Abstract
Context: Silymarin, a flavonolignan from Silybum marianum (L.) Gaertn. (Asteraceae), has been reported to have antioxidant and anti-inflammatory properties. Therefore, it may be worthwhile to study the effect of silymarin on wound healing.
Objective: To evaluate the effect of silymarin on human fibroblast cells in an in vitro model of wound healing.
Materials and methods: Human fibroblast cells were treated with different concentrations (4.5, 9, 18, 36 µg/mL) of silymarin. The effects of silymarin on cell viability, proliferation, collagen synthesis, and expression of cyclooxygenase-2 (COX-2) and inducible nitric oxide synthetase (iNOS) were assessed by 3-(4,5-dimethylthiazol-2-yl)-2,5-diphenyltetrazolium bromide, 5-bromo-2′-deoxy-uridine, hydroxyproline analysis and real-time PCR, respectively. The effect of silymarin on cellular antioxidant status was determined by protection against hydrogen peroxide (H2O2)-induced cell injury and free radical scavenging activity (ABTS assay) of the cells.
Results: Results of the present study indicate that pretreatment of fibroblast cells with silymarin significantly protected cells against H2O2-induced injury (p < 0.05). After an 18 h treatment of cells with 36 µg/mL silymarin, total antioxidant capacity of cells significantly increased (p < 0.05). Furthermore, pretreatment of human fibroblast cells with silymarin significantly inhibited lipopolysaccharide (LPS)-induced COX-2 mRNA expression (p < 0.001). There was no significant difference in fibroblast proliferation and collagen synthesis between treatment and control groups (p > 0.05).
Discussion and conclusion: Silymarin may be useful as a therapeutic agent for the treatment of cutaneous wounds through its antioxidation and anti-inflammation effects.
Introduction
Wound healing is a complex process characterized by inflammation, cell proliferation and remodeling of the extracellular matrix (CitationDiegelmann & Evans, 2004). Fibroblast cells play an important role in wound healing by initiating the proliferative phase of repair. These cells secrete the collagens and the glycosaminoglycans of the new granulation tissue and subsequently affect the remodeling of the granulation tissue into mature dermis (CitationSinger & Clark, 1999).
Impairment in each of wound healing processes can lead to healing delay or even the inability for dermal wound healing. Reactive oxygen species (ROS) that are produced in response to cutaneous injury may impair the healing process (CitationGupta et al., 2002). Furthermore, the prolonged inflammation phase may lead to cell destruction and altered composition of extracellular matrix with subsequent failure in epithelization (CitationDovi et al., 2003; CitationGutiérrez-Fernández et al., 2007). Hence, if a compound has antioxidant and anti-inflammation potential, it can be a good therapeutic agent for accelerating the wound healing process (CitationHoughton et al., 2005). Among the inflammatory pathways, nitric oxide synthase (NOS) and cyclooxygenase (COX) and their products play an important role in wound healing (CitationStallmeyer et al., 1999; CitationAbd-El-Aleem et al., 2001). Human fibroblasts express two NOS isoforms, the constitutively expressed endothelial NOS (eNOS) and the inducible NOS (iNOS) form. iNOS is involved in provoking and maintaining inflammatory phase (CitationSoneja et al., 2005). COX enzymes control the initial steps of the inflammation process by converting arachidonic acid to prostaglandins (PGs) and thromboxane. Like NOS, COX has two isoforms. COX-1, a constitutive enzyme, is expressed at a constant level and does not fluctuate in response to various stimuli. COX-2, an inducible enzyme, is up-regulated in the early inflammatory response and produce a large amount of proinflammatory PGs at the inflammatory site (CitationFutagami et al., 2002).
Silymarin, a mixture of bioactive flavonolignans obtained from Silybum marianum (L.) Gaertn. (Asteraceae), possesses strong antioxidant, anti-inflammatory and anticancer activity (CitationKatiyar et al., 1997, 2002; CitationMeeran et al., 2006; CitationGu et al., 2007). It also modulates many molecular changes caused by xenobiotics and ultraviolet radiation to protect the skin (CitationZhao et al., 2000; CitationKatiyar, 2002; CitationMeeran et al., 2006; Svobodova et al., 2006, 2007).
Although silymarin has antioxidant and anti-inflammation effects, its effect on wound healing has not been investigated to date. The aim of the present study was to investigate the effect of silymarin on human fibroblast cells in an in vitro model of wound healing.
Materials and methods
Materials
Human neonatal foreskin fibroblast cells were obtained from Shaheed Beheshti Skin Research Center. Silymarin, lipopolysaccaride (LPS; Escherichia coli 0111:B4), tumor necrosis factor-α (TNF-α), phorbol 12-myristate 13-acetate, and 3-(4,5-dimethylthiazol-2-yl)-2,5-diphenyltetrazolium bromide (MTT) were purchased from Sigma (Seelze, Germany). Cell culture reagents were purchased from GIBCO Life Technologies (Paisley, UK) and all the other reagents were obtained from Sigma.
Cell culture and treatment
Human fibroblast cells were grown in Dulbecco modified Eagle’s medium supplemented with 10% fetal bovine serum, 100 U/mL penicillin G sodium, 100 g/mL streptomycin sulfate and l-glutamine, at 37°C in a humidified 5% CO2 atmosphere. Cells were treated with serial concentrations (4.5, 9, 18, 36 µg/mL) of silymarin that was dissolved in 100% DMSO.
Fibroblast proliferation and viability assay
Assessment of cell growth was examined by 3-(4,5-dimethylthiazol-2-yl)-2,5-diphenyltetrazolium bromide (MTT) assay. Briefly, human fibroblast cells (2 × 104 well) were seeded in 96-well plates and treated with different concentrations of silymarin for 24 and 48 h. Cells were incubated for an additional 3 h with MTT, followed by treatment with 100 μL of DMSO for 1 h to solubilize formazan violet crystals in the cells. The absorbance of each well was determined by spectrophotometer at dual wavelengths of 540 and 620 nm with a microplate reader.
MTT tetrazolium assay may lead to false positive results when testing natural compounds with intrinsic reductive potential (CitationPeng et al., 2005). Therefore, cells were washed several times before adding MTT.
Cell proliferation was also measured by determining the extent of 5-bromo-2′-deoxy-uridine (BrdU) incorporation into DNA of human fibroblast cells (ELISA kit, Roche, Germany). The amounts of BrdU incorporated into the fibroblast cells were assayed according to the manufacturer’s instructions.
Protection against damage to fibroblasts by hydrogen peroxide
The method used for the hydrogen peroxide (H2O2) assay was described by CitationAnnan and Houghton (2008). Fibroblast cells were seeded at 4 × 103 cells/well in 96-well plates and incubated at 37°C until near confluence. The concentration of H2O2 inducing about 50% inhibition of the MTT response in fibroblasts was determined to be 2.5 × 10−3 M H2O2 and was used for all experiments.
To assess the protective effect of silymarin on cells, three different types of experiments were performed. In the first experiment, the cells were pretreated with silymarin overnight and then were treated with H2O2 for 3 h. In the second experiment, the cells were pretreated with silymarin overnight and then were treated with silymarin and H2O2 simultaneously for 3 h. In the third experiment, cells were treated with silymarin and H2O2 simultaneously for 3 h.
Catalase (250 IU/mL), a scavenger of H2O2, was used as the positive control in all experiments. After the incubation period, the MTT assay was used to quantify the degree of protection of fibroblast cells by silymarin against H2O2 damage.
Determination of total antioxidant capacity
Fibroblast cells (2 × 106) were seeded in 25 cm2 flask and treated with different concentrations of silymarin for 3 and 18 h. After treatment, cells were scraped, pelleted and re-suspended in 1 mL of 5 mM phosphate buffer, pH: 7.4. Cell membranes were disrupted by sonication on ice and the cell lysates centrifuged at 4°C, 10,000g, for 15 min. The supernatant was separated from cell debris and used for total antioxidant assay measurement. The results were normalized to the protein content of the cell lysates.
Cellular antioxidant levels were determined by measuring the ability of antioxidants in the sample to inhibit the oxidation of ABTS (2,2′-azino-di-[3-ethylbenzthiazoline sulphonate]) to the ABTS+ radical by H2O2 with the Total Antioxidant Assay Kit (Cayman, USA). According to manufacturer’s instructions, the amount of ABTS+ radical was monitored by reading the absorbance at 405 nm. Torolox concentrations from 0 to 0.35 mM were used to make a standard curve. The capacity of the antioxidants in the samples to prevent ABTS+ oxidation were determined according to the standard curve (CitationMiller et al., 1993).
Measurement of collagen synthesis by hydroxyproline analyses
The assay was performed following the protocol published by CitationStipcevic et al. (2006) with small modification. Briefly, cells were seeded at a density of 2 × 106 in 75 cm2 flask and treated with different concentrations of silymain for 12 days. Ascorbic acid solution was added every day in concentration of 50 µg/mL to the medium. After 12 days, cells were harvested by rubber policeman in 1 mL of 10 mM PBS. Cell membranes were disrupted by sonicator and total protein amount was quantified using CitationBradford (1976). For quantifying hydroxyproline, 0.5 mL of sonicated samples was mixed with 0.5 mL of 12 N HCl and incubated in 115°C oven for 18 h. The residue was re-suspended in 250 μL deionized water and 50 μL was mixed with 450 μL of deionized water to perform assay. The incubation of samples were as follows: 20 min incubation with 250 μL of 0.05 M chloramine T solution [chloramine T solution = 1.41 g chloramine T (Sigma–Aldrich,) dissolved in 20 mL dH2O, 30 mL methyl cellosolve (2-methoxyethanol) and 50 mL citric acid buffer]; 5 min incubation with 250 μL of 3.15 M perchloric acid; 20 min incubation with 250 μL of 20% p-dimethylbenzaldehyde in 60°C water bath dissolved in methyl cellosolve to a final volume of 50 mL and heated to 60°C to dissolve the reagent. Absorbance was measured at 540 nm. Hydroxyproline (Sigma–Aldrich) concentrations from 0 to 10 µg/mL were used to make a standard curve.
COX-2 and iNOS mRNA expression analysis
Human fibroblast cells (5 × 105 cells/well) were seeded in 6-well plates and allowed to grow for 24 h in a growth medium at 37°C and 5% CO2. Cells were then washed twice with PBS and then pretreated with silymarin at various concentrations for 18 h. After pretreatment, LPS (10 µg/mL) was added, and the cells were incubated at 37°C and 5% CO2 for 24 h. For iNOS induction, LPS (1–10 µg/mL) plus TNF-α (20–50 ng/mL) or LPS (1–10 µg/mL) plus phorbol 12-myristate 13-acetate (PMA) (20–50 ng/mL) were used.
Total cellular RNA was extracted from cells using RNeasy protect cell mini kit (Qiagen, Hilden, Germany) and its quality was controlled. Complementary DNA (cDNA) was synthesized from 1 µg of total RNA using QuantiTect Rev. Transcription kit (Qiagen, Hilden, Germany) according to manufacturer’s instructions. Real-time PCR was performed using SYBR premix Ex Taq (Qiagen, Hilden, Germany) and predesigned primers for COX-2, iNOS, and GAPDH genes (QuantiTect Primer Assay, Qiagen, MD, USA). Each cycle consisted of denaturation at 95°C for 10 s, and combined annealing and extension at 60°C for 30 s. A standard curve was generated from dilution series constructed from pooled cDNA. Relative changes in gene expression were quantified using ΔΔCT method.
Statistical analysis
Analysis of variance was used to compare means in different groups. Values of less than 0.05 were considered significant (*p < 0.05, **p < 0.01, ***p < 0.001). Data were expressed as mean ± SE.
Results
Effect of silymarin on the viability and proliferation of human fibroblast cells
The effects of silymarin on the viability and proliferation of human fibroblast cells were examined by the MTT assay and by measuring the amounts of BrdU incorporated into cellular DNA in the presence of serial concentrations of silymarin for 24 and 48 h are shown in .
Figure 1. Effect of silymarin on cellular viability and proliferation of human fibroblast cells. Cells were treated with different concentrations of silymarin for 24 and 48 h. (A) Cell viability was determined by the MTT assay. (B) Cell proliferation was determined by DNA (BrdU) incorporation assay. The data are expressed as mean ± SE from at least three separate experiments.

Results showed that silymarin does not have any cytotoxic and proliferative effect on fibroblast cells in the studied concentration range (4.5–36 µg/mL) and the data obtained by the cell proliferation ELISA assay are well correlated with those obtained by MTT assay.
Effect of silymarin on fibroblast injury by H2O2
As shown in and , when the cells were pretreated with silymarin overnight before the application of the H2O2, the MTT assay showed that the cells were protected. However, when cells were incubated with different concentrations of silymarin and H2O2 simultaneously for 3 h at 37°C, it was observed that fibroblast cells were not protected against H2O2 damage by various concentrations of the silymarin ().
Figure 2. Effect of silymarin on hydrogen peroxide-induced damage in fibroblasts using different treatment regimens. (A) Pretreatment only. Fibroblasts were incubated with various concentrations of silymarin overnight before exposure to hydrogen peroxide without silymarin for 3 h. (B) Fibroblasts were pretreated with silymarin overnight and then exposed to hydrogen peroxide and silymarin for 3 h. (C) Fibroblasts were incubated with silymarin and hydrogen peroxide simultaneously for 3 h. The data are expressed as mean ± SE of three separate experiments. Treated cells were compared with cells exposed only to hydrogen peroxide. *p < 0.0.05, **p < 0.01, ***p < 0.001.
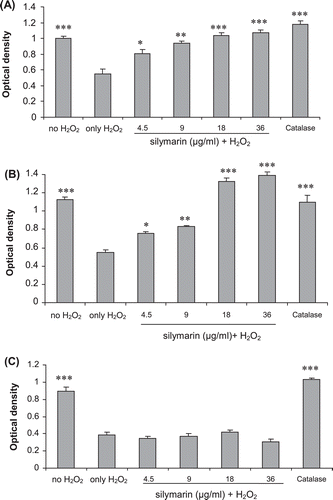
Effect of silymarin on fibroblast total antioxidant capacity
The antioxidant capacity of silymarin was evaluated according to the ABTS decoloration. As shown in , incubation of silymarin led to increase in total antioxidant capacity of cell extract (reaching statistical significant after 18 h incubation).
Figure 3. Total antioxidant capacity of cell extracts after 3 and 18 h incubation of the cells with various concentrations of silymarin. Total antioxidant capacity was measured by monitoring of ABTS solution decolorization in supernatants of cell homogenates after incubation with silymarin for various times. Results represent mean ± SE, *p < 0.05 compared to the control group.
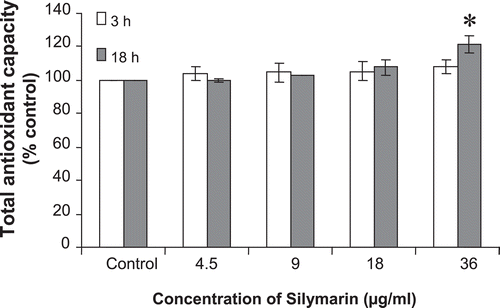
Effect of silymarin on fibroblast collagen synthesis
Collagen synthesis was determined by measuring hydroxyproline levels after fibroblast treatment with different concentrations of silymarin is shown in . The results demonstrated that silymarin has no effect on collagen synthesis compared to the control group (p > 0.05).
Effect of silymarin on LPS-induced COX-2 expression
Incubation with LPS significantly increased the expression of COX-2 mRNA in fibroblast cells. Through this study, iNOS mRNA was not induced in human fibroblast cells regardless of inflammatory stimuli. As it is evident in , pretreatment with silymarin significantly inhibits LPS-induced mRNA expression of COX-2 (p < 0.001).
Figure 5. Real-time RT-PCR measured effect of silymarin on COX-2 mRNA expression of human fibroblast cells. Cells were incubated first with indicated concentrations of silymarin for 18 h and then in the absence or presence of LPS (10 µg/mL) for 24 h. The COX-2 mRNA level was normalized by GAPDH RNA level. Data are mean ± SE of triplicates from an experiment repeated three times. ***p < 0.001 compared with cells treated only with LPS.
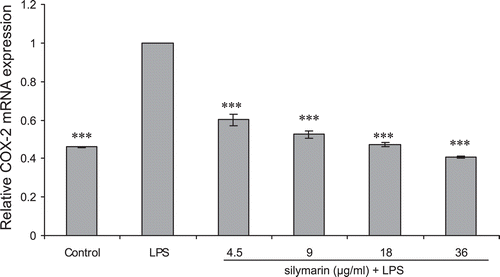
Discussion
Wound healing is categorized into three phases: inflammatory phase (including homeostasis and inflammation), proliferative phase (including granulation, contraction and epithelialization) and the remodeling phase which ultimately determines the appearance of the healed tissue and its strength (CitationDiegelmann & Evans, 2004).
Regulation of inflammation and oxidation are important in the process of wound healing. Production of free radicals in response to cutaneous injury, may delay the healing process through the destruction of lipids, proteins and extracellular matrix elements (CitationRusso et al., 2002). Antioxidant activity of silymarin was previously demonstrated in vitro and in vivo. Most of these reported antioxidant activities were based on cell-free biochemical tests (CitationPsotová et al., 2001; CitationAsghar & Masood, 2008; CitationTurgut et al., 2008; CitationKöksal et al., 2009).
In the present study, we have found that silymarin has a protective effect on cells against H2O2. The fibroblast cells that were pretreated with silymarin were well protected against H2O2 while cells treated with silymarin and H2O2 simultaneously were not protected. These results suggest that the protection is not due to direct interaction of the silymarin and H2O2. Therefore, the protective effect of silymarin could be attributed to an indirect effect by altering the cell membrane and limiting the damage induced by H2O2. Also in this study, we used a cell-based in vitro assay for testing the antioxidant activities of silymarin. Similarly, total antioxidant capacity was significantly increased in cells that were treated with silymarin for 18 h. These findings further support that silymarin has an indirect effect on ROS condition. It was demonstrated that a number of endogenous antioxidants, including GSH, superoxide dismutase (SOD), catalase, glutathione peroxidase (GPx), gluthatione reductase, and glucose 6-phosphate dehydrogenase can be induced by silymarin (CitationRamakrishnan et al., 2006). Oral administration of silymarin has been shown to reduce lipid peroxidation of brain tissues in acetaminophen-induced toxicity by enhancing antioxidant enzymes (CitationNencini et al., 2007). Silymarin also inhibited tetradecanoyl phorbol-13-acetate (TPA)-induced lipid peroxidation and depletion of antioxidant enzymes such as SOD, catalase and GPx in mouse skin (CitationLahiri-Chatterjee et al., 1999; CitationZhao et al., 1999). Furthermore, CitationToklu et al. (2007) showed that administration of silymarin was effective against burn-induced oxidative damage and depressed TNF-α and reversed malondialdehyde and glutathione levels in rats.
On the other hand, a prolonged inflammatory phase may lead to cell destruction and altered composition of the extracellular matrix with subsequence of epithelization (CitationGutiérrez-Fernández et al., 2007). One of the early responses to inflammatory stimuli in cells which is involved in the repair processes is the induction of COX-2 (CitationFutagami et al., 2002). Upregulation of COX-2 is involved in the persistent inflammation in chronic wounds. Studies show that high COX-2 expression causes a delay in healing and production of a scar (CitationWilgus et al., 2003, 2004). The present investigation has clearly shown that silymarin pretreatment attenuated the LPS-induced COX-2 mRNA expression in skin fibroblasts. Previously, it was found that silymarin down-regulates COX-2 induction in macrophage cell line J774A.1 (CitationRaso et al., 2001). Furthermore, it has been shown that silymarin is a potent and specific inhibitor of nuclear transcription factor κB, and also modulates immune response, by augmenting synthesis of anti-inflammatory cytokines, such as IL-10, IL-12 (CitationSchümann et al., 2003; CitationMeeran et al., 2006). Silymarin also inhibits chemically induced expression of TNF-α and interleukin-1α (IL-1α) and reduces TPA-induced of myeloperoxidase, lipoxygenase, and COX-2 activities in mouse skin (CitationZhao et al., 1999; CitationZi et al., 1997).
Fibroblast viability and proliferation are important in the granulation formation in wound healing. Fibroblasts secrete collagens and subsequently affect the remodeling of granulation tissue into mature dermis (CitationLau et al., 2007). In this study, results of BrdU incorporation and MTT assays revealed that silymarin had no effect on fibroblast proliferation in the studied concentration range.
In addition, by measuring hydroxyproline content, we found that silymarin did not exhibit a significant effect on collagen content in fibroblast cell culture. It was shown previously that silibinin, the major component of silymarin, has no effect on type I collagen synthesis in vitro (Abul CitationHashem et al., 2008).
Conclusion
In conclusion, this study showed that silymarin has antioxidant and anti-inflammatory effects in human fibroblast cells, but does not have any effect on total collagen and cell proliferation. Therefore, silymarin may be useful as a therapeutic agent for the treatment of cutaneous wounds through antioxidation and anti-inflammation effects.
Declaration of interest
This article is a part of the PhD dissertation which was financially supported by Tehran University of Medical Sciences (Grant No. 88-1-30-8602) and Food and Drug Control Laboratory and Research Center. The authors report no conflict of interest.
References
- Abd-El-Aleem SA, Ferguson MWJ, Appleton I, Bhowmick A, Mccollum CN. Ireland GW. (2001). Expression of cyclooxygenase isoforms in normal human skin and chronic venous ulcers. J Pathol, 195, 616–623.
- Hashem MA, Jun KY, Lee E, Lim S, Choo HY, Kwon Y. (2008). A rapid and sensitive screening system for human type I collagen with the aim of discovering potent anti-aging or anti-fibrotic compounds. Mol Cells, 26, 625–630.
- Annan K, Houghton PJ. (2008). Antibacterial, antioxidant and fibroblast growth stimulation of aqueous extracts of Ficus asperifolia Miq. and Gossypium arboreum L., wound-healing plants of Ghana. J Ethnopharmacol, 119, 141–144.
- Asghar Z, Masood Z. (2008). Evaluation of antioxidant properties of silymarin and its potential to inhibit peroxyl radicals in vitro. Pak J Pharm Sci, 21, 249–254.
- Bradford MM. (1976). A rapid and sensitive method for the quantitation of microgram quantities of protein utilizing the principle of protein-dye binding. Anal Biochem, 72, 248–254.
- Diegelmann RF, Evans MC. (2004). Wound healing: An overview of acute, fibrotic and delayed healing. Front Biosci, 9, 283–289.
- Dovi JV, He LK, DiPietro LA. (2003). Accelerated wound closure in neutrophil-depleted mice. J Leukoc Biol, 73, 448–455.
- Futagami A, Ishizaki M, Fukuda Y, Kawana S, Yamanaka N. (2002). Wound healing involves induction of cyclooxygenase-2 expression in rat skin. Lab Invest, 82, 1503–1513.
- Gu M, Singh RP, Dhanalakshmi S, Agarwal C, Agarwal R. (2007). Silibinin inhibits inflammatory and angiogenic attributes in photocarcinogenesis in SKH-1 hairless mice. Cancer Res, 67, 3483–3491.
- Gupta A, Singh RL, Raghubir R. (2002). Antioxidant status during cutaneous wound healing in immunocompromised rats. Mol Cell Biochem, 241, 1–7.
- Gutiérrez-Fernández A, Inada M, Balbín M, Fueyo A, Pitiot AS, Astudillo A, Hirose K, Hirata M, Shapiro SD, Noël A, Werb Z, Krane SM, López-Otín C, Puente XS. (2007). Increased inflammation delays wound healing in mice deficient in collagenase-2 (MMP-8). FASEB J, 21, 2580–2591.
- Houghton PJ, Hylands PJ, Mensah AY, Hensel A, Deters AM. (2005). In vitro tests and ethnopharmacological investigations: Wound healing as an example. J Ethnopharmacol, 100, 100–107.
- Katiyar SK. (2002). Treatment of silymarin, a plant flavonoid, prevents ultraviolet light-induced immune suppression and oxidative stress in mouse skin. Int J Oncol, 21, 1213–1222.
- Katiyar SK, Korman NJ, Mukhtar H, Agarwal R. (1997). Protective effects of silymarin against photocarcinogenesis in a mouse skin model. J Natl Cancer Inst, 89, 556–566.
- Köksal E, Gülçin I, Beyza S, Sarikaya O, Bursal E. (2009). In vitro antioxidant activity of silymarin. J Enzyme Inhib Med Chem, 24, 395–405.
- Lahiri-Chatterjee M, Katiyar SK, Mohan RR, Agarwal R. (1999). A flavonoid antioxidant, silymarin, affords exceptionally high protection against tumor promotion in the SENCAR mouse skin tumorigenesis model. Cancer Res, 59, 622–632.
- Lau TW, Chan YW, Lau CP, Chan CM, Lau CB, Fung KP, Leung PC, Ho YY. (2007). Investigation of the effects of Chinese medicine on fibroblast viability: implications in wound healing. Phytother Res, 21, 938–947.
- Meeran SM, Katiyar S, Elmets CA, Katiyar SK. (2006). Silymarin inhibits UV radiation-induced immunosuppression through augmentation of interleukin-12 in mice. Mol Cancer Ther, 5, 1660–1668.
- Miller NJ, Rice-Evans C, Davies MJ, Gopinathan V, Milner A. (1993). A novel method for measuring antioxidant capacity and its application to monitoring the antioxidant status in premature neonates. Clin Sci, 84, 407–412.
- Nencini C, Giorgi G, Micheli L. (2007). Protective effect of silymarin on oxidative stress in rat brain. Phytomedicine, 14, 129–135.
- Peng L, Wang B, Ren P. (2005). Reduction of MTT by flavonoids in the absence of cells. Colloids Surf B Biointerfaces, 45, 108–111.
- Psotová J, Zahálková J, Hrbác J, Simánek V, Bartek J. (2001). Determination of total antioxidant capacity in plasma by cyclic voltammetry. Two case reports. Biomed Pap Med Fac Univ Palacky Olomouc Czech Repub, 145, 81–83.
- Ramakrishnan G, Raghavendran HR, Vinodhkumar R, Devaki T. (2006). Suppression of N-nitrosodiethylamine induced hepatocarcinogenesis by silymarin in rats. Chem Biol Interact, 161, 104–114.
- Raso GM, Meli R, Di Carlo G, Pacilio M, Di Carlo R. (2001). Inhibition of inducible nitric oxide synthase and cyclooxygenase-2 expression by flavonoids in macrophage J774A.1. Life Sci, 68, 921–931.
- Russo A, Longo R, Vanella A. (2002). Antioxidant activity of propolis: Role of caffeic acid phenethyl ester and galangin. Fitoterapia, 73 Suppl 1, S21–S29.
- Schümann J, Prockl J, Kiemer AK, Vollmar AM, Bang R, Tiegs G. (2003). Silibinin protects mice from T cell-dependent liver injury. J Hepatol, 39, 333–340.
- Singer AJ, Clark RA. (1999). Cutaneous wound healing. N Engl J Med, 341, 738–746.
- Soneja A, Drews M, Malinski T. (2005). Role of nitric oxide, nitroxidative and oxidative stress in wound healing. Pharmacol Rep, 57 Suppl, 108–119.
- Stallmeyer B, Kämpfer H, Kolb N, Pfeilschifter J, Frank S. (1999). The function of nitric oxide in wound repair: inhibition of inducible nitric oxide-synthase severely impairs wound reepithelialization. J Invest Dermatol, 113, 1090–1098.
- Stipcevic T, Piljac J, Vanden Berghe D. (2006). Effect of different flavonoids on collagen synthesis in human fibroblasts. Plant Foods Hum Nutr, 61, 29–34.
- Svobodová A, Walterová D, Psotová J. (2006). Influence of silymarin and its flavonolignans on H(2)O(2)-induced oxidative stress in human keratinocytes and mouse fibroblasts. Burns, 32, 973–979.
- Svobodová A, Zdarilová A, Malisková J, Mikulková H, Walterová D, Vostalová J. (2007). Attenuation of UVA-induced damage to human keratinocytes by silymarin. J Dermatol Sci, 46, 21–30.
- Toklu HZ, Tunali-Akbay T, Erkanli G, Yüksel M, Ercan F, Sener G. (2007). Silymarin, the antioxidant component of Silybum marianum, protects against burn-induced oxidative skin injury. Burns, 33, 908–916.
- Turgut F, Bayrak O, Catal F, Bayrak R, Atmaca AF, Koc A, Akbas A, Akcay A, Unal D. (2008). Antioxidant and protective effects of silymarin on ischemia and reperfusion injury in the kidney tissues of rats. Int Urol Nephrol, 40, 453–460.
- Wilgus TA, Bergdall VK, Tober KL, Hill KJ, Mitra S, Flavahan NA, Oberyszyn TM. (2004). The impact of cyclooxygenase-2 mediated inflammation on scarless fetal wound healing. Am J Pathol, 165, 753–761.
- Wilgus TA, Vodovotz Y, Vittadini E, Clubbs EA, Oberyszyn TM. (2003). Reduction of scar formation in full-thickness wounds with topical celecoxib treatment. Wound Repair Regen, 11, 25–34.
- Zhao J, Lahiri-Chatterjee M, Sharma Y, Agarwal R. (2000). Inhibitory effect of a flavonoid antioxidant silymarin on benzoyl peroxide-induced tumor promotion, oxidative stress and inflammatory responses in SENCAR mouse skin. Carcinogenesis, 21, 811–816.
- Zhao J, Sharma Y, Agarwal R. (1999). Significant inhibition by the flavonoid antioxidant silymarin against 12-O-tetradecanoylphorbol 13-acetate-caused modulation of antioxidant and inflammatory enzymes, and cyclooxygenase 2 and interleukin-1α expression in SENCAR mouse epidermis: Implications in the prevention of stage I tumor promotion. Mol Carcinog, 26, 321–333.
- Zi X, Mukhtar H, Agarwal R. (1997). Novel cancer chemopreventive effects of a flavonoid antioxidant silymarin: inhibition of mRNA expression of an endogenous tumor promoter TNF alpha. Biochem Biophys Res Commun, 239, 334–339.