J Ethnopharmacol, 124, 440–443.
- Del Médico Zajac MP, Ladelfa MF, Kotsias F, Muylkens B, Thiry J, Thiry E, Romera SA. (2010). Biology of bovine herpesvirus 5. Vet J, 184, 138–145.
- Flores EF, Donis RO. (1995). Isolation of a mutant MDBK cell line resistant to bovine viral diarrhea virus infection due to a block in viral entry. Virology, 208, 565–575.
- Gautam R, Saklani A, Jachak SM. (2007). Indian medicinal plants as a source of antimycobacterial agents. J Ethnopharmacol, 110, 200–234.
- Harvey AL. (2007). Natural products as a screening resource. Curr Opin Chem Biol, 11, 480–484.
- Jassim SA, Naji MA. (2003). Novel antiviral agents: A medicinal plant perspective. J Appl Microbiol, 95, 412–427.
- Kim HY, Eo EY, Park H, Kim YC, Park S, Shin HJ, Kim K. (2010). Medicinal herbal extracts of Sophorae radix, Acanthopanacis cortex, Sanguisorbae radix and Torilis fructus inhibit coronavirus replication in vitro. Antivir Ther (Lond), 15, 697–709.
- Kohn LK, Pavam CH, Veronese D, Coelho F, De Carvalho JE, Almeida WP. (2006). Antiproliferative effect of Baylis-Hillman adducts and a new phthalide derivative on human tumor cell lines. Eur J Med Chem, 41, 738–744.
- Koseki I, Simoni IC, Nakamura IT, Noronha AB, Costa SS. (1990). Antiviral activity of plants extracts against aphtovirus, pseudorabies virus and pestivirus in cell cultures. Microbios Letters, 44, 19–30.
- Leitão DP, Filho AA, Polizello AC, Bastos JK, Spadaro AC. (2004). Comparative evaluation of in-vitro effects of Brazilian green propolis and Baccharis dracunculifolia extracts on cariogenic factors of Streptococcus mutans. Biol Pharm Bull, 27, 1834–1839.
- McCutcheon AR, Roberts TE, Gibbons E, Ellis SM, Babiuk LA, Hancock RE, Towers GH. (1995). Antiviral screening of British Columbian medicinal plants. J Ethnopharmacol, 49, 101–110.
- Mosmann T. (1983). Rapid colorimetric assay for cellular growth and survival: Application to proliferation and cytotoxicity assays. J Immunol Methods, 65, 55–63.
- Mukhtar M, Arshad M, Ahmad M, Pomerantz RJ, Wigdahl B, Parveen Z. (2008). Antiviral potentials of medicinal plants. Virus Res, 131, 111–120.
- Nishimoto N, Nakai S, Takagi N, Hayashi S, Takemoto T, Odashima S, Kizu H, Wada Y. (1984). Pfaffosides and Nortriterpenoid Saponins from Pfaffia Paniculata. Phytochemistry, 23, 139–142.
- Queiroga CL, Duarte MC, Ribeiro BB, de Magalhães PM. (2007). Linalool production from the leaves of Bursera aloexylon and its antimicrobial activity. Fitoterapia, 78, 327–328.
- Queiroga CL, Silva GF, Dias PC, Possenti A, de Carvalho JE. (2000). Evaluation of the antiulcerogenic activity of friedelan-3β-ol and friedelin isolated from Maytenus ilicifolia (Celastraceae). J Ethnopharmacol, 72, 465–468.
- Reed LJ, Muench H. (1938). A simple method of estimating fifty percent endpoints. Am J of Hyg, 27, 493–497.
- Sampaio Lde F, Maia JG, de Parijós AM, de Souza RZ, Barata LE. (2012). Linalool from rosewood (Aniba rosaeodora Ducke) oil inhibits adenylate cyclase in the retina, contributing to understanding its biological activity. Phytother Res, 26, 73–77.
- Scudiero DA, Shoemaker RH, Paull KD, Monks A, Tierney S, Nofziger TH, Currens MJ, Seniff D, Boyd MR. (1988). Evaluation of a soluble tetrazolium/formazan assay for cell growth and drug sensitivity in culture using human and other tumor cell lines. Cancer Res, 48, 4827–4833.
- Simic A, Sokovic MD, Ristic M, Grujic-Jovanovic S, Vukojevic J, Marin PD. (2004). The chemical composition of some Lauraceae essential oils and their antifungal activities. Phytother Res, 18, 713–717.
- Smee DF, Morrison AC, Barnard DL, Sidwell RW. (2002). Comparison of colorimetric, fluorometric, and visual methods for determining anti-influenza (H1N1 and H3N2) virus activities and toxicities of compounds. J Virol Methods, 106, 71–79.
- Wilhelm S, Zimmermann P, Selbitz HJ, Truyen U. (2006). Real-time PCR protocol for the detection of porcine parvovirus in field samples. J Virol Methods, 134, 257–260.