Abstract
Context: The number of patients with cancer is increasing. New therapeutic agents to overcome drug-resistant tumors are urgently needed. Cyrtosperma johnstonii N.E. Br. (Araceae) is used for treatment of cancer in Thai traditional medicine. This study aimed to evaluate antioxidant activity and cytotoxicity of C. johnstonii extracts on human cancer cells.
Materials and methods: Dried powder of C. johnstonii rhizomes was extracted with several solvents. The 0.1 mg/ml extract solution was tested for antioxidant activity by 2,2′-azinobis-3-ethylbenzothiazoline-6-sulfonic acid (ABTS) and ferric reducing antioxidant power (FRAP) assays. Color formation from 3-(4,5-dimethylthiazol-2-yl)-2,5-diphenyltetrazolium bromide was used to determine cell viability. Standardization of the extract was performed by high-performance liquid chromatography (HPLC) with photodiode array detector at 254 and 360 nm. Cell cycle arrest was evaluated by flow cytometry after 5 min, 12 h and 24 h treated with 20 µg/ml of the acetone extract.
Results: The acetone extract exhibited the highest phenolic content and antioxidant activity (TEAC and EC values = 19.2 ± 0.14 and 19.2 ± 0.31 mM/mg, respectively). The IC50 values for leukemia ranged from 11 ± 1 to 29 ± 3 µg/ml and from 5 ± 2 to 6 ± 0 µg/ml for small cell lung carcinoma cells. Cell cycle arrest occurred at the G2/M phase followed by apoptosis. HPLC analysis revealed that rutin is the major constituents of the extract.
Discussion and conclusion: The acetone extract of C. johnstoni is a promising source of natural antioxidants and anticancer. The extract inhibits cancer cells effectively with less effect on normal cells.
Introduction
It is well known that cancer treatments based on chemotherapeutic agents, surgery, and radiation are not fully effective in many cases. Recurrence of cancer frequently occurs due to resistance of cancers to chemotherapeutic agents (CitationRyu et al., 2011; CitationBroxterman et al., 2009). Therefore, new therapeutic approaches to fight drug-resistant tumors are urgently needed. Recent studies have shown that biologically active oxygen species (ROS) formed in vivo are involved in the development of cancer (CitationHalliwell, 1998). ROS play a role in the pathogenesis of cancer by causing damage to DNA. The unrepaired-damaged DNA can lead to the formation of malignant tumors by the accumulation of somatic mutations (CitationTsao et al., 2004). Antioxidants can reduce ROS levels and have the potential to prevent cancer occurrence (CitationClerkin et al., 2008). Many tropical plant species have high antioxidant activity and have the potential to inhibit carcinogenesis (CitationOkonogi et al. 2007; CitationKapadia et al., 2003; CitationKhan & Sultana, 2005). The proposed mechanisms of action of plant extracts include carcinogen inactivation, induction of apoptosis and/or differentiation, cell cycle arrest, inhibition of angiogenesis, and reversal of multidrug resistance, or combinations of these mechanisms (CitationZhang et al., 2004).
C. johnstonii N.E. Br. (Araceae) is a rhizome plant commonly grown in Thailand and also found in other Asian countries like Malaysia and Indonesia. The rhizome of this plant has been used in Thai traditional medicine for treatment of many diseases including several types of cancer. The effective ethno-medicinal use of C. johnstonii suggests that it might have antioxidant and cytotoxicity properties. To verify these assumptions, we have studied the rhizome extracts of C. johnstonii in several in vitro models of antioxidant and anticancer activity. Ideally, suitable therapeutic agents would selectively kill tumor cells without toxic effect on normal cells. Therefore, cytotoxicity of C. johnstonii extracts to normal cells was also investigated in this study.
Materials and methods
Plant materials
Rhizomes of C. johnstonii were collected in September, 2010 from the central part of Thailand. A voucher specimen (No 010112) was deposited in the Herbarium of the Faculty of Pharmacy, Chiang Mai University (CMU), Thailand and authenticated by the CMU staff botanist.
Preparation of plant extracts
Dried powder of C. johnstonii rhizomes was sequentially fractionated by extracting with hexane, ethyl acetate, acetone, and methanol, respectively. The filtrate of each solvent was gathered and evaporated under vacuum in a rotary evaporator at 45°C. The obtained extracts were kept in vacuum desiccators overnight to remove residual solvent. The percentage yield of the dried extract obtained from each solvent was found to be 1.6, 0.7, 1.5, and 2.5% wt/wt for hexane, ethyl acetate, acetone, and methanol, respectively.
Antioxidant activity tests
Free-radical scavenging assay
This assay measures the ROS scavenging activity of compounds based on the decolorization of 2,2′-azinobis-3-ethylbenzothiazoline-6-sulfonic acid (ABTS) radical cation. The assay was performed as described by CitationTachakittirungrod et al. (2007). Briefly, ABTS free radicals were generated by reaction of ABTS and potassium persulfate in solution. The free radical solution was diluted to an absorbance reading of 0.7 ± 0.2 at 750 nm and subsequently stored in the dark for 12 h. The test extracts were dissolved in ethanol and diluted to a concentration of 0.1 mg/ml. The samples were mixed with the ABTS free radical solution in 96-well plates. The absorbance of the mixture was measured using a microtitre plate reader. Vitamin E and butylated hydroxyl toluene (BHT) were used as positive controls. A water soluble analogue of vitamin E (Trolox) was used for calibration. The antioxidant potential is expressed as Trolox equivalent antioxidant capacity (TEAC) in mM of a Trolox solution whose antioxidant capacity is equivalent to 1.0 mg of the extract.
Ferric reducing antioxidant power (FRAP) assay
The assay measures the reduction of ferric ion by the tested extracts using a spectrophotometer with adaptable microplates. The FRAP reagent was freshly prepared by mixing 2,4,6-tri(2-pyridyl)-S-triazine and FeCl3 in acetate buffer (200 mM, pH 3.6). The test extracts in ethanol at a concentration of 0.1 mg/ml were reacted with the FRAP reagent in 96-well plates for 5 min. A microtitre plate reader was used to measure the absorbance of the solutions. The reducing antioxidant power was expressed as an equivalent concentration (EC) value. This value is defined as a ferric reducing ability of the test samples equivalent to that of 1 mM FeSO4.
Determination of total phenolic content (TPC)
TPC of C. johnstonii extracts was determined according to a modification of the Folin-Ciocalteu colorimetric method (CitationKhonkarn et al., 2010). Briefly, at a concentration of 0.1 mg/ml, the tested extract solution (20 µl) was mixed with Folin-Ciocalteu reagent (45 µl) and sodium carbonate (2.7 × 103 µg, 135 µl). The mixtures were incubated for 2 h at room temperature in the dark. The developed blue color was measured on a microtitre plate reader. TPC of each sample was calculated using gallic acid for calibration and expressed as gallic acid equivalent (GAE) concentration (mg/ml).
Test of cytotoxicity against cancer cells
Human erythromyelogenous leukemia cells (K562), the corresponding drug-resistant cells with P-glycoprotein (P-gp) overexpression (K562/ADR), small cell lung carcinoma cells (GLC4) and the corresponding drug-resistant cells with multidrug resistance-associated protein (MRP1) overexpression (GLC4/ADR) were the cancer cells tested. The cells were suspended in 1 ml medium and were seeded into the 24-well plates at a density of 5 × 104 cells/well. After 24-h incubation, solutions with different concentrations of the extracts (final concentration of the extracts ranged from 5 to 200 μg/ml) were added and the cells were cultured further for 72 h. The numbers of cells were counted by flow cytometry (Coulter® Epics® XLTM). The 50% inhibitory concentration (IC50) was determined from the dose response curves of percentages of cell growth inhibition versus the concentration of the extracts.
Cancer cell cycle progression analysis
Cells were exposed to 20 µg/ml acetone extract of C. johnstonii at 37°C for 5 min to 24 h and subsequently washed with phosphate-buffered saline (PBS). Next, the cells were fixed overnight with 70% ethanol, washed with PBS and stained for 30 min in the dark at 37°C with propidium iodide (PI) in PBS (10 µg/ml), also containing RNase (0.2 mg/ml) and 0.1% of Triton X-100. The cell cycle progression and cellular kinetics were investigated by flow cytometry (Coulter® Epics® XLTM) and the data were analyzed by the Origin software program. The number of cells in different cell cycle phases (G0/G1, S, G2/M) was calculated using DNA content histograms as described in our previous report (CitationKhonkarn et al., 2011).
Test of cytotoxicity against normal cells
Peripheral blood mononuclear cells (PBMC) were isolated from freshly drawn blood of normal human volunteers. The cytotoxicity of the extracts towards normal PBMC was tested using the MTT assay (CitationAlley et al., 1988) with some modification. Briefly, PBMC suspended in 100 µl medium were plated into the wells of 96-well plates (105 cells/well). After 24-h incubation, various concentrations (0.5–100 μg/ml) of the extracts were added and further incubated for 48 h. The supernatant (100 μl) was removed and 15 μl of 5 mg/ml MTT dye in PBS was added to each well. After 4 h, the supernatant was removed, 200 μl DMSO was added and mixed thoroughly to dissolve the dye crystals. The absorbance at 540 nm was measured with a microtitre plate reader. The percentage of cell viability for each extract was calculated using the following equation.
HPLC analysis
The extract solution (10 mg/ml) was filtered through a 0.45-µm filter (nylon syringe filter; Filtrex, Virginia Beach, VA, USA). The sample was analyzed using a Dionex ICS-3000 HPLC system with PDI-100 photodiode array detector. A C-18 column (150 × 4.6 mm, 5 µm particle size, Nucleodur®) with guard column was used. For the elution of the constituents, a gradient of two solvents denoted as A and B was applied. A was 2% vol/vol acetic acid in water whereas B was 2% vol/vol acetic acid in methanol. The gradient programme was as follows: 0 min 3% eluent B, 7 min 10% eluent B, 7.1 min 20% eluent B, 17 min 30% eluent B, 27 min 50% eluent B, 35 min 0% eluent B. The flow rate was 0.5 ml/min at room temperature and the injection volume was 20 µl. Rutin was used as a standard phenolic compound. The retention times (Rt) and UV spectra of major peaks were recorded at 254 and 360 nm.
Statistical analysis
The experiments were done in triplicate and the results are expressed as mean ± SD. Statistical analysis was done using one-way ANOVA with a p value of 95% confidence limit.
Results
Antioxidant activity
shows the results of the ABTS assay. The acetone extract showed the highest free radical scavenging activity with a TEAC value of 19.2 mM/mg which was approximately three times higher than that of the methanol extract (6.9 mM/mg) and about 12 and 34 times higher than the respective values of the ethyl acetate (1.5 mM/mg) and hexane (0.6 mM/mg) extracts. The reducing power shown in revealed that the highest EC value was obtained from the acetone extract (19.24 mM/mg) whereas the hexane extract had the lowest activity (0.06 mM/mg). The EC values of the ethyl acetate and the methanol extracts were 0.67 and 6.03 mM/mg, respectively. The results of reducing activity in the FRAP assay was in accordance with those of free radical scavenging activity in ABTS assay indicating that the acetone extract possessed the most potent antioxidant activity.
TPC
The values of TPC are shown in . The highest TPC was found in the acetone extract with a GAE value of 1.8 mg/ml. The TPC values of the methanol, ethyl acetate, and hexane extracts were substantially lower than that of the acetone extract with GAE values of 0.5, 0.3, and 0.2 mg/ml, respectively. The plots of TPC vs. TEAC and TPC vs. EC showed good linear relationship with R2 = 0.967 and 0.976, respectively. Moreover, the relationships between TPC vs. the cytotoxicity toward resistant leukemia (K562/ADR) and small cell lung carcinoma (GLC4/ADR) cells at 50% inhibitory concentration (IC50) were found to be in a good linear relationship with R2 = 0.840 and 0.818, respectively.
Cytotoxicity to cancer cells
C. johnstonii extracts obtained with the different solvents exerted different levels of cytotoxicity to the tested cancer cells. The acetone and ethyl acetate extracts exhibited the highest cytotoxic activity toward sensitive leukemic cells with an IC50 value of 11 µg/ml. For drug-resistant cancer cells, only the acetone extract showed strong cancer cell inhibition with an IC50 value of 29 ± 3 µg/ml as shown in . For small cell lung carcinoma cells, the acetone extract showed the highest level of cytotoxicity observed against cancer cells in the present study, with IC50 values of 6 ± 0 and 5 ± 2 µg/ml for drug sensitive and resistant cancer cells, respectively ().
Table 1. Cytotoxic activity of C. johnstonii extracts against leukemia cells.
Table 2. Cytotoxic activity of C. johnstonii extracts against small cell lung carcinoma cells.
Cytotoxicity to normal cells
Ideally, suitable anticancer agents should have potent antitumor activity but not cause serious damage to healthy normal cells. All extracts were subjected to the cytotoxicity test against normal PBMC. shows that the hexane extract did not influence the viability of the cells. The acetone extract slightly reduced the viability of PBMC but, even considering the higher limit of the SD at 50 µg/ml, the decrease in viability was not >30%. In contrast, the ethyl acetate and methanol extracts more strongly decreased the viability of PBMC.
Cancer cell cycle progression
Because of the highest antioxidant and anticancer activities of the acetone extract, this extract was selected for further study on the mechanism of cancer cell growth inhibition and phytochemical identification. Flow cytometry analysis with PI-stained cells was performed to determine the mechanism of the growth inhibition of the tested cancer cell lines. The percentages of sensitive K562 leukemia and GLC4 small cell lung carcinoma cells in each phase of the cell cycle were evaluated and the results are shown in and . As compared to controls, the percentage in G2/M phase of the K562 cells treated with the acetone extract (20 µg/ml, from 5 min to 24 h) increased, whereas the numbers in G1 phase decreased. The subG1 fraction was commonly used as an indication of apoptotic-associated chromatin fragmentation. The subG1 population increased after K562 cells had been cultured with the acetone extract (12 h: 2.3%; 24 h; 7.1%) which suggested the sequential events of cell cycle arrest at G2/M phase followed by apoptosis. In the nonapoptotic population, we found that GLC4 also accumulated in G2/M phase after administration of the acetone extract. Besides, the subG1 group gradually increased with time (from 11.4% at 5 min to 25.6% at 24 h). This indicated that the acetone extract can induce cell cycle arrest in G2/M phase and apoptosis on GLC4 cells in a time-dependent manner.
Table 3. Cell cycle distribution of K562 and GLC4 incubated with 20 µg/ml acetone extract (SA) of C. johnstonii at 5 min, 12 h, and 24 h.
Figure 4 . Histograms of propidium iodide (PI)-associated K562 (left) and GLC4 (right) control cells and after incubation with 20 µg/ml acetone extract of C. johnstonii at 5 min, 12 h, and 24 h.
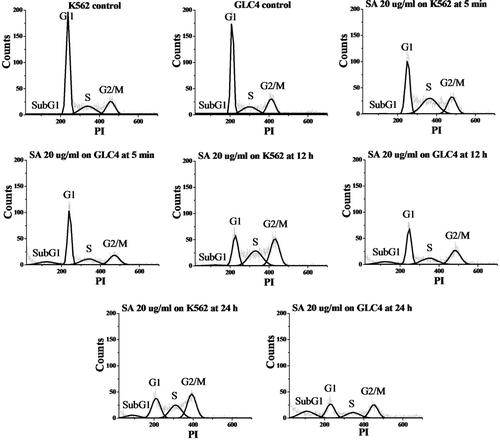
The same induction of cell cycle arrest and apoptosis was observed using resistant leukemia (K562/ADR) and small cell lung carcinoma (GLC4/ADR) cells exposed to the acetone extract, as shown in and . The numbers of K562/ADR cells in G2/M phase gradually increased upon incubation with the acetone extract (from 26.7% at 5 min to 36.6% at 24 h) whereas the G1 population decreased from 41.3 to 21.5%. The results show that K562/ADR cells are arrested in the G2/M phase by the acetone extract. The apoptotic nature of the extract-induced K562/ADR cell death was evident by the presence of a subG1 fraction (from 3.1% at 12 h to 10.7% at 24 h) in the flow cytometry analysis. Our data also demonstrated cell cycle arrest at G2/M phase and sequential apoptotic cell death in a time-dependent manner (from 2.0% at 5 min to 15.8% at 24 h) on GLC4/ADR cells after treatment with the acetone extract.
Table 4. Cell cycle distribution of K562/ADR and GLC4/ADR incubated with 20 µg/ml acetone extract (SA) of C. johnstonii at 5 min, 12 h, and 24 h.
HPLC analysis
shows two major peaks of the acetone extract absorbing at 254 and 360 nm with Rt of 26 and 32 min. The UV spectra of these two substances showed maxima at 255 and 354 nm as shown in the small graphs of . By comparison of Rt and absorption maxima with standard compounds, the results indicate that the HPLC peak at Rt 26 min corresponds to rutin.
Discussion
It is known that free radicals cause oxidative damage in biological systems, and antioxidants are known to delay or inhibit the free radical oxidation chain by donating hydrogen from the phenolic hydroxyl groups. In this study, we determined the total antioxidant capacities of C. johnstonii extracts by the ABTS and FRAP assays which provide a measure of two different mechanisms of antioxidant action, free radical scavenging and reducing power, respectively. Among four extracts of C. johnstonii rhizomes, the acetone extract showed the highest free radical scavenging and reducing power. Interestingly, the antioxidant activity of the acetone extract is significant higher (p value <0.05) than that of vitamin E and BHT. The correlation between the TEAC and EC values of the different extracts of C. johnstonii demonstrated an excellent linear correlation (R2 = 0.999) indicating that the extracts act by both antioxidant mechanisms.
As far as can be ascertained, no previous studies have been done on phytochemistry of C. johnstonii. However, it has been reported that plants belonging to the genus Cyrtosperma contain carotinoids, i.e., β-carotene, α-carotene, and lutein (CitationEnglberger et al., 2008). In addition, it was reported that plants of the family Araceae, to which C. johnstonii belongs, contain certain polyphenols (CitationVan et al., 2006). We then determined the TPC of C. johnstonii extracts. The results indicate that TPC of the extracts obtained with different solvents range from 0.2 to 1.8 mg/ml. We are the first who demonstrate that the acetone extract of C. johnstonii is a rich source of phenolic compounds. Moreover, the good linear correlations between TPC vs. TEAC and TPC vs. EC suggest that the antioxidant activity by both mechanisms of the extracts is caused by polyphenolic compounds.
The results on cancer cell toxicity indicate that C. johnstonii extracts with different solvents have strong cytotoxicity against not only the sensitive but also against the resistant cancer cells. The acetone extract showed the strongest cytotoxicity to different cancer cells. CitationAmpasavate et al. (2010) reported that the potent anticancer activity of certain Thai edible plant extracts had IC50 <50 µg/ml. The acetone extract of C. johnstonii has excellent anticancer potency as it shows IC50 values to all tested cancer cell lines ranging from 5 to 29 µg/ml. More interestingly, the present study found that the acetone and hexane extracts have higher cytotoxicity to resistant GLC4/ADR than to sensitive GLC4 cells (with resistance factor <1) whereas the extracts have mild activity against normal cells. This result is similar to the report of CitationAnazetti et al. (2003) that dehydrocrotonin and its derivative inhibited HL60 cell growth, but caused only mild damage to PBMC. Therefore, the acetone extract of C. johnstonii is an interesting natural source for potential novel cytotoxic agents against cancer cells.
Previous studies have shown that medicinal plant extracts affect cancer cell growth through several mechanisms. For example, the extracts from Oplopanax horridus (Sm.) Miq. (Araliaceae) effectively inhibit the proliferation of breast cancer cells via induction of cell cycle arrest (CitationWang et al., 2010). The extracts of Citrus grandis Osbeck (Rutaceae) and Onosma paniculatum Bur. & Franch. (Boraginaceae) induce apoptosis in leukemia cells (CitationKim et al., 2010; CitationRinner et al., 2010). We therefore further investigated the effect on cell cycle progression of the acetone extract of C. johnstonii as it showed the most cytotoxic activities. The results indicate the sequential occurrence of cell cycle arrest at G2/M phase followed by apoptosis of sensitive K562 leukemia and GLC4 small cell lung carcinoma cells. Similar results of this induction of cell cycle arrest and apoptosis were obtained in drug-resistant cancer cells (K562/ADR and GLC4/ADR). It is noted that, at subG1 phase, the efficacy of induction of apoptotic cell death of C. johnstonii acetone extract with GLC4 small cell lung carcinoma cells (from 11.4% at 5 min to 25.6% at 24 h) was higher than that of the same extract with K562 leukemia cells (12 h: 2.3%; 24 h: 7.1%). This corresponds with the results of cytotoxicity indicating that the IC50 value of this extract on GLC4 cells (6 µg/ml) is lower than that on K562 cells (11 µg/ml). Moreover, induction of apoptosis by C. johnstonii acetone extract in resistant GLC4/ADR small cell lung carcinoma cells (from 2.0% at 5 min to 15.8% at 24 h) is also higher than that of the same extract in resistant K562/ADR leukemia cells (from 3.1% at 12 h to 10.7% at 24 h) which corresponds with the observed cytotoxicity (with IC50 values of 5 and 29 µg/ml, respectively). We conclude that the acetone extract of C. johnstonii has higher activity in vitro against small cell lung carcinoma than leukemia cells.
A good linear relationship of the cytotoxicity toward resistant leukemia (K562/ADR) and small cell lung carcinoma (GLC4/ADR) cells at 50% inhibitory concentration (IC50) with TPC proposes that the polyphenolic compounds in C. johnstonii extracts play a role in resistant cancer cell inhibitory action. Similar results on cancer inhibitory action of polyphenolic compounds were reported e.g. to inhibit lung metastasis in mice induced by B16Fl0 melanoma cells (CitationMenon et al., 1995). The present results on different cancer cell inhibitory potential of antioxidant polyphenolic compounds existing in C. johnstonii extracts support this anticancer mechanism of action.
As chemical components may vary depending on harvest seasons, plant origins, drying processes and other factors (CitationMahady et al., 2001), standardization using chromatographic fingerprints or standard compounds as markers is crucial in order to control the quality of extracts (CitationChang et al., 2008). In the present study, HPLC conditions were established to determine the major constituents of the acetone extract of C. johnstonii, and the UV spectra of representative peaks in HPLC chromatograms were assayed. The HPLC chromatogram shows two major peaks of the acetone extract at Rt of 26 and 32 min. Based on comparison of the resulting Rt and absorption maxima of some standard compounds, it is considered that rutin is present as a main constituent in acetone extracts of C. johnstonii. Rutin has been reported to possess antioxidant and antitumor activities. CitationYang et al. (2008) reports that rutin exhibits strong in vitro antioxidant properties. Moreover, it has been reported that rutin inhibits spleen leukemia tumor growth in aWEHI-3 leukemia murine model (CitationLin et al., 2009) and induces apoptosis in cultured human glioblastoma cells (CitationSantos et al., 2011). Recently, a study of rutin with DNA has been performed. DNA interacts with rutin in the form of a non-electroactive complex (CitationBian et al. 2011) or cooperative intercalative interaction (CitationTian et al., 2008). The HPLC peak at Rt 32 min does not correspond to any of the standard substances examined. However, the compound with Rt 32 min presumably has a flavonoid type skeleton (maximum UV spectra at ~250–280 and ~330–360 nm). This is in keeping with the acetone extract of C. johnstonii being a rich source of flavonoid- or phenolic-type compounds.
Conclusions
Based on the results of this study, it can be concluded that C. johnstonii rhizomes contain compounds with both antioxidant activity and cytotoxicity to cancer cells. Its acetone extract shows a high level of cytotoxicity against both drug sensitive and resistant leukemia and small cell lung carcinoma cells without serious toxicity to normal PBMC. Regarding the mechanism of cell death, the acetone extract affected the sequential events of cell cycle arrest at G2/M phase and apoptosis. These findings seem to justify the use of this plant in traditional medicine for cancer treatment. Further study will be directed towards an investigation of in vivo cytotoxicity in cancer-related animal models.
Acknowledgments
We are very thankful to Prof. Wim E. Hennink of Utrecht University for his valuable discussion. We also thank the Graduate school, Chiang Mai University for partial support.
Declaration of interest
The authors are grateful for the financial support of the National Research Council of Thailand (NRCT) and the Royal Golden Jubilee (RGJ) Grant by the Thailand Research Fund (TRF). The authors report no conflicts of interest. The authors alone are responsible for the content and writing of the paper.
References
- Alley MC, Scudiero DA, Monks A, Hursey ML, Czerwinski MJ, Fine DL, Abbott BJ, Mayo JG, Shoemaker RH, Boyd MR. (1988). Feasibility of drug screening with panels of human tumor cell lines using a microculture tetrazolium assay. Cancer Res, 48, 589–601.
- Ampasavate C, Okonogi S, Anuchapreeda S. (2010). Cytotoxicity from fruit plants against leukemic cell lines. Afr J Pharm Pharmacol, 4, 013–021.
- Anazetti MC, Melo PS, Durán N, Haun M. (2003). Comparative cytotoxicity of dimethylamide-crotonin in the promyelocytic leukemia cell line (HL60) and human peripheral blood mononuclear cells. Toxicology, 188, 261–274.
- Bian CL, Zeng QX, Yang LJ, Xiong HY, Zhang XH, Wang SF. (2011). Voltammetric studies of the interaction of rutin with DNA and its analytical applications on the MWNTs–COOH/Fe3O4 modified electrode. Sensor Actuator, 156, 615–620.
- Broxterman HJ, Gotink KJ, Verheul HM. (2009). Understanding the causes of multidrug resistance in cancer: a comparison of doxorubicin and sunitinib. Drug Resist Updat, 12, 114–126.
- Chang YX, Ding XP, Qi J, Cao J, Kang LY, Zhu DN, Zhang BL, Yu BY. (2008). The antioxidant-activity-integrated fingerprint: an advantageous tool for the evaluation of quality of herbal medicines. J Chromatogr A, 1208, 76–82.
- Choiprasert W, Dechsupa N, Kothan S, Garrigos M, Mankhetkorn S. (2010). Quercetin, quercetrin except rutin potentially increased pirarubicin cytotoxicity by non-competitively inhibiting the P-glycoprotein-and MRP1 function in living K562/adr and GLC4/adr cells. Am J Pharmacol Toxicol, 5, 24–33.
- Clerkin JS, Naughton R, Quiney C, Cotter TG. (2008). Mechanisms of ROS modulated cell survival during carcinogenesis. Cancer Lett, 266, 30–36.
- Englberger L, Schierle J, Kraemer K, Aalbersberg W, Dolodolotawake U, Humphries J, Graham R, Reid AP, Lorens A, Albert K, Levendusky A, Johnson E, Paul Y, Sengebau F. (2008). Carotenoid and mineral content of Micronesian giant swamp taro (Cyrtosperma) cultivars. J Food Comp Anal, 21, 93–106.
- Halliwell B. (1998). Biomarkers of oxidative damage associated with human disease. Pathophysiol, 5, 267–267.
- Kapadia GJ, Azuine MA, Sridhar R, Okuda Y, Tsuruta A, Ichiishi E, Mukainake T, Takasaki M, Konoshima T, Nishino H, Tokuda H. (2003). Chemoprevention of DMBA-induced UV-B promoted, NOR-1-induced TPA promoted skin carcinogenesis, and DEN-induced phenobarbital promoted liver tumors in mice by extract of beetroot. Pharmacol Res, 47, 141–148.
- Khan N, Sultana S. (2005). Chemomodulatory effect of Ficus racemosa extract against chemically induced renal carcinogenesis and oxidative damage response in Wistar rats. Life Sci, 77, 1194–1210.
- Khonkarn R, Okonogi S, Ampasavate C, Anuchapreeda S. (2010). Investigation of fruit peel extracts as sources for compounds with antioxidant and antiproliferative activities against human cell lines. Food Chem Toxicol, 48, 2122–2129.
- Khonkarn R, Mankhetkorn S, Hennink WE, Okonogi S. (2011). PEG-OCL micelles for quercetin solubilization and inhibition of cancer cell growth. Eur J Pharm Biopharm, 79, 268–275.
- Kim H, Moon JY, Mosaddik A, Cho SK. (2010). Induction of apoptosis in human cervical carcinoma HeLa cells by polymethoxylated flavone-rich Citrus grandis Osbeck (Dangyuja) leaf extract. Food Chem Toxicol, 48, 2435–2442.
- Lin JP, Yang JS, Lu CC, Chiang JH, Wu CL, Lin JJ, Lin HL, Yang MD, Liu KC, Chiu TH, Chung JG. (2009). Rutin inhibits the proliferation of murine leukemia WEHI-3 cells in vivo and promotes immune response in vivo. Leuk Res, 33, 823–828.
- Mahady GB, Fong HHS, Farnsworth NR. (2001). Botanical Dietary Supplements: Quality, Safety and Efficacy. Lisse, The Netherlands: Swets and Zeitlinger Publishers.
- Menon LG, Kuttan R, Kuttan G. (1995). Inhibition of lung metastasis in mice induced by B16F10 melanoma cells by polyphenolic compounds. Cancer Lett, 95, 221–225.
- Okonogi S, Duangrat C, Anuchpreeda S, Tachakittirungrod S, Chowwanapoonpohn S. (2007). Comparison of antioxidant capacities and cytotoxicities of certain fruit peels. Food Chem, 103, 839–846.
- Rinner B, Kretschmer N, Knausz H, Mayer A, Boechzelt H, Hao XJ, Heubl G, Efferth T, Schaider H, Bauer R. (2010). A petrol ether extract of the roots of Onosma paniculatum induces cell death in a caspase dependent manner. J Ethnopharmacol, 129, 182–188.
- Ryu CS, Kwak HC, Lee KS, Kang KW, Oh SJ, Lee KH, Kim HM, Ma JY, Kim SK. (2011). Sulfur amino acid metabolism in doxorubicin-resistant breast cancer cells. Toxicol Appl Pharmacol, 255, 94–102.
- Santos BL, Silva AR, Pitanga BPS, Sousa CS, Grangeiro MS, Fragomeni BO, Coelho PLC, Oliveira MN, Menezes-Filho NJ, Costa MFD, El-Bacha RS, Velozo ES, Sampaio GP, Freire SM, Tardy M, Costa SL. (2011). Antiproliferative, proapoptotic and morphogenic effects of the flavonoid rutin on human glioblastoma cells. Food Chem, 127, 404–411.
- Tachakittirungrod S, Okonogi S, Chowwanapoonpohn S. (2007). Study on antioxidant activity of certain plants in Thailand: Mechanism of antioxidant action of guava leaf extract. Food Chem, 103, 381–388.
- Tian X, Li F, Zhu L, Ye B. (2008). Study on the electrochemical behavior of anticancer herbal drug rutin and its interaction with DNA. J Electroanal Chem, 621, 1–6.
- Tsao AS, Kim ES, Hong WK. (2004). Chemoprevention of cancer. CA Cancer J Clin, 54, 150–180.
- Van NTH, Minh CV, Marinella DL, Tiziana S, Alessandra B. (2006). Secondary metabolites from Lasia spinosa (L.) Thw. (Araceae). Biochem Syst Ecol, 34, 882–884.
- Wang CZ, Aung HH, Mehendale SR, Shoyama Y, Yuan CS. (2010). High performance liquid chromatographic analysis and anticancer potential of Oplopanax horridus: comparison of stem and berry extracts. Fitoterapia, 81, 132–139.
- Yang J Guo J, Yuan J. (2008). In vitro antioxidant properties of rutin. LWT Food Sci Technol, 41, 1060–1066.
- Zhang S, Yang X, Morris ME. (2004). Flavonoids are inhibitors of breast cancer resistance protein (ABCG2)-mediated transport. Mol Pharmacol, 65, 1208–1216.