Abstract
Context: Cucurbitacins are a group of triterpenoids that have a cucurbitane skeleton with a wide range of biological activities.
Objectives: This study evaluated the anticancer properties of one cucurbitacin isolated from Cayaponia racemosa Cong. (Cucurbitaceae), 2β,3β,16α,20(R),25-pentahydroxy-22-oxocucurbita-5-en (1), with in vitro and in vivo models.
Materials and methods: In vitro cytotoxic activity was determined with human leukemia (HL60) and normal blood cells (PBMC). Sarcoma 180 was used as in vivo model.
Results: The cucurbitacin (1) reduced the number of viable cells; however, there was no changed in the number of non-viable cells at 5 µg/mL. Selectivity towards cancer cells was suggested by the absence of activity on normal proliferating lymphocytes at the concentrations tested (IC50 >25 µg/ml). Morphological analysis of compound 1-treated cells showed typical apoptotic features, such as intense deposition of granules in the cytoplasm (eosinophilia), DNA fragmentation and irregularities in the plasma membrane. In addition, the cells treated with compound 1 presented intense vacuolization and disruption of the plasma membrane. Acridine orange/Ethidium bromide staining confirmed these findings, revealing an increased number of apoptotic cells. In the Sarcoma 180 tumor model, compound 1 showed 52 and 62% of antitumor activity, either alone (25 mg/kg/day) or in association with the chemotherapeutic agent 5-FU (10 + 10 mg/kg/day), respectively. Moreover, either alone or associated with 5-FU, treatment with compound 1 caused an increase in spleen weight and morphological alterations related to immunostimulatory properties.
Conclusion: These data indicate that these naturally occurring compounds have anticancer potential.
Keywords::
Introduction
Cucurbitacins are a special group of triterpenoids that have a cucurbitane skeleton, and a wide range of biological activities is associated with their chemical structural complexity (CitationChen et al., 2005). Since their isolation and structural elucidation in the 1960s, these compounds have been of great interest within the medicinal chemistry and drug discovery communities, particularly from an anticancer drug development perspective (CitationChen et al., 2005). Some reports attributed in vitro and in vivo anticancer activities to the cucurbitacins (CitationFang et al., 1984; CitationDuncan et al., 1996; CitationChan et al., 2010a; CitationSun et al., 2010). Jayaprakasam and co-workers demonstrated the cytotoxic properties of cucurbitacins B, D, E and I, isolated from the fruits of Cucurbita andreana, against colon, breast, lung and central nervous system cancer cell lines (CitationJayaprakasam et al., 2003). Among this class of compounds, cucurbitacin B is the most studied, and while several studies demonstrated its efficacy in different models of cancer, including in vivo tumor xenografts (CitationLiu et al., 2008; CitationWakimoto et al., 2008; CitationZhang et al., 2009; Chan et al., 2010), there is disagreement regarding the mechanisms underlying its anticancer activity. Suppression of the oncogene STAT 3 (signal transducer and activator of transcription 3) appears to be related to tumor inhibition (Zhang et al., 2004; CitationChan et al., 2010b), which does not exclude alternative mechanisms (CitationYasuda et al., 2010).
Our previous work with Cayaponia racemosa Cong. (Cucurbitaceae), a climber plant found in northeastern Brazil and popularly known as “guardião,” described the isolation of three cucurbitacins, 2,3,16,20(R),25-pentahydroxy-11,22-dioxocucurbita-5-en, 2,3,16,20(R),25-pentahydroxy-22-oxocucurbita-5-en and 2,3,16,20(R),25-pentahydroxy-22-oxocucurbita-5,23(E)-diene, which induced lethality to brine shrimp, inhibited the development of sea urchin eggs and slowed tumor cell proliferation, highlighting their anticancer potential (CitationDantas et al., 2006). The purpose of this work was to investigate the anticancer activity of 2,3,16,20(R),25-pentahydroxy-22-oxocucurbita-5-en (compound 1) using in vitro and in vivo models.
Materials and methods
Plant material
The plant material was collected in Palmácia, Ceará, Brazil and it was identified by Dr. Edson Paula Nunes. A voucher specimen (30532) was deposited at the Herbário Prisco Bezerra, Federal University of Ceará, Brazil. The air-dried, powdered fruits (0.91 kg) of C. racemosa were extracted exhaustively with EtOH (3 L) at room temperature. After removal of the solvent under reduced pressure, the dark green residue (27.8 g) was fractionated by silica gel (Kieseegel 60 H) column chromatography using hexane, CHCl3, EtOAc and MeOH as eluents. The EtOAc fraction (8.34 g) contained a mixture of cucurbitacins. A portion (700.0 mg) of this fraction was subjected to reverse-phase hplc on a Hibar RP-18 column (250 × 4 mm) and eluted with MeOH/H2O (6:4) to yield 2β,3β,16α,20(R),25-pentahydroxy-22-oxocucurbita-5-en (1) (325.0 mg), −687.6° (c 0.43, C5H5N), m.p. 141–144°C. Structural elucidation of 1 () was based mainly on spectroscopic analyses using infrared (IR) and 1H and 13C NMR, including special pulse sequences (one- and two-dimensional NMR, such as DEPT 135°, 1H 1H COSY, 1H 13C HMQC, 1H 13C HMBC and 1H 1H NOESY), and the spectrographs were compared with published data (CitationStuppner et al., 1981; CitationAbd El-Fattah, 1994).
Figure 1. A – Chemical structure of 2β,3β,16α,20(R),25-pentahydroxy-22-oxocucurbita-5-en (1) isolated from Cayaponia racemosa. B – Activity of compound 1 and at 2.5 and 5.0 µg/mL on HL60 cells assessed by Trypan blue exclusion. C – Effect of cucurbitacin on the pattern of HL60 cell death, determined by acridine orange and ethidium bromide staining (AO/BE). Negative controls (C) were treated with solvent (DMSO); positive controls (+) were treated with doxorubicin (0.3 µg/mL). The cells were treated for 24 h. *p < 0.05 compared with controls by ANOVA followed by Student Newman–Keuls test.
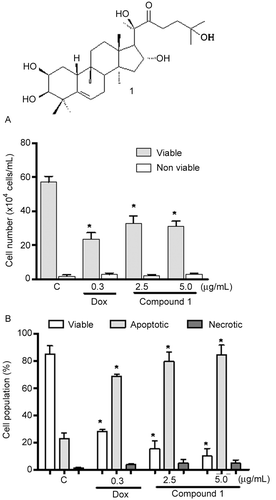
Antiproliferative activity with human leukemia (HL-60) and peripheral blood mononuclear cells (PBMC)
To investigate the selectivity of compound 1 for normal proliferating cells, the Alamar blue™ assay was performed with human peripheral blood mononuclear cells (PBMC) after 72 h of drug exposure. All studies were performed in accordance with Brazilian research guidelines (Law 196/96, National Council of Health). Heparinized blood (from healthy, non-smoker donors who had not taken any drug for at least 15 days prior to sampling, aged between 18 and 30 year olds) was collected, and PBMC were isolated by the standard method of density-gradient centrifugation over Ficoll-Histopaque. The cells were removed, washed with phosphate buffer saline (PBS) and resuspended in RPMI 1640 medium supplemented with 20% of fetal bovine serum, 100 U/mL penicillin and 100 µg/mL of streptomycin to a final concentration of 3 × 105 cells/mL. Phytohemagglutinin (4%) was added at the beginning of culture, according to CitationFerreira et al. (2010) with some modifications. After 24 h, the compounds (0.39–25 µg/mL) dissolved in DMSO were added to each well and incubated for 72 h. Doxorubicin (0.009–5 µg/mL) was used as positive control. Control groups received the same amount of DMSO. Twenty-four hours before the end of the incubation, 10 µL of Alamar blue stock solution (Resazurin, Sigma Aldrich Co., 0.312 mg/mL) was added to each well. The absorbance was measured using a multiplate reader (DTX 880 Multimode Detector, Beckman Coulter®), and the drug effect was quantified as the percentage of the control absorbances at 570 and 595 nm. HL60 cell line (promyelocytic leukemia) was obtained from the National Cancer Institute (Bethesda, MD) and was maintained in RPMI 1640 medium supplemented with 10% fetal bovine serum, 2 mM glutamine, 100 U/mL penincillin and 100 µg/mL streptomycin, at 37°C with 5% CO2. The experiments were performed to investigate the mechanism underlying the cytotoxic effect of a 24 h exposure to cucurbitacin 1 on HL-60. The compound, dissolved in sterilized DMSO, was added to HL-60 cells (3 × 105 cells/mL) to final concentrations of 2.5 and 5.0 µg/mL. Doxorubicin (0.3 µg/mL) was used as a positive control. Cell viability was determined by the Trypan blue dye exclusion test. After incubation of HL-60 cells with cucurbitacin, aliquots were removed from the cultures and stained with Trypan blue. The cells were then scored for dye exclusion in a Neubauer chamber.
Morphological analysis with fluorescence microscopy
Acridine orange/ethidium bromide (AO/EB) staining of HL-60 cells was performed to evaluate the cell death pattern induced by cucurbitacin treatment. After the incubation, the cells were pelleted, and each sample was mixed with 1 µL of aqueous AO/EB solution (100 µg/mL of AO in PBS; 100 µg/mL EB in PBS). Three hundred cells were counted per sample using a fluorescence microscope (Olympus, Tokyo, Japan) and scored as viable, apoptotic or necrotic (CitationMilitão et al., 2006).
Analysis of morphological changes by hematoxylin-eosin staining
Untreated or cucurbitacin-treated HL-60 cells were examined for morphological changes by light microscopy (Metrimpex Hungary/PZO-Labimex Modelo Studar lab®). To evaluate morphology, cells were harvested, transferred to cytospin slides, fixed with methanol for 1 min and stained with hematoxylin-eosin (H/E) (CitationCury-Boaventura et al., 2004).
In vivo antitumor evaluation
Adult Swiss mice were obtained from the animal facilities of Universidade Federal do Ceará. They were kept in well-ventilated cages (ALESCO, São Paulo) under standard conditions of light (12 h with alternative day and night cycles) and temperature (25 ± 1°C) and were housed with access to commercial rodent stock diet (Purina, São Paulo, Brazil) and water ad libitum. The investigational protocol was approved by the local Ethical Committee on Animal Research at Universidade Federal do Ceará (Process No. 102/2007) and is in accordance with the international standard on the care and use of experimental animals (EEC Directive of 1986, 86/609/EEC). Sixty healthy male mice weighing 23–26 g were subcutaneously implanted with 9-day-old Sarcoma 180 ascites tumor cells (2 × 106 cells/0.5 mL) into the left hind groin. The next day, the mice were randomly divided into six groups (n = 10 per group), and compound 1 dissolved in DMSO 4% was intraperitoneally administered for 7 days at 10 and 25 mg/kg/day doses with or without 5-fluorouracil (5-FU + compound 1, 10 + 10 mg/kg/day, respectively). Negative and positive controls received DMSO 4% and 5-FU (10 mg/kg/day), respectively. On day 8, mice were sacrificed by cervical dislocation, and their organs (kidneys, spleens and livers) and tumors were dissected out, grossly examined for size, color changes and hemorrhage, weighed and preserved in a 10% formaldehyde solution. The inhibition of tumor growth (%) was calculated using the following formula: percent inhibition (%) = [(A–B)/A] × 100, where A is the average tumor weight of the negative control, and B is the average tumor weight of the treated group. To examine morphological changes by light microscopy (Olympus, Tokyo, Japan), small pieces of organs and tumors were processed and embedded in paraffin, and 3–5 µm sections were prepared and stained with hematoxylin-eosin.
Statistical analysis
For cytotoxicity assays, the IC50 values and their 95% confidence intervals were obtained by nonlinear regression using the GraphPad Prism program (GraphPad Software, San Diego, CA). To determine differences, data were compared by one-way analysis of variance (ANOVA) followed by Newman–Keuls test (p < 0.01).
Results
Antiproliferative activity on HL-60 leukemia cell and peripheral blood mononuclear cells (PBMC)
Trypan blue exclusion analysis confirmed the anti-proliferative activity of cucurbitacin 1 () on HL60. The compound reduced the number of viable cells at both tested concentrations. After a 24-h incubation at 2.5 µg/mL, compound 1 inhibited growth by 40%, without altering the number of non-viable cells (). Only doxorubicin, the positive control, caused cytotoxicity to peripheral blood mononuclear cells with an IC50 of 0.02 µg/mL (0.03 µM), suggesting that the cucurbitacins 1 isolated from C. racemosa was selective for cancer cells, with an IC50 > 25 µg/mL.
Analysis of morphological changes
To further determine whether the growth-inhibitory effect of cucurbitacins was related to the induction of apoptosis and/or necrosis, treated cells were analyzed using AO/EB staining, and the numbers of viable, apoptotic and necrotic cells were determined by fluorescence microscopy. After 24 h of treatment with tested compound, a decreased number of viable cells and an increased number of apoptotic cells were observed in a dose-dependent manner (). The number of viable cells reduced from 85% (control) to 10% after treatment with 5 µg/mL of compound 1. The number of apoptotic cells increased from 20% (control) to 85 and 95% after treatment with 2.5 and 5 µg/mL of compound 1, respectively. Regarding necrosis, no increase was observed after treatment with 5 µg/mL of cucurbitacin ().
Analysis of HL-60 by hematoxilin/eosin staining revealed several drug-induced morphological changes. After 24 h in culture, control cells exhibited a typical non-adherent and vacuolated round morphology. HL60 cells treated with compound 1 showed DNA fragmentation, membrane blebs and eosinophilic granules in the cytoplasm. Doxorubicin (0.3 µg/mL) also reduced cell volume, in addition to causing fragmentation of the DNA and blebbing of the plasma membrane ().
In vivo antitumor evaluation
The effects of compound 1 on tumor growth are presented in . A significant reduction in tumor weight (p < 0.01) was found at 25 mg/kg/day for compound 1- and 5-FU + compound 1-treated animals (1.01 ± 0.1 and 0.8 ± 0.1 g, respectively) in comparison to negative controls (1.7 ± 0.1 g), representing 52 and 62% tumor growth inhibition, respectively. In contrast, at 10 mg/kg/day, neither 5-FU nor compound 1 was able to inhibit tumor growth under identical experimental conditions (p > 0.05).
Figure 3. Tumor mass of mice transplanted with Sarcoma 180 cells after 7 days of treatment by intraperitoneal route with compound 1 (2β,3β,16α,20(R),25-pentahydroxy-22-oxocucurbita-5-en) isolated from Cayaponia racemosa and in association with 5-fluorouracil (5-FU, positive control). Negative control (C) received only DMSO 4%. Data are expressed as mean ± SEM (n = 10 animals/group). Within the square is demonstrated the tumor inhibition capacity expressed as perceptual in comparison with the negative control. *p < 0.01 compared to control by ANOVA followed by Newman–Keuls test.
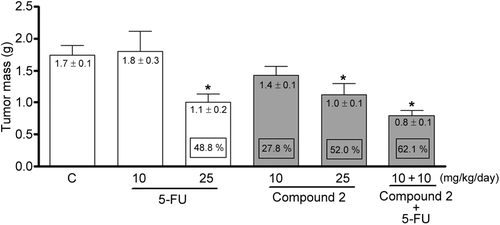
Examination of tumor morphology from the control group revealed large, polygonal cells with pleomorphic shapes, hyperchromatic nuclei, acidophilic macronucleoli, atypical mitosis and muscle invasion, typical findings in a neoplasm (). Similar results were observed after treatment with 5-FU () and compound 1 () at 10 mg/kg/day. Meanwhile, tumors excised from mice treated with 5-FU at 25 mg/kg/day (), compound 1 at 25 mg/kg/day () and 5-FU + compound 1 at 10 + 10 mg/kg/day () exhibited extensive areas of coagulative necrosis interspersed with pleormorphic cells. Compound 1, either alone or in association with 5-FU, reduced tumor growth to a similar extent as did the positive control, 5-FU, confirming the in vivo antiproliferative activity of the cucurbitacin.
Figure 4. Histopathology of tumors excised from Sarcoma 180 transplanted mice after 7 days of intraperitoneal treatment with compound 1 (2β,3β,16α,20(R),25-pentahydroxy-22-oxocucurbita-5-en) isolated from Cayaponia racemosa. A – Negative control (DMSO 4%), B and C – 5-Fluorouracil 10 and 25 mg/kg/day, D and E – Compound 1, 10 and 25 mg/kg/day, F – 5-Fluorouracil + Compound 1 (10 + 10 mg/kg/day), respectively. Magnification, 400×.
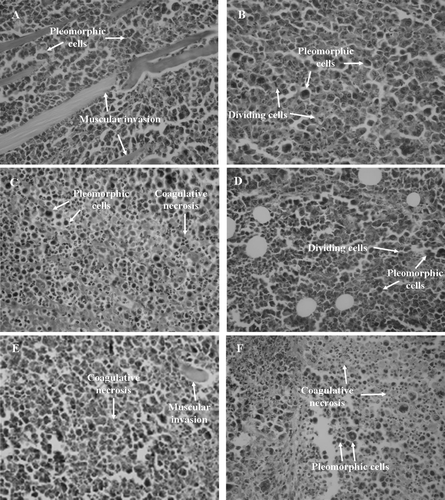
In mice, intraperitoneal treatment with compound 1 did not interfere with final body weight and relative liver and kidney weights of the experimental groups () when compared to the negative control group (p > 0.05). Moreover, neither mortality nor morbidity was detected during the experiment. 5-FU (25 mg/kg) and the co-treatment with 5-FU caused a reduction in body weight (p < 0.01), and all groups administered compound 1 displayed an increase in relative spleen weight (0.70 ± 0.04 and 0.84 ± 0.03 g, 10 and 25 mg/kg/day, respectively), including the group administered the associated treatment (0.64 ± 0.03 g) (p < 0.01) ().
Table 1. Body effects of compound 1 (2β,3β,16α,20(R),25-pentahydroxy-22-oxocucurbita-5-en) isolated from Cayaponia racemosa on mice transplanted with Sarcoma 180 cells.
Figure 5. Histopathology of spleens excised from Sarcoma 180 transplanted mice after 7 days of intraperitoneal treatment with compound 1 (2β,3β,16α,20(R),25-pentahydroxy-22-oxocucurbita-5-en) isolated from Cayaponia racemosa. A – Negative control (DMSO 4%), B and C – 5-Fluorouracil 10 and 25 mg/kg/day, D and E – Compound 1, 10 and 25 mg/kg/day, F – 5-Fluorouracil + Compound 1 (10 + 10 mg/kg/day), respectively. Magnification, 400×.
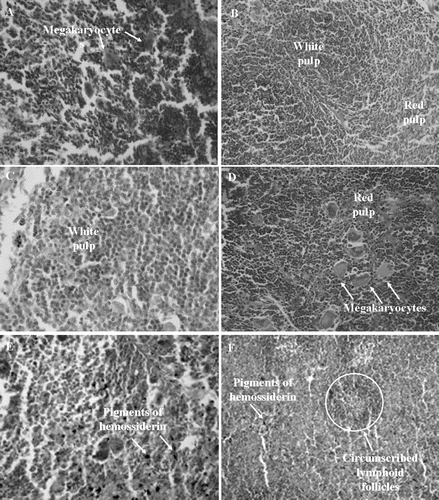
Hepatic analysis of compound 1-treated mice (10 and 25 mg/kg/day) revealed histological findings similar to that of the negative control group. Comparable alterations were found in the liver of the 5-FU co-treated group, but it also showed sinusoidal hemorrhage and focal infiltration of inflammatory cells, the latter more evident in the groups treated with 5-FU (10 mg/kg/day), which presented focal microvesicular steatosis and enucleated hepatocytes (data not shown). Regarding the kidneys, glomerular and tubular hemorrhage and hyaline cylinders with preservation of the basic outline of glomerular and tubular architecture was observed in all groups. Focal hydropic changes of the tubular epithelium in those mice that received compound 1 at 25 mg/kg/day were also detected.
There was considerable hyperplasia of the splenic white pulp [5-FU + compound 1 (10 + 10 mg/kg/day), ] and megakaryocytes (10 mg/kg/day, ) in all groups treated with compound 1. Pigments of hemosiderin were observed more frequently after treatment with 25 mg/kg/day of compound 1 () and in the 5-FU co-treated group (). Meanwhile, negative controls demonstrated no alterations, and 5-FU groups exhibited circumscribed lymphoid follicles, which were more pronounced in the 5-FU co-treated group ().
Discussion
The search for antineoplastic compounds has demonstrated the great pharmacological relevance of plant secondary metabolites (CitationCragg & Newman, 2005). Curcubitacins possess remarkable pharmacological effects, such as inhibition of cell adhesion (CitationMusza et al., 1994) and T-lymphocyte proliferation (CitationSmit et al., 2000) and anti-inflammatory (CitationJayaprakasam et al., 2003), immunomodulatory (CitationAttard et al., 2005) and anti-angiogenic activities (CitationDuncan et al., 1996).
Previous work indicated that compound 1 has cytotoxic effects against HL-60 (human leukemia), CEM (human leukemia), HCT-8 (human colon cancer), MCF-7 (human breast cancer) and B-16 (murine melanoma) tumor cell lines. Nevertheless, this compound did not induce hemolysis or alter the development of sea urchin eggs (CitationDantas et al., 2006).
In the first part of this study, we demonstrated that the cucurbitacin 1 was not cytotoxic to PBMCs at the tested concentrations. In terms of chemotherapy side effects, it is very important to verify whether the drug shows a harmful effect against normal dividing cells, such as proliferating lymphocytes. Additional experiments were performed with HL-60 cells to access the mechanisms underlying the cytotoxic activity of this cucurbitacin on tumor cells. The results of our experiments suggest that the treated cells died via the apoptotic pathway, which is characterized by dissipation of the mitochondrial transmembrane potential and peripheral nuclear condensation, followed by DNA fragmentation and membrane blebbing. Apoptosis is regarded as the preferred process for eliminating undesired cells, and induction of that process is an important strategy in cancer therapies (CitationLeistl & Jäättelä, 2001).
The capacity of the cucurbitacins to induce apoptosis in cultured cells was addressed in several studies (CitationShan et al., 2010; CitationYasuda et al., 2010). However, the precise effects and the mechanisms involved in the cellular effects seemed to be greatly affected by the structures of the cucurbitacins and by the cell line studied (CitationShan et al., 2010; CitationYasuda et al., 2010). Cucurbitacin B, as previously mentioned, was extensively studied in different models. Doskotch and colleagues were the first to describe the antiproliferative effects of cucurbitacin B using in vitro models (CitationDoskotch et al., 1969). Recently, CitationChan et al. (2010a) demonstrated the in vitro and in vivo effects of cucurbitacin B against hepatocellular carcinoma. They showed that cucurbitacin B also led to a cyclin D1/cdc2-dependent S-phase arrest followed by apoptosis, with an inhibition of c-Raf activation and unaltered STAT3 phosphorylation. However, the same research group described that the effects of cucurbitacin B on K562 leukemia cells were related to the inhibition of STAT-3 expression (CitationChan et al., 2010b). Another recent work on the effects of cucurbitacin E on pancreatic cells demonstrated an association with inhibition of STAT 3 (CitationSun et al., 2010). The STAT proteins have dual roles as cytoplasmic signaling proteins and nuclear transcription factors. Persistent activation of Stats, particularly STAT3, has been implicated in the pathogenesis of a whole spectrum of malignancies, including T-cell lymphoma (CitationBlaskovich et al., 2003). Previous studies showed that cucurbitacin I decreased the expression of Stat3 in Sz cells (T-cell lymphoma) and ALK+ALCL cells (ALK-positive anaplastic large cell lymphoma). Furthermore, cucurbitacin I inhibited the growth of the aforementioned cells by inducing apoptosis (CitationShi et al., 2006). These data reinforce the anticancer potential of cucurbitacins, while also highlighting the diverse mechanisms that can be triggered by this class of compounds.
Herein, we also reported, for the first time, the antitumor activity of compound 1, obtained from C. racemosa, in mice bearing the Sarcoma 180 tumor, a mouse-derived tumor widely exploited in antitumor research in vivo (CitationSato et al., 2005; CitationMagalhães et al., 2010). Compound 1, either alone or in association, with 5-FU, reduced tumor growth to a similar extent as did the positive control, 5-FU, confirming the in vivo antiproliferative activity of the cucurbitacin. Cucurbitacin B, another molecule isolated from C. racemosa, dose-dependently inhibited hepatocellular carcinoma cell (BEL-7402) tumor growth in vivo at 0.1, 0.5 and 1 mg/kg by mouth (CitationChan et al., 2010a). In association with docetaxel, cucurbitacin B also d antitumor effects in the Hep-2 (laryngeal cancer) xenograft model (CitationLiu et al., 2008). Because such combined biological therapies hold promise for controlling tumor proliferation and drug-resistance, the development of combination chemotherapies is expected to increase the response rate and the frequency of long-term survival (CitationBezerra et al., 2008).
The kidney and liver have been proposed as key organs for the metabolism of environmental toxic substances (CitationScheuer & Lefkowitch, 2000). Furthermore, these organs also possess a pronounced regenerative capacity: even when necrosis is found with conjunctive tissue preservation, there is often complete tissue restoration (CitationKumar et al., 2004). In this context, the activity of compound 1 in vivo was related to moderate effects on the liver and kidney, and all observed morphological alterations in cucurbitacin-treated animals could be considered potentially reversible. Additionally, compound 1 presented effects on the spleen of treated animals, leading to hyperplasia of the splenic white pulp and an increased number of megakaryocytes, which is suggestive of an immunostimulatory effect that may also contribute to its in vivo antitumor properties (CitationImboden, 1988).
Conclusions
In conclusion, the present data highlight the importance of cucurbitacins as an emerging class of potential anticancer lead molecules, exhibiting an antiproliferative effect on leukemia cells and an antitumor effect in vivo that is associated with reversible toxic effects.
Acknowledgments
We are grateful to Silvana França for technical assistance. The English was edited by American Journal Experts (key # ADC5-52E4-E64C-F4E9-24BD).
Declaration of interest
We wish to thank Conselho Nacional de Desenvolvimento Científico e Tecnológico (CNPq), Coordenação de Aperfeiçoamento de Pessoal de Nível Superior (CAPES), Fundação Cearense de Apoio ao Desenvolvimento Científico e Tecnológico (FUNCAP), Financiadora de Estudos e Projetos (FINEP), Banco do Nordeste do Brasil (BNB) and Instituto Claude Bernard (InCb) for financial support in the form of grants and fellowship awards. The authors report no declarations of interest.
References
- Abd El-Fattah H. (1994). Structure revision of cucurbitacin Q1. Phytochemistry, 36, 159–161.
- Attard E, Brincat MP, Cuschieri A. (2005). Immunomodulatory activity of cucurbitacin E isolated from Ecballium elaterium. Fitoterapia, 76, 439–441.
- Bezerra DP, de Castro FO, Alves AP, Pessoa C, de Moraes MO, Silveira ER, Lima MA, Elmiro FJ, de Alencar NM, Mesquita RO, Lima MW, Costa-Lotufo LV. (2008). In vitro and in vivo antitumor effect of 5-FU combined with piplartine and piperine. J Appl Toxicol, 28, 156–163.
- Blaskovich MA, Sun J, Cantor A, Turkson J, Jove R, Sebti SM. (2003). Discovery of JSI-124 (cucurbitacin I), a selective Janus kinase/signal transducer and activator of transcription 3 signaling pathway inhibitor with potent antitumor activity against human and murine cancer cells in mice. Cancer Res, 63, 1270–1279.
- Chan KT, Li K, Liu SL, Chu KH, Toh M, Xie WD. (2010a). Cucurbitacin B inhibits STAT3 and the Raf/MEK/ERK pathway in leukemia cell line K562. Cancer Lett, 289, 46–52.
- Chan KT, Meng FY, Li Q, Ho CY, Lam TS, To Y, Lee WH, Li M, Chu KH, Toh M. (2010b). Cucurbitacin B induces apoptosis and S phase cell cycle arrest in BEL-7402 human hepatocellular carcinoma cells and is effective via oral administration. Cancer Lett, 294, 118–124.
- Chen JC, Chiu MH, Nie RL, Cordell GA, Qiu SX. (2005). Cucurbitacins and cucurbitane glycosides: Structures and biological activities. Nat Prod Rep, 22, 386–399.
- Cragg GM, Newman DJ. (2005). Plants as a source of anti-cancer agents. J Ethnopharmacol, 100, 72–79.
- Cury-Boaventura MF, Pompéia C, Curi R. (2004). Comparative toxicity of oleic acid and linoleic acid on Jurkat cells. Clin Nutr, 23, 721–732.
- Dantas IN, Gadelha GC, Chaves DC, Monte FJ, Pessoa C, de Moraes MO, Costa-Lotufo LV. (2006). Studies on the cytotoxicity of cucurbitacins isolated from Cayaponia racemosa (Cucurbitaceae). Z Naturforsch, C, J Biosci, 61, 643–646.
- Doskotch RW, Malik MY, Beal JL. (1969). Cucurbitacin B, the cytotoxic principle of Begonia tuberhybrida var. alba. Lloydia, 32, 115–122.
- Duncan KL, Duncan MD, Alley MC, Sausville EA. (1996). Cucurbitacin E-induced disruption of the actin and vimentin cytoskeleton in prostate carcinoma cells. Biochem Pharmacol, 52, 1553–1560.
- Fang X, Phoebe CH Jr, Pezzuto JM, Fong HH, Farnsworth NR, Yellin B, Hecht SM. (1984). Plant anticancer agents, XXXIV. Cucurbitacins from Elaeocarpus dolichostylus. J Nat Prod, 47, 988–993.
- Ferreira PM, Santos AG, Tininis AG, Costa PM, Cavalheiro AJ, Bolzani VS, Moraes MO, Costa-Lotufo LV, Montenegro RC, Pessoa C. (2010). Casearin X exhibits cytotoxic effects in leukemia cells triggered by apoptosis. Chem Biol Interact, 188, 497–504.
- Imboden JB. (1988). The regulation of intracellular signals during lymphocyte activation. Immunol Today, 9, 17–18.
- Jayaprakasam B, Seeram NP, Nair MG. (2003). Anticancer and antiinflammatory activities of cucurbitacins from Cucurbita andreana. Cancer Lett, 189, 11–16.
- Kumar V, Abbas AK, Fausto N, Robbins SL, Cotran RS. (2004). Pathology Basis of Disease. China: WB Saunders.
- Leist M, Jäättelä M. (2001). Four deaths and a funeral: From caspases to alternative mechanisms. Nat Rev Mol Cell Biol, 2, 589–598.
- Liu T, Zhang M, Zhang H, Sun C, Deng Y. (2008). Inhibitory effects of cucurbitacin B on laryngeal squamous cell carcinoma. Eur Arch Otorhinolaryngol, 265, 1225–1232.
- Magalhães HI, Ferreira PM, Moura ES, Torres MR, Alves AP, Pessoa OD, Costa-Lotufo LV, Moraes MO, Pessoa C. (2010). In vitro and in vivo antiproliferative activity of Calotropis procera stem extracts. An Acad Bras Cienc, 82, 407–416.
- Militão GC, Dantas IN, Pessoa C, Falcão MJ, Silveira ER, Lima MA, Curi R, Lima T, Moraes MO, Costa-Lotufo LV. (2006). Induction of apoptosis by pterocarpans from Platymiscium floribundum in HL-60 human leukemia cells. Life Sci, 78, 2409–2417.
- Musza LL, Speight P, McElhiney S, Barrow CJ, Gillum AM, Cooper R, Killar LM. (1994). Cucurbitacins, cell adhesion inhibitors from Conobea scoparioides. J Nat Prod, 57, 1498–1502.
- Sato DY, Wal R, de Oliveira CC, Cattaneo RI, Malvezzi M, Gabardo J, Buchi Dde F. (2005). Histopathological and immunophenotyping studies on normal and sarcoma 180-bearing mice treated with a complex homeopathic medication. Homeopathy, 94, 26–32.
- Scheuer PJ, Lefkowitch JH . (2000). Liver Biopsy Interpretation. England: WB Saunders.
- Shan XL, Zhou XY, Yang J, Wang YL, Deng YH, Zhang MX. (2010). [Inhibitory effect of cucurbitacin E on the proliferation of ovarian cancer cells and its mechanism]. Chin J Cancer, 29, 20–24.
- Shi X, Franko B, Frantz C, Amin HM, Lai R. (2006). JSI-124 (cucurbitacin I) inhibits Janus kinase-3/signal transducer and activator of transcription-3 signalling, downregulates nucleophosmin-anaplastic lymphoma kinase (ALK), and induces apoptosis in ALK-positive anaplastic large cell lymphoma cells. Br J Haematol, 135, 26–32.
- Smit HF, van den Berg AJ, Kroes BH, Beukelman CJ, Quarles van Ufford HC, van Dijk H, Labadie RP. (2000). Inhibition of T-lymphocyte proliferation by cucurbitacins from Picrorhiza scrophulariaeflora. J Nat Prod, 63, 1300–1302.
- Stuppner H, Muller EP, Wagner H. (1981). Cucurbitacins from Picrorhiza kurooa. Phytochemistry, 30, 305–310.
- Sun C, Zhang M, Shan X, Zhou X, Yang J, Wang Y, Li-Ling J, Deng Y. (2010). Inhibitory effect of cucurbitacin E on pancreatic cancer cells growth via STAT3 signaling. J Cancer Res Clin Oncol, 136, 603–610.
- Wakimoto N, Yin D, O’Kelly J, Haritunians T, Karlan B, Said J, Xing H, Koeffler HP. (2008). Cucurbitacin B has a potent antiproliferative effect on breast cancer cells in vitro and in vivo. Cancer Sci, 99, 1793–1797.
- Yasuda S, Yogosawa S, Izutani Y, Nakamura Y, Watanabe H, Sakai T. (2010). Cucurbitacin B induces G2 arrest and apoptosis via a reactive oxygen species-dependent mechanism in human colon adenocarcinoma SW480 cells. Mol Nutr Food Res, 54, 559–565.
- Zhang M, Zhang H, Sun C, Shan X, Yang X, Li-Ling J, Deng Y. (2009). Targeted constitutive activation of signal transducer and activator of transcription 3 in human hepatocellular carcinoma cells by cucurbitacin B. Cancer Chemother Pharmacol, 63, 635–642.