Abstract
Context: There are 33 Geranium species growing in Turkey characterized by the presence of polyphenolic compounds. Some Geranium (Geraniaceae) species are used as antidiabetics, hemostatics, antihemorrhoidals, antidiarrheics and for the treatment of pain, fevers, and gastrointestinal ailments, or are consumed as food.
Objective: The in vitro antioxidant activity and antihemolytic effect of ethyl acetate (EtOAc), n-butanol (BuOH), methanol (MeOH) and water extracts of Geranium tuberosum L. subsp. tuberosum (Geraniaceae), a medicinal food plant, have been evaluated.
Materials and methods: The two antioxidant enzyme activities of human erythrocyte, namely superoxide dismutase (SOD) and catalase (CAT), after in vitro incubation with the extracts, were examined in order to see whether the observed effects are related to altered enzymatic efficiency. Reduced glutathione (GSH) levels were also measured as oxidative stress marker. Antihemolytic activity of extracts was shown by hemolysis assay in erythrocytes. Furthermore, total phenolic content of extracts was measured by Folin–Ciocalteu method.
Results: All extracts enhanced GSH levels, and the activity of SOD and CAT. The EtOAc extracts seems to be the most potent antioxidant at 100 µg/mL (SOD activity 173.736 ± 8.33, CAT activity 133.218 ± 3.31, GSH level 2.264 ± 2.21). However, apart from the MeOH extracts at 100 µg/mL (68.699 ± 3.93), they didn’t increase the resistance of erythrocytes to H2O2 induced cytotoxicity. Therefore, while a significant antioxidant effect was observed in these samples, antihemolytic effect was not determined.
Discussion and conclusion: The title plant has shown high antioxidant activity without cytotoxicity up to 100 µg/mL, thus could be a potent source as natural antioxidant.
Introduction
Using antioxidants against oxidative stress in humans has attracted considerable interest for the last two decades. An approach could be to use natural combination of various antioxidants as herbal mixtures or extracts. Oxidative stress plays an important role in many physiological and pathological phenomena. It is known that free oxygen radicals are responsible for the development of many diseases including carcinogenesis, atherosclerosis, diabetes, and heart diseases and directly induce cell damage by modifying proteins, lipids or DNA (CitationHalliwell & Gutteridge, 1989; CitationHalliwell, 1994).
Although synthetic antioxidants have often been used to protect against free radicals by scavenging reactive oxygen or ending radical chain reactions, recent health concerns draw much attention to the use of natural antioxidants. Aromatic and medicinal plants are a good source of bioactive compounds which may be valuable ingredients for different applications, including pharmaceuticals, functional foods and food supplements (CitationRice-Evans & Miller, 1997; CitationBaytop, 1999; CitationRobards et al., 1999; CitationLu & Foo, 2000).
Some Geranium species have been employed in folk medicine for their tonic, diuretic, antidiabetic, antidiarrheal, and antihemorrhoidal properties. Moreover, they have been used in the treatment of fever, tonsillitis, cough, whooping cough, urticaria, dysentery, kidney pain, and gastrointestinal ailments. These traditional usages and the rich polyphenolic content of Geranium species make these plants interesting from the viewpoint of biological and toxicological research (CitationBaytop, 1999; CitationCalzada et al., 1999, Citation2005). Geranium tuberosum subsp. tuberosum L. (Geraniaceae) grows widely through Turkey (CitationDavis, 1966; CitationGüner, 2000). This plant is eaten as salad or added to pastry in Turkey (CitationErtuğ, 2000; CitationKargıoğlu, 2010). From the ethyl acetate (EtOAc) extract of G. tuberosum subsp. tuberosum a phenolic compound (methyl gallate) and several flavonoids as quercetin, quercetin-3-O-β-glucopyranoside, quercetin-3-O-β-galactopyranoside, quercetin-3-O-(2″-O-galloyl)-β-glucopyranoside, quercetin-3-O-(2″-O-galloyl)-β-galactopyranoside, quercetin-3-O-(6″-O-galloyl)-β-glucopyranoside, quercetin-3-O-(6″-O-galloyl)-β-galactopyranoside, quercetin-3-O-α-arabinofuranoside, and quercetin-3-O-α-rhamnopyranosyl-(1→6)-β-glucopyranoside have been isolated previously (CitationŞöhretoğlu et al., 2009).
In this study, effects on glutathione (GSH) level, endogenous antioxidant enzyme (superoxide dismutase, SOD and catalase, CAT) activities as well as antihemolytic effects of different extracts of G. tuberosum subsp. tuberosum have been examined in addition to total polyphenolic content of the extracts.
Materials and methods
Chemicals
Folin–Ciocalteu’s reagent, pyrogallol, trizma TM base, and sodium carbonate were obtained from Sigma. Sodium azide, potassium monohydrogen phosphate, potassium dihydrogen phosphate, hydrogen peroxide (H2O2) solution, and sodium dihydrogen phosphate were purchased from Merck.
The N-(1-pyrenyl) maleimide, GSH, 1,1,3,3-tetraethoxypropane, aminoguanidine hydrochloride were purchased from Sigma. High performance liquid chromatography (HPLC)-grade acetonitrile, methanol (MeOH) and butanol were purchased from Merck and HPLC-grade acetic acid and 85% phosphoric acid were purchased from Riedel.
The HPLC system was Agilent 1200 Series. The system consists of a G 1311A pump, a G 1313A autoinjector, and a G 1321A spectrofluorophotometer. The 100 mm × 4.6 mm column is packed with 3 µm particle size C18 materials. Thiols were resolved by isocratic elution using mobile phase consisting of 60% acetonitrile, 40% nanopure water, 1 mL/L acetic acid, and 1 mL/L O-phosphoric acid. The pH of this solvent system was approximately 2.5.
Plant material
The aerial parts of G. tuberosum subsp. tuberosum were collected from Tuluntaş, Ankara, in May 2009. The plant was identified by Serap Arabacı (Hacettepe University, Faculty of Pharmacy, Department of Pharmaceutical Botany, Ankara, Turkey). Voucher specimens (HUEF 09174) have been deposited at the Herbarium of the Faculty of Pharmacy, Hacettepe University.
Preparation of herbal extracts
Dried and powdered aerial parts of G. tuberosum subsp. tuberosum (20 g) was extracted with 80% MeOH (4 × 50 mL) at room temperature and combined MeOH extracts were concentrated under reduced pressure. The resultant extract (3.4 g, yield 17%) was then dissolved in H2O and water-soluble portion was partitioned against petroleum ether (40–60°C) (4 × 15 mL) (0.68 g), EtOAc (6 × 15 mL) (0.62 g) and n-butanol (n-BuOH) (4 × 15 mL) (0.77 g), respectively.
Blood sampling
Blood samples were collected from healthy volunteers in heparinized polypropylene tubes. After immediate centrifugation (1000g, 10 min, +4°C), plasma and buffy coat phases were removed and erythrocytes were washed four times with phosphate buffer solution (PBS).
Reduced GSH levels
GSH determinations were performed using the HPLC methodology developed by CitationWinters et al. (1995).
SOD activity
SOD activity was measured, by the method of CitationMarklund and Marklund (1974). Blood samples were collected from healthy volunteers. Each erythrocyte hemolysate was further diluted 1:5 with 50 mM Tris–HCl buffer (pH = 8.2; containing 1.2 mM EDTA). Diluted hemolysate (20 μL) was mixed with 2,900 μL of buffer solution in a quartz cuvette. Subsequently, 100 μL of pyrogallol solution (6 mM, in 10 mM HCl) was added to the mixture, mixed for 20 s, and decrease in absorbance was followed at 420 nm for 2 min. Nonenzymatic reaction rate as blank was determined by substituting buffer solution for the sample and this reaction rate was used for the activity measurements.
CAT activity
CAT activity was measured by monitoring the decomposition of H2O2 as previously described (CitationAebi, 1984).
CAT enzyme activity in erythrocyte lysate was measured after dilution of the erytrocyte hemolysates 1:500 with 50 mM phosphate buffer pH 7.00, 10 mM H2O2 and erythrocyte lysate. The reduction rate of H2O2 was followed at 240 nm for 30 s at room temperature.
Protective effect against H2O2-induced hemolysis in erythrocytes
Hemolysis assay was carried out according to literature (CitationŞöhretoğlu et al., 2012). Briefly, pretreatment stage was carried out by adding different concentration of extracts to 200 μL of erythrocyte suspension and incubating at 37°C for 1 h. A positive control for this experiment was prepared by pretreating the erythrocyte suspension with 1 mL of 10 mg/mL of vitamin C dissolved in PBS. The nonpretreated erythrocyte suspension was used as the negative control. The volume of all pretreated and nonpretreated erythrocyte suspensions were adjusted to 2 mL by adding PBS. Oxidative stress was then induced by adding 1 mL of 10 mM H2O2 and incubated at 37°C for 150 min. After incubation, the released hemoglobins into the supernatant of the mixtures were measured using a spectrophotometer at 540 nm and were represented on the basis of the maximum absorbance (100%) in the aliquots of H2O2-induced hemolysis.
Estimation of total phenol content
The total phenolic content was determined using Folin–Ciocalteu reagent. Briefly, 10 µL sample or standard (10–100 µM catechin) plus 150 µL diluted Folin–Ciocalteu reagent (1:4 reagent:water) was placed in each well of a 96-well plate, and incubated at room temperature for 3 min. Following addition of 50 µL sodium carbonate (2:3 saturated sodium carbonate:water) and a further incubation of 2 h at room temperature, absorbance was read at 725 nm. Results are expressed as µmol catechin equivalent (Cat E) per gram. All tests were conducted in triplicate (CitationDomínguez et al., 2005).
Protein determination
Protein was determined by the method of CitationLowry et al. (1951) using bovine serum albumin (BSA) as the standard.
Statistical methods
The results obtained in vitro were statistically processed using Microsoft Excel program. Student’s t-test was applied and p < 0.05 was accepted for statistical significance.
Results and discussion
Oxidative stress is known to directly induce cell damage by modifying target molecules, including proteins, lipids, and DNA. Antioxidants play an important role in inhibiting and scavenging free radicals, thus providing protection to humans against infections and degenerative diseases (CitationLahouel et al., 2006). The mechanisms by which the erythrocyte defends itself against oxidative damage are very efficient and are located in the cytosol and double membrane domains (CitationShiva Shankar Reddy et al., 2007; CitationTulipani et al., 2011). Thereby, erythrocyte is a good model for the oxidative stress studies. For this reason, we used the erythrocytes to evaluate antioxidant potential and antihemolytic effects of Geranium extracts in our study.
H2O2 is not a free radical but is nonetheless highly important because of its ability to penetrate biological membranes. Furthermore, the mechanism increasing the toxic potency of H2O2 is a modified Haber–Weiss reaction that involves the direct reduction of H2O2 by superoxide radicals with the formation of superoxide radicals and hydroxyl radicals. H2O2 generates hydroxyl radicals which is one of the toxicity mechanisms of H2O2 (CitationHalliwell & Gutteridge, 1989; CitationShiva Shankar Reddy et al., 2007).
Cells contain several antioxidant defense mechanisms to prevent oxidative radical damage. These mechanisms include enzymatic and nonenzymatic defenses. GSH is an important antioxidant tripeptide as a part of nonenzymatic defense and an extremely important cell protectant. It is present in virtually all mammalian tissues, provides reducing capacity for several reactions and plays an important role in detoxification of H2O2, other peroxides, and free radicals. GSH in fact is both a nucleophile and a reductant, and can therefore react with electrophilic or oxidizing species before the latter interact with more critical cellular constituents such as nucleic acids and proteins (CitationHalliwell & Gutteridge, 1989; CitationPowers & Hamilton, 1999). Therefore, decreased GSH levels may reflect a depletion of nonenzymatic antioxidant reserves.
The results from the experiment the effect of the extracts on H2O2-induced diminution of GSH levels are shown in . As it is shown in the figure, none of the extracts prevented H2O2-induced decrease of GSH level at 50 µg/mL. On the contrary, all the extracts have the significant prevention on this diminution at 100 µg/mL. BuOH, EtOAc and water extracts showed the same preventive effect at 200 µg/mL concentration whereas MeOH extract did not. These results show all the extracts have the dose dependent antioxidant activity.
Figure 1. GSH levels in erytrocyte. H2O2, hydrogen peroxide; blank, without extracts; AA, ascorbic acid; BuOH, butanol; EtOAc, ethyl acetate; MeOH, methanol. *p < 0.05, significantly different from blank; δp < 0.05, significantly different from ascorbic acid; &p < 0.05, significantly different from H2O2. Results are expressed as mean ±SD values of three observations.
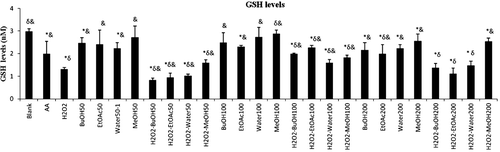
CAT and SOD are important parts of antioxidant defense in the cells (CitationHalliwell & Gutteridge, 1989).
CAT is one of the major antioxidant enzymes in human erythrocytes. In vivo degradation of H2O2 at physiological concentrations is catalyzed by glutathione peroxidase, while higher concentrations are detoxified by CAT. In this respect, any compound increasing the activity of CAT might be considered “beneficial,” since it will contribute decomposing H2O2 and prevent the formation of most dangerous hydroxyl radical (CitationHalliwell & Gutteridge, 1989; CitationHalliwell, 1994). represents the effects of the extracts on the activity of CAT.
Figure 2. Concentration response (A: percentage of inhibition of CAT activity; B: percentage inhibition of SOD activity) of the G. tuberosum extracts, ascorbic acid and blank. H2O2, hydrogen peroxide; blank, without extracts; AA, ascorbic acid; BuOH, butanol; EtOAc, ethyl acetate; MeOH, methanol. *p < 0.05, significantly different from blank; δp < 0.05, significantly different from ascorbic acid. Results are expressed as mean ±SD values of three observations.
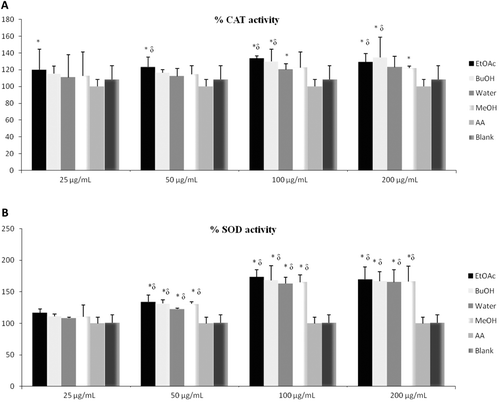
SOD is another major antioxidant enzyme in human erythrocytes. SOD catalyzes dismutation of superoxide anion radical to H2O2. Two enzymes degrade H2O2: glutathione peroxidase and CAT. Thus, SOD functions at the first step to detoxify superoxide anion radical and the other two enzymes complete the process (CitationHalliwell & Gutteridge, 1989). represents the effects of the extracts on the activity of SOD.
These findings confirm that different samples of extracts have dose-dependent influence on these two enzymes. Therefore, all the extracts increased SOD and CAT activities depending on antioxidant activity.
In the present study, the maximum erythrocyte hemolysis (100%) was accepted using 1 M H2O2 and other hemolytic values from free radical damage were presented as percentage based on the former. The results of the erythrocytes hemolysis assay are shown in .
Figure 3. Cytotoxicity of plant extracts by showing hemolytic capacity. A: 50 µg/mL extract; B: 100 µg/mL extract; C: 200 µg/mL extract. H2O2, hydrogen peroxide; blank, without extracts; AA, ascorbic acid; BuOH, butanol; EtOAc, ethyl acetate; MeOH, methanol. *p < 0.05, significantly different from blank; δp < 0.05, significantly different from ascorbic acid; &p < 0.05, significantly different from H2O2. Results are expressed as mean ±SD values of three observations.
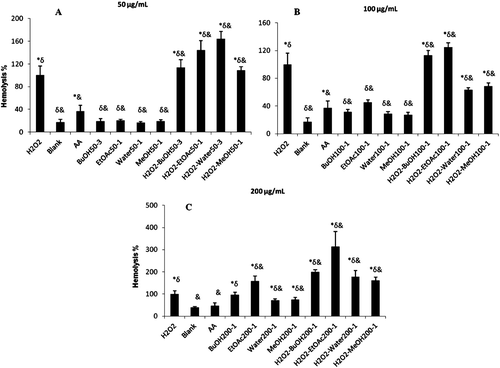
The assay was performed at three concentrations and results are presented as mean percent induction to hemolysis. Interestingly, according to the results, extracts don’t have the antihemolytic effects at 50 and 200 µg/mL. However, it is shown that water and MeOH extracts have significant antihemolytic activity at 100 µg/mL.
According to the studies, polyphenols have the antioxidant effects but they can also exert pro-oxidant activities under certain experimental conditions (CitationSakihama et al., 2002; CitationLahouel et al., 2006; CitationHalliwell, 2008).
The total phenolic content of the extracts is given in . The EtOAc extract had the highest polyphenol content, followed by BuOH, MeOH and water extracts. In general, the EtOAc fraction of G. tuberosum subsp. tuberosum extracts showed higher phenolic content, better antioxidant activity.
Conclusion
Geranium species are well known for their polyphenolic contents and traditional usage worldwide (CitationCalzada et al., 1999, Citation2005). Natural antioxidants are useful in the food industry as preservatives to increase the life of food products preventing the loss of their sensory and nutritional quality.
In this study, we investigated the food plant, G. tuberosum subsp. tuberosum extracts for antioxidant activities and antihemolytic activity in order to find promising herbal extracts and compounds for drug discovery. Concerning our results above, all extracts enhanced the GSH levels, activity of SOD and CAT, although they did not increase the resistance of erythrocytes to H2O2-induced cytotoxicity, except MeOH extracts at 100 µg/mL. Our enzymatic assay results provide important evidence that G. tuberosum subsp. tuberosum is a potent source of protective agent against the oxidative process. On the other hand, it has been reported that plant polyphenols are naturally occurring antioxidants but they also exhibit pro-oxidant properties under certain conditions. For instance, the pro-oxidant action of plant polyphenols could contribute to their anticancer properties (CitationSakihama et al., 2002; CitationLahouel et al., 2006). High dietary intake of polyphenols is associated with low risk of cardiovascular, neurodegenerative disease and cancer (CitationOvaskainen et al., 2008).
In summary, this study has indicated that extracts with different polarities (EtOAc, BuOH, water and MeOH) of G. tuberosum subsp. tuberosum exhibit different biological activities, which could be attributed to mainly on the presence of phenolic compounds and flavonoids indicated in the literature (CitationŞöhretoğlu et al., 2009). High correlation between the amount of phenolic content with the cytotoxicity and antioxidant activities was revealed. In conclusion, G. tuberosum subsp. tuberosum may be potent source of protective chemicals against the oxidative process. These findings make the title plant very interesting for the search for new therapeutic agents. Our results suggest that further investigations are necessary to determine the medicinal properties of the plant in vivo and in vitro.
Declaration of interest
The authors declare no conflicts of interest.
References
- Aebi H. (1984). Catalase in vitro. Meth Enzymol, 105, 121–126.
- Baytop T. (1999). Therapy with Medicinal Plants in Turkey (Past and Present). Istanbul, Turkey: Nobel Medical Publishing.
- Calzada F, Cerda-García-Rojas CM, Meckes M, Cedillo-Rivera R, Bye R, Mata R. (1999). Geranins A and B, new antiprotozoal A-type proanthocyanidins from Geranium niveum. J Nat Prod, 62, 705–709.
- Calzada F, Cervantes-Martínez JA, Yépez-Mulia L. (2005). In vitro antiprotozoal activity from the roots of Geranium mexicanum and its constituents on Entamoeba histolytica and Giardia lamblia. J Ethnopharmacol, 98, 191–193.
- Davis PH. (1966). Geranium L. In: Davis PH, ed. Flora of Turkey and East Aegean Islands. Vol 2. Edinburgh: Edinburgh University Press, 441–474.
- Domínguez M, Nieto A, Marin JC, Keck AS, Jeffery E, Céspedes CL. (2005). Antioxidant activities of extracts from Barkleyanthus salicifolius (Asteraceae) and Penstemon gentianoides (Scrophulariaceae). J Agric Food Chem, 53, 5889–5895.
- Ertuğ F. (2000). An ethnobotanical study in central Anatolia (Turkey). Econ Bot, 54, 155–182.
- Güner A. (2000). Geranium L. In: Güner A, Özhatay N, Ekim T, Başer KHC, eds. Flora of Turkey and East Aegean Islands. Suppl. II. Edinburgh: Edinburgh University Press, 104–105.
- Halliwell B, Gutteridge JMC. (1989). Free Radicals in Biology and Medicine. Oxford: Clarendion Press.
- Halliwell B. (1994). Free radicals and antioxidants: A personal view. Nutr Rev, 52, 253–265.
- Halliwell B. (2008). Are polyphenols antioxidants or pro-oxidants? What do we learn from cell culture and in vivo studies? Arch Biochem Biophys, 476, 107–112.
- Kargıoğlu M, Cenkci S, Serteser A, Konuk M, Vural, G. (2010). Traditional uses of wild plants in the middle Aegean Region of Turkey. Human Ecol, 38, 429–450.
- Lahouel M, Amedah S, Zellagui A, Touil A, Rhouati S, Benyache F, Leghouchi E, Bousseboua H. (2006). The interaction of new plant flavonoids with rat liver mitochondria: Relation between the anti- and pro-oxydant effect and flavonoids concentration. Therapie, 61, 347–355.
- Lowry OH, Rosebrough NJ, Farr AL, Randall RJ. (1951). Protein measurement with the Folin phenol reagent. J Biol Chem, 193, 265–275.
- Lu Y, Foo LY. (2000). Flavonoid and phenolic glycosides from Salvia officinalis. Phytochemistry, 55, 263–267.
- Marklund S, Marklund G. (1974). Involvement of the superoxide anion radical in the autoxidation of pyrogallol and a convenient assay for superoxide dismutase. Eur J Biochem, 47, 469–474.
- Ovaskainen ML, Törrönen R, Koponen JM, Sinkko H, Hellström J, Reinivuo H, Mattila P. (2008). Dietary intake and major food sources of polyphenols in Finnish adults. J Nutr, 138, 562–566.
- Powers SK, Hamilton K. (1999). Antioxidants and exercise. Clin Sports Med, 18, 525–536.
- Rice-Evans C, Miller N. (1997). Measurement of the antioxidant status of dietary constituents, low density lipoproteins and plasma. Prostaglandins Leukot Essent Fatty Acids, 57, 499–505.
- Robards K, Prenzler PD, Tucker G, Swatsitang P, Glover W. (1999). Phenolic compounds and their role in oxidative processes in fruits. Food Chem, 66, 401–436.
- Sakihama Y, Cohen MF, Grace SC, Yamasaki H. (2002). Plant phenolic antioxidant and prooxidant activities: Phenolics-induced oxidative damage mediated by metals in plants. Toxicology, 177, 67–80.
- Shiva Shankar Reddy CS, Subramanyam MV, Vani R, Asha Devi S. (2007). In vitro models of oxidative stress in rat erythrocytes: Effect of antioxidant supplements. Toxicol In Vitro, 21, 1355–1364.
- Şöhretoğlu D, Sakar MK, Atasayar Sabuncuoğlu S, Özgüneş H, Sterner O. (2009). Antioxidant galloylated flavonoids from Geranium tuberosum L. subsp. tuberosum. Turk J Chem, 33, 685–692.
- Şöhretoğlu D, Sabuncuoglu S, Harput US. (2012). Evaluation of antioxidative, protective effect against H2O2 induced cytotoxicity, and cytotoxic activities of three different Quercus species. Food Chem Toxicol, 50, 141–146.
- Tulipani S, Alvarez-Suarez JM, Busco F, Bompadre S, Quiles JL, Mezzetti B, Battino M. (2011). Strawberry consumption improves plasma antioxidant status and erythrocyte resistance to oxidative haemolysis in humans. Food Chem, 128, 180–186.
- Winters RA, Zukowski J, Ercal N, Matthews RH, Spitz DR. (1995). Analysis of glutathione, glutathione disulfide, cysteine, homocysteine, and other biological thiols by high-performance liquid chromatography following derivatization by n-(1-pyrenyl) maleimide. Anal Biochem, 227, 14–21.