Abstract
Context: Recent ecological evidence has showed a lag-correlation between the prevalence of diabetes and consumption of niacin (nicotinamide and nicotinic acid) in the US. Nicotinamide has been demonstrated to induce insulin resistance due to excess reactive oxygen species and methyl depletion, whereas the effect of nicotinic acid is poorly understood.
Objective: To examine the mechanism of the effect of nicotinic acid on glucose metabolism.
Materials and methods: Rats were injected with different cumulative doses of nicotinic acid (0.5, 2, 4 g/kg) and nicotinamide (2 g/kg). A glucose tolerance test was given 2 h after the final injection. The role of methyl consumption and reactive oxygen species generation were evaluated by measuring N1-methylnicotinamide and hydrogen peroxide.
Results: Cumulative doses of nicotinic acid produced a dose-dependent increase in the plasma levels of N1-methylnicotinamide and hydrogen peroxide, which was associated with a decrease in liver and skeletal muscle glycogen levels. At the same dosage (2 g/kg), in comparison with nicotinamide, nicotinic acid was weaker in raising plasma N1-methylnicotinamide levels (0.7 ± 0.11 µg/mL vs. 4.69 ± 0.24 µg/mL, P < 0.001), but stronger in increasing plasma hydrogen peroxide levels (1.88 ± 0.07 µmol/L vs. 1.55 ± 0.05 µmol/L, P < 0.001). Moreover, nicotinamide, unlike nicotinic acid, did not reduce liver glycogen levels.
Discussion and conclusion: This study suggested that excessive nicotinic acid, like nicotinamide, might induce methyl consumption, oxidative stress and insulin resistance. Long-term consumption high niacin may increase the risk of type 2 diabetes.
Introduction
Type 2 diabetes, a risk factor for cardiovascular disease, has been increasing worldwide over the last few decades at an alarming rate, not only in adults but also in children and adolescents (CitationChen et al., 2012). Increased plasma glucose levels and decreased insulin sensitivity (i.e., insulin resistance) are the hallmarks of type 2 diabetes. Increasing evidence indicates that insulin resistance can be caused by oxidative stress due to overproduction of reactive oxygen species (ROS) (CitationHoustis et al., 2006; CitationHenriksen et al., 2011; CitationZhou et al., 2011). Therefore, to understand where excessive ROS originates from may be of great importance in determining the etiology of type 2 diabetes.
Diet has long been known to play a role in the development of type 2 diabetes. More recently, it has been suspected that the prevalence of obesity, the risk factor for type 2 diabetes, may be due to changes in the global food system (CitationSwinburn et al., 2011). As shown in , there have been significant increases in consumption of B vitamins in the US since the implementation of mandatory B vitamins fortification (i.e., addition of thiamine, riboflavin, and niacin to foods) in the early 1940s.
Figure 1. The trends of the US per capita daily consumption of B vitamins. (a–d) Average US per capita daily consumption of B vitamins during 1909–2004. (Data were derived from the Economic Research Service of the US Department of Agriculture, http://www.ers.usda.gov/Data/FoodConsumption/NutrientAvailIndex.htm, accessed March 29, 2012). RDA, Recommended Dietary Allowances; EAR, Estimated Average Requirements.
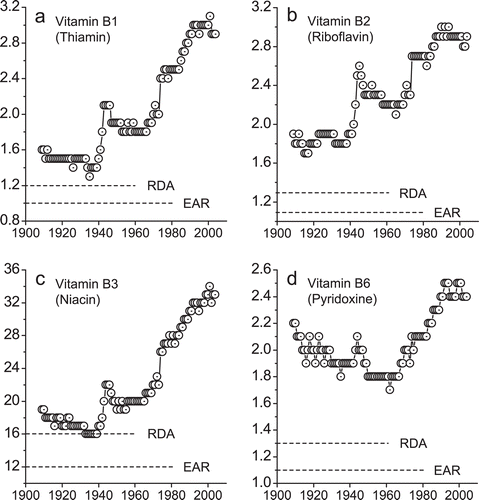
However, little is known about the relationship between B vitamins fortification and increased prevalence of type 2 diabetes. Our recent study provided the first ecological evidence indicating that the prevalence of obesity and type 2 diabetes in the US is strongly correlated with the per capita consumption of niacin (CitationZhou et al., 2010). This raises the possibility that obesity and type 2 diabetes may involve excess niacin intake.
Niacin refers to both nicotinic acid and its amide derivative, nicotinamide. Both nicotinic acid and nicotinamide have been known to induce glucose intolerance (CitationInstitute of Medicine, 1998; CitationGinsberg, 2006). Our previous studies have demonstrated that excessive nicotinamide can lead to methyl group depletion by increasing methyl group consumption, and to oxidative stress by increasing ROS generation (CitationZhou et al., 2009; CitationLi et al., 2010; CitationSun et al., 2012). However, it is not known whether nicotinic acid works using a similar mechanism. The purpose of the present brief study is to examine this issue.
Materials and methods
Chemicals
Nicotinic acid and nicotinamide were purchased from Sigma (St. Louis, MO, USA).
Animals and experimental designs
Adult male Sprague–Dawley rats weighing 180–220 g were purchased from Dalian Medical University Animal Center. Rats were housed under standard laboratory conditions (22 ± 2°C, 12/12 h light–dark cycle with lights on from 6:00 a.m. to 6:00 p.m.) and given access to food and water ad libitum. All animal experiments were performed according to the NIH Guide for Care and Use of Laboratory Animals, and were approved by the Animal Ethics Committee of China Medical University (CMU/2010/0005).
Rats were divided randomly into five groups (n = 7 in each) and fasted for 14 h before the experiments: a control group (saline injection, i.p.), three nicotinic acid-treated groups (100, 400 or 800 mg/kg, i.p.), and a nicotinamide-treated group (400 mg/kg, i.p.). The injection was repeated every 2 h for a total of five doses. Glucose (2 g/kg, i.p.) was given 2 h after the final injection. Samples of plasma, liver and gastrocnemius muscle were collected and stored 2 h after glucose load, as previously described (CitationZhou et al., 2009).
Assays of glycogen, insulin, and hydrogen peroxide
Liver and muscle glycogen contents were determined with Glycogen Assay Kits (Nanjing Jiancheng Bioengineering Institute, Nanjing, China). Plasma insulin was measured by radioimmunoassay using commercial kits (Beijing North Institute of Biological Technology, Beijing, China). Hydrogen peroxide (H2O2) concentrations in rat plasma were measured using H2O2 Assay Kit (Beyotime Biotechnology, Jiangsu, China).
Determination of N1-methylnicotinamide
N1-Methylnicotinamide was analyzed, as previously described (CitationZhou et al., 2009), using a high performance liquid chromatography system that consisted of an LC-9A pump (Shimadzu, Kyoto, Japan), a Rheodyne 7725i sample injector with a 20 µL sample loop (Rheodyne LLC, Rohnert Park, CA, USA), a Hypersil ODS C18 column (Thermo, Bellefonte, PA, USA) and a Waters 470 fluorescence detector (Milford, MA, USA).
Statistical analysis
The data are presented as means ± SE. Statistical differences in the data were evaluated by a Student’s t-test or one-way ANOVA as appropriate, and were considered significant at P < 0.05.
Results
Effects of nicotinic acid and nicotinamide on N1-methylnicotinamide generation
N1-Methylnicotinamide is a methylated intermediate metabolite of nicotinamide. The conversion of nicotinamide to N1-methylnicotinamide is a methyl group consuming process (CitationZhou et al., 2009). The more N1-methylnicotinamide is generated, the more methyl groups are consumed. As shown in , both nicotinic acid and nicotinamide significantly increased the level of plasma N1-methylnicotinamide. The effect was more profound in nicotinamide-treated rats. The level of plasma N1-methylnicotinamide in nicotinamide-treated rats was almost five times higher than that of in nicotinic acid-treated rats under equivalent exposure conditions. These results indicated that both nicotinamide and nicotinic acid increase the consumption of methyl groups, and that nicotinamide can consume more methyl groups than nicotinic acid.
H2O2 generating-effect of nicotinic acid and nicotinamide
Oxidative stress implies an increased production of ROS. H2O2 is a major ROS in living organisms (CitationChang et al., 2004). We therefore investigated whether nicotinic acid could induce oxidative stress by measuring H2O2 generation. Our previous studies demonstrated that excessive nicotinamide increases the production of H2O2 (CitationZhou et al., 2009; CitationLi et al., 2010). The present results showed that high nicotinic acid exposure also increased the plasma H2O2 levels (). This suggests that excessive nicotinic acid degradation is a ROS generating process.
Comparison of the effects of nicotinic acid and nicotinamide on glucose metabolism
Insulin resistance is a reduced capacity of peripheral tissues to respond to physiological levels of circulating insulin. Defective muscle glycogen synthesis is a major cause for insulin resistance (CitationAbdul-Ghani & DeFronzo, 2010). We therefore compared glucose-induced glycogen synthesis in rat livers and skeletal muscles with or without exposure to nicotinic acid or nicotinamide. As shown in , both nicotinic acid- and nicotinamide-treated rats showed a low skeletal muscle glycogen content 2 h after glucose load, compared with the control group. Nicotinic acid decreased rat liver glycogen contents in a dose-dependent manner. In contrast, nicotinamide increased liver glycogen content. High cumulative nicotinic acid exposure showed a high level of plasma insulin.
Discussion
Type 2 diabetes involves oxidative stress, insulin resistance and epigenetic changes: the latter are linked to methyl metabolism (CitationZhou et al., 2011). Our recent ecological analysis has suggested that increased prevalence of obesity and type 2 diabetes might involve niacin consumption, primarily due to fortification of grain with niacin (CitationZhou et al., 2010). If niacin plays a causal role in obesity and type 2 diabetes, it should induce oxidative stress and insulin resistance, and affect methyl metabolism. In fact, nicotinamide and nicotinic acid have been known to induce glucose intolerance (CitationInstitute of Medicine, 1998) and insulin resistance (CitationKahn et al., 1989; CitationGreenbaum et al., 1996; CitationChang et al., 2006), although the role of long-term high exposure to niacin in the development of type 2 diabetes remains unsolved. Our previous studies demonstrated that nicotinamide can cause oxidative stress (CitationZhou et al., 2009; CitationLi et al., 2010) and deletion of methyl groups (CitationSun et al., 2012), which it is proposed accounts for insulin resistance and epigenetic changes in type 2 diabetes (CitationZhou et al., 2011). The present results showed that nicotinic acid also increased the generation of H2O2 and N1-methylnicotinimade, the latter being a methyl group consuming process (CitationSun et al., 2012). These findings further supported the hypothesis that chronic high niacin exposure may be a potent risk factor for type 2 diabetes.
Nicotinic acid and nicotinamide come from different dietary sources: dietary nicotinic acid is derived from plant foods, while nicotinamide is derived from animal foods. Fortified grains have provided an additional nicotinamide source, as nicotinamide is typically used for food supplements and food fortification. Moreover, there are some differences between the degradation of nicotinic acid and nicotinamide (CitationZhou et al., 2011). Excessive nicotinamide is rarely excreted into the urine, and has to be converted to N1-methylnicotinamide before elimination (CitationKnip et al., 2000), whereas nicotinic acid can be either converted to nicotinamide and then degraded via the nicotinamide catabolic pathway, or excreted in the urine unchanged and as nicotinuric acid (CitationMenon et al., 2007). This may explain why nicotinamide-treated rats have much higher plasma N1-methylnicotinamide levels than nicotinic acid-treated rats. Since the methylation of niacin increases the consumption of the body’s methyl groups which are also necessary for other methylation reactions, including DNA methylation (CitationZhou et al., 2011). Thus, it is conceivable that chronic high niacin exposure might induce methyl pool depletion, and thus play a role in DNA methylation-mediated epigenetic changes, but further studies are needed to confirm this.
It should be noted that nicotinamide exposure increases the content of rat liver glycogen, while nicotinic acid exposure decreases it. This suggests that nicotinamide may increase the synthesis of glycogen in rat liver. Since nicotinamide is the primary precursor of nicotinamide adenine dinucleotide, an essential coenzyme in many enzymes in glucose metabolism, the increase in liver glycogen synthesis by nicotinamide may involve changes in the activity of glycogen synthetic enzymes. Interestingly, evidence has indicated that obesity is associated with increased hepatic glycogen content (CitationAllick et al., 2004) on the one hand, and on the other hand, that the sharp increase in the prevalence of obesity has occurred following the worldwide spread of mandatory fortification with nicotinamide (CitationZhou et al., 2010). Thus, it may be possible that an excess nicotinamide-induced increase in hepatic glycogen synthesis may play a role in the development of obesity. Studying the relationship between excessive nicotinamide intake and hepatic glycogen synthesis might be a promising way to gain more insight into the pathophysiology of obesity.
Taken together, high niacin exposure may be a major risk for type 2 diabetes. Because niacin fortification is a major risk factor for excess niacin intake, it therefore seems that urgent, large-scale controlled studies are needed to assess the safety of fortification-induced high niacin consumption.
Declaration of interest
This study was supported by National Natural Science Foundations of China (No. 31140036, 81000575, 30971065).
References
- Abdul-Ghani MA, DeFronzo RA. (2010). Pathogenesis of insulin resistance in skeletal muscle. J Biomed Biotechnol, 2010, 476279.
- Allick G, Sprangers F, Weverling GJ, Ackermans MT, Meijer AJ, Romijn JA, Endert E, Bisschop PH, Sauerwein HP. (2004). Free fatty acids increase hepatic glycogen content in obese males. Metab Clin Exp, 53, 886–893.
- Chang AM, Smith MJ, Galecki AT, Bloem CJ, Halter JB. (2006). Impaired beta-cell function in human aging: Response to nicotinic acid-induced insulin resistance. J Clin Endocrinol Metab, 91, 3303–3309.
- Chang MC, Pralle A, Isacoff EY, Chang CJ. (2004). A selective, cell-permeable optical probe for hydrogen peroxide in living cells. J Am Chem Soc, 126, 15392–15393.
- Chen L, Magliano DJ, Zimmet PZ. (2012). The worldwide epidemiology of type 2 diabetes mellitus–present and future perspectives. Nat Rev Endocrinol, 8, 228–236.
- Ginsberg HN. (2006). Niacin in the metabolic syndrome: More risk than benefit? Nat Clin Pract Endocrinol Metab, 2, 300–301.
- Greenbaum CJ, Kahn SE, Palmer JP. (1996). Nicotinamide’s effects on glucose metabolism in subjects at risk for IDDM. Diabetes, 45, 1631–1634.
- Henriksen EJ, Diamond-Stanic MK, Marchionne EM. (2011). Oxidative stress and the etiology of insulin resistance and type 2 diabetes. Free Radic Biol Med, 51, 993–999.
- Houstis N, Rosen ED, Lander ES. (2006). Reactive oxygen species have a causal role in multiple forms of insulin resistance. Nature, 440, 944–948.
- Institute of Medicine. (1998). Niacin. In: Food and Nutrition Board, ed. Dietary Reference Intakes for Thiamin, Riboflavin, Niacin, Vitamin B6, Folate, Vitamin B12, Pantothenic Acid, Biotin, and Choline. Washington, DC: National Academy Press, 123–149.
- Kahn SE, Beard JC, Schwartz MW, Ward WK, Ding HL, Bergman RN, Taborsky GJ Jr, Porte D Jr. (1989). Increased beta-cell secretory capacity as mechanism for islet adaptation to nicotinic acid-induced insulin resistance. Diabetes, 38, 562–568.
- Knip M, Douek IF, Moore WP, Gillmor HA, McLean AE, Bingley PJ, Gale EA; European Nicotinamide Diabetes Intervention Trial Group. (2000). Safety of high-dose nicotinamide: A review. Diabetologia, 43, 1337–1345.
- Li D, Sun WP, Zhou YM, Liu QG, Zhou SS, Luo N, Bian FN, Zhao ZG, Guo M. (2010). Chronic niacin overload may be involved in the increased prevalence of obesity in US children. World J Gastroenterol, 16, 2378–2387.
- Menon RM, González MA, Adams MH, Tolbert DS, Leu JH, Cefali EA. (2007). Effect of the rate of niacin administration on the plasma and urine pharmacokinetics of niacin and its metabolites. J Clin Pharmacol, 47, 681–688.
- Sun WP, Li D, Lun YZ, Gong XJ, Sun SX, Guo M, Jing LX, Zhang LB, Xiao FC, Zhou SS. (2012). Excess nicotinamide inhibits methylation-mediated degradation of catecholamines in normotensives and hypertensives. Hypertens Res, 35, 180–185.
- Swinburn BA, Sacks G, Hall KD, McPherson K, Finegood DT, Moodie ML, Gortmaker SL. (2011). The global obesity pandemic: Shaped by global drivers and local environments. Lancet, 378, 804–814.
- Zhou SS, Li D, Sun WP, Guo M, Lun YZ, Zhou YM, Xiao FC, Jing LX, Sun SX, Zhang LB, Luo N, Bian FN, Zou W, Dong LB, Zhao ZG, Li SF, Gong XJ, Yu ZG, Sun CB, Zheng CL, Jiang DJ, Li ZN. (2009). Nicotinamide overload may play a role in the development of type 2 diabetes. World J Gastroenterol, 15, 5674–5684.
- Zhou SS, Li D, Zhou YM, Sun WP, Liu QG. (2010). B-Vitamin consumption and the prevalence of diabetes and obesity among the US adults: Population based ecological study. BMC Public Health, 10, 746.
- Zhou SS, Zhou YM, Li D, Lun YZ. (2011). Dietary methyl-consuming compounds and metabolic syndrome. Hypertens Res, 34, 1239–1245.