Abstract
Context: Cassia alata Linn. [syn. Senna alata (L.) Roxb.] (Caesalpiniaceae) is used for treating various disease conditions including diabetes but its mechanism(s) of action and active principles remain to be elucidated.
Objective: The antidiabetic principles were identified using an in vitro α-glucosidase inhibition study.
Materials and methods: The methanol extract of leaves of C. alata, which showed potent α-glucosidase inhibitory activity (IC50, 63.75 ± 12.81 µg/ml), was fractionated. Active fractions were taken for further analysis by a variety of techniques including HPLC and Combiflash chromatography. The identity of the isolated compounds was established by spectroscopic analysis while their potential antidiabetic activity was assessed by in vitro enzyme inhibition studies.
Results: The α-glucosidase inhibitory effect of the crude extract was far better than the standard clinically used drug, acarbose (IC50, 107.31 ± 12.31 µg/ml). A subsequent fractionation of the crude extract was made using solvents of ascending polarity (petroleum ether, chloroform, ethyl acetate, n-butanol and water). The ethyl acetate (IC50, 2.95 ± 0.47 µg/ml) and n-butanol (IC50, 25.80 ± 2.01 µg/ml) fractions which contained predominantly kaempferol (56.7 ± 7.7 µM) and kaempferol 3-O-gentiobioside (50.0 ± 8.5 µM), respectively, displayed the highest carbohydrate enzyme inhibitory effect.
Discussion: One of the possible antidiabetic mechanisms of action of C. alata is by inhibiting carbohydrate digestion. This is the first report on α-glucosidase activity of kaempferol 3-O-gentiobioside.
Conclusion: Considering the activity profile of the crude extract and isolated bioactive compounds, further in vivo and clinical studies on C. alata extracts and compounds are well merited.
Introduction
Diabetes is a metabolic disorder that arises from the body’s failure to produce sufficient amounts of insulin and/or development of resistance to insulin. The resulting high level of blood glucose is known to lead to a variety of disease conditions such as stroke, kidney failure, blindness, limb amputations, heart diseases, and birth defects (CitationWHO, 2012). To date, over 250 million people worldwide are known to suffer from diabetes. In the USA alone, 25.8 million children and adults (8.3% of the population) are known to have diabetes (CitationAmerican Diabetes Association, 2012) while the figure for the UK is about 2.9 million people (Diabetes UK, 2012). The disease is now also reported to be increasing in emerging and many developing countries at epidemic proportions. India is among the highest affected emerging economy country with 41 million diabetes cases reported in 2006 and a projected rise to 70 million by the year 2025 (CitationWHO, 2012). One of the drug treatment regimes for diabetes is based on lowering postprandial hyperglycemia through inhibition of key carbohydrate digesting enzymes in the small intestine. Accordingly, α-glucosidase enzyme is now a validated target for many antidiabetic drugs such as acarbose (CitationDonner 2006; Citationde Melo et al., 2006). During the last few years, research in our laboratories and many others worldwide focused on the identification of potent α-glucosidase enzyme inhibitors of natural-origin with potentially less adverse side effects than the clinically used drugs (CitationHabtemariam, 2011; CitationRoselli et al., 2012).
Cassia alata Linn. [synonym: Senna alata (L.) Roxb.] (Caesalpiniaceae) is a shrub commonly known as “Ath thora”, “Eth thora” (in Sri Lanka), candle bush/tree (Malaysia), candle stick, Carrion Crow Bush, Winged Senna, Empress Candle plant, Dadmardan (India), Roman Candle tress (Fiji), and ringworm shrub as the plant leaves have been effectively used to treat ringworm infections (CitationUSDA, 2012). The plant is native to South America but is now introduced and naturalized in many pantropical countries of Southeast Asia, Africa, and North America (CitationProtabase, 2012).
Apart from being cultivated for ornamental purposes, numerous recent reports suggest that C. alata is extensively used for treating gastrointestinal conditions, skin diseases, allergic reactions, internal and external infections, and inflammatory conditions. For an extensive review of ethnobotanical applications, readers are directed to the recent review literature by CitationHennebelle et al. (2009). The plant is also one of the most popular natural antidiabetic remedies in Africa (CitationAbo et al., 2008), Caribbean (CitationGirón et al., 1991) and India (CitationKhan & Yadava, 2010). In this connection, some preliminary in vivo studies have confirmed the antidiabetic potential of the 85% ethanol leaves extract as a reducer of blood sugar in streptozotocin-induced hyperglycemic animals (CitationPalanichamy et al., 1988) and other experimental models (CitationVillaseñor et al., 2002). Through the use of a major antidiabetic target, intestinal α-glucosidase enzyme, the present study was designed to isolate and characterize potential antidiabetic agents from the leaves of C. alata.
Materials and methods
Materials
Acarbose, yeast α-glucosidase (from Saccharomyces cerevisiae, 750 units), p-nitrophenyl-α-d-glucopyranoside (pNPG), Sephadex LH-20 and thin layer chromatographic analytical plates were purchased from Sigma (Sigma-Aldrich Chemical Company, Dorset, UK). HPLC solvents and other reagents were obtained from Fisher Scientific UK Ltd (Loughborough, UK). All other chemicals and reagents were of analytical grade.
General phytochemical analysis methodology
1H NMR, 13C NMR and 2D-NMR (COSY, NOESY, HSQC and HMBC) spectra were obtained on a JEOL 500 MHz instrument. Homonuclear 1H connectivities were determined by using the COSY experiment. One bond 1H–13C connectivities were determined with HSQC while two- and three-bond 1H–13C connectivities were determined by HMBC experiments. Chemical shifts were reported in δ (ppm) using the solvent (CD3OD) standard and coupling constants (J) were measured in Hz. A Waters Synapt G2 TOF mass spectrometer equipped with an electrospray positive ionisation probe was used. Data were acquired over a mass range 50–2500. The instrumental set up was as follows: lock spray correction was applied to each acquired data set using Leu-Enk; capillary 3.0 kV, sampling cone 30 V, extraction cone 39, source 120°C, desolvation temperature 500°C, cone gas flow 120 L/h and desolvation gas 1000 L/h. Samples dissolved in methanol were introduced via an Acquity UPLC system coupled to Acquity UPLC BEH C18 1.7 µm (2.1 × 50 mm) column. Either Silica gel 60 or Sephadex LH-20 was used as an adsorbent for open column chromatography. TLC analysis was routinely performed using CHCl3:MeOH mixtures as the mobile phase and compounds were visualized under UV light.
Plant material
Healthy leaves from plants growing wild were collected in April 2012 from Kottayam district, Kerala state, India. The location of the plant population lies between 09°θ 34.295′ north latitude and 76°θ 31.189′ east longitude, 1 km south of Kottayam town. A voucher specimen numbered 2525, following identification by Dr George K. Varghese, was deposited at CMS Herbarium, C. M. S. College, Kottayam.
Extraction and fractionation procedures
Dried powdered leaves of C. alata (500 g) were soaked in cold methanol for 2 weeks. Removal of the solvent under reduced pressure followed by freeze-drying resulted in 55 g of the crude extract. A portion of the crude extract (50 g) was suspended in 750 ml of water and successively extracted (3x) with solvents of increasing polarity: petroleum ether (750 ml, yield, 9.1 g), chloroform (750 ml yield, 15.1 g), ethyl acetate (750 ml yield, 3.9 g) and n-butanol (200 ml yield, 7.5 g), respectively. All fractions including the final water residue (yield, 11.8 g) were dried using a rotary evaporator and then freeze-dried.
HPLC analysis
An Agilent 1200 series gradient HPLC system composed of a degasser (G1322A), quaternary pump (G1322A), auto sampler (G1329A), thermostat column compartment (G1316A) maintained at 25°C and a diode array detector (G1315D) was used. Various concentrations of standard samples and plant extracts/fractions were injected (20 µL) onto a reverse phase column (Agilent-Eclipse Plus C18, 5 µm, 4.9 × 150 mm). The mobile phase was a mixture of water (A) and methanol (B). The composition of the mobile phase at a flow rate of 1 ml/min was rising from 10 to 90% B over a period of 50 min.
α-Glucosidase inhibition assay
Our previously published microtiter-based assay was used (CitationHabtemariam, 2011). All experiments were carried out in a total volume of 100 μL reaction mixture containing 25 μL each of 250 mM phosphate buffer (pH 6.8), 2.5 mM pNPG, experimental drugs and 1.2 U/mL α-glucosidase. After incubation of the plates at 37°C for 10 min, the absorbance of 4-nitrophenol released during the enzyme-catalysed hydrolysis reaction was measured at 405 nm using Multiskan plate reader (Thermo Labsystems, UK).
Isolation of compounds from the ethyl acetate fraction
The ethyl acetate fraction (3 g) was applied onto a silica gel column (30 cm × 3 cm) and elutions done with chloroform and methanol mixtures of increasing polarity. Four major fractions were obtained: I (2 L, 0–6% methanol in CHCl3), II (0.8 L, 7% methanol in CHCl3), III (0.9 L 9–40% methanol in CHCl3), IV (2.5 L 20–40% methanol in CHCl3). Fraction II (155 mg) was applied onto a Sephadex column (30 cm × 2.5 cm) and eluted with chloroform:methanol (1:1) mixture to yield three fractions: A (43 ml), B (44 ml) and C (200 ml). Upon drying, fraction C yielded pure kaempferol (39 mg). Similar treatment of fraction III (367 mg) resulted in the isolation of 158 mg of kaempferol.
Isolation of compounds from the n-butanol fraction
RediSep Rf silica Column (24 g; Presearch, Hampshire, UK) attached to a Teledyne ISCO flash Combi chromatography system (Nebraska, USA) was used for isolating the major constituent of the n-butanol fraction. Briefly, a solvent gradient system of 0–40% methanol in ethyl acetate was maintained for 20 min followed by an isocratic 40% methanol maintained for 10 min. A constant flow rate of 35 ml/min was used throughout the system. The chromatogram was monitored by observing absorbance at dual wavelengths: 214 and 254 nm. Fractions corresponding to the major peak, kaempferol 3-O-gentiobioside, was collected and taken to dryness (38 mg).
NMR data for kaempferol 3-O-gentiobioside
1H NMR (500 MHz, methanol-d4): δ 8.09 (2H, d, J = 9.05), 6.88 (2H, d, J = 9.1 Hz), 6.39 (2H, d, J = 9.1 Hz), 6.19 (2H, d, J = 1.9 Hz), 5.22 (1H, d, J = 7.6 Hz, glucosyl anomeric H), 4.13 (1H, d, J = 7.8 Hz, glucosyl anomeric H), 3.01–3.97 (sugar protons). 13C NMR (125 MHz, methanol-d4): δ (kaempferol) 159.0 (C-2), 135.6 (C-3), 179.4 (C-4), 163.5 (C-5), 100.0 (C-6), 165.9 (C-7), 94.9 (C-8), 158.6 (C-9), 105.8 (C-10), 122.7 (C-1′), 132.3 (C-2’, C-6′), 116.3 (C-3′, C-5′), 161.5 (C-4′); δ (glucose x2)104.5, 104.1 (C-1), 75.4, 75.1 (C-2), 77.9, 77.8 (C-3), 72.4, 71.7 (C-4), 77.7, 77.6 (C-5), 69.6, 64.7 (C-6).
Statistical analysis
Data are presented as mean and SEM values from a minimum of four replicates and all experiments were repeated at least four times. Where appropriate, the significance of difference between two means was analyzed by using unpaired t-test.
Results and discussion
The anti-α-glucosidase activity of the methanol extract of C. alata was tested by using our established in vitro assay model (CitationHabtemariam, 2011). As shown in , potent concentration-dependent effect was demonstrated for the crude extract. IC50 values obtained from over four separate experiments are also shown in . It is apparent from these data that the extract was more active than the standard antidiabetic drug, acarbose (). In order to identify the active principles, a bioassay-guided fraction of the crude extract was further undertaken. Five fractions obtained by using solvents of ascending polarity (namely, petroleum ether, chloroform, ethyl acetate, n-butanol and water) were tested for their inhibitory property against α-glucosidase enzyme. As shown in , the most active fraction was ethyl acetate followed by the n-butanol fraction. The chloroform fraction was equipotent with the crude extract () while no activity was recorded for the petroleum ether and water fractions (). Further fractionation study was thus conducted on the ethyl acetate and n-butanol fractions which were 36- and 4-times, respectively, more active than the crude extract ().
Table 1. Anti-α-glucosidase activity of C. auriculata extract, fractions and isolated compounds.
Figure 1. Anti-α-glucosidase activity of the crude ethanol extract of C. alata leaves and its fractions. Data from a representative result shows mean and SEM values (n = 4).
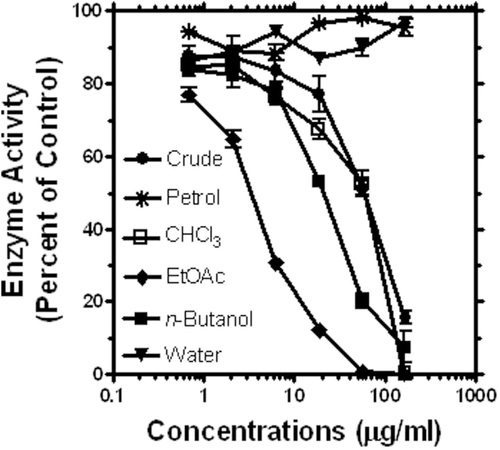
HPLC chromatograms showing constituents of the two most active fractions are shown in . The ethyl acetate extract appears to contain predominantly one compound which was eluted at a retention time (Rt) of 36.1 min while the predominant compound in the n-butanol fraction has a Rt of 27.2 min. The major component of the ethyl acetate fraction was isolated through a combination of gel filtration and silica gel-based chromatography system while that from the n-butanol fraction was isolated by using Combiflash ISCO chromatography system. Through the use of spectroscopic analysis including UV, IR, MS and NMR (1H, 13C, COSY, HSQC, HMBC and NOESY) studies, the two compounds from the ethyl acetate and n-butanol fraction were identified as kaempferol and kaempferol 3-O-gentiobioside, respectively (). The assignement of kaempferol was straight forward and was done by comparison with authentic sample obtained from Cassia species (CitationJuan-Badaturuge et al., 2011). The ESI MS of the major component of the n-butanol fraction gave the molecular ion mass [M+H]+ of 611.1619. The calculated mass at 611.1612 for this positive ion corresponds to a molecular formula of C27H31O16. The molecular ion was also labile for further MS-MS breakdown and gave a peak at 449.1033 (calc 449.1084; C21H21O11) which was consistent with a loss of one glucose mass unit from the molecular ion. All the other spectroscopic data were in agreement with those published before for kaempferol 3-O-gentiobioside (CitationIwashina et al., 2000).
Figure 2. HPLC chromatograms of active fractions obtained from the crude ethanol extract of C. alata leaves. Note that one major component of the ethyl acetate (panel A) and n-butanol (panel B) fraction.
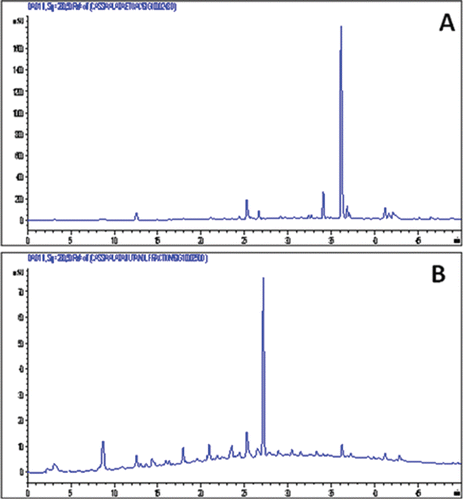
Figure 3. Structures of major components of the active fractions: kaempferol from the ethyl acetate and kaempferol 3-O-gentiobioside from the n-butanol fraction.
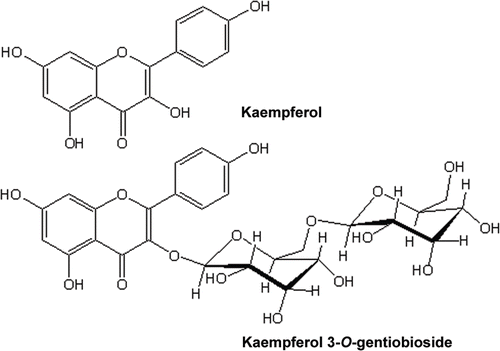
The major secondary metabolites of C. alata are reported to be flavonoids including kaempferol and its glycosides (e.g., kaempferol-3-O-gentiobioside and kaempferol-3-O-β-d-glucopyranoside), anthraquinones derivatives (e.g., alatonal, alatinone, chrysaphanol, emodin, rhein, aloe-emodin), essential oils, fatty acids and terpenoids (e.g., palmitic, oleic, linoleic acids, terpenoids (β-sitosterol, stigmasterol, campesterol) and many others including ellagitannins and p-hydroxybenzoic acid (CitationHennebelle et al., 2009 and references therein). Despite these large numbers of chemical components identified from C. alata, however, the active principles responsible for the antidiabetic properties of the plant were not known. Herewith, our study demonstrated that kaempferol and its major glycoside kaempferol-3-O-gentiobioside from the ethyl acetate and n-butanol fractions respectively displayed moderate anti-α-glucose-inhibitory activity. It is worth noting that the ethyl acetate fraction was more active than these isolated major constituents of the two fractions (). This could be due to either the presence of a potent minor components or synergistic mechanism of action of the ethyl acetate constituents. The exact mechanism of this discrepancy remains to be established but our most recent study demonstrated that many flavonoids including kaempferol inhibit α-glucosidase enzyme through synergistic mechanism (CitationHabtemariam, 2011).
In addition to moderate antioxidant effect (CitationJuan-Badaturuge et al., 2011), kaempferol displays a range of pharmacological activities including in vitro anticancer (CitationLuo et al., 2011) and antiangiogenic (CitationLuo et al., 2012) effects. Our previous studies on α-glucosidase activity of flavonoids also demonstrated that the 3-O-rutinoside of kaempferol displays potent enzyme inhibitory effect while that of quercetin derivative (rutin) was inactive (CitationHabtemariam, 2011). Here, we reported for the first time that the other kaempferol derivative, kaempferol 3-O-gentiobioside does also possess activity against α-glucosidase. Apart from C. alata (CitationMoriyama et al., 2003), the rare flavonoid, kaempferol 3-O-gentiobioside was isolated from few other plants such as Planchonia careya (F. Muell) R. Knuth (CitationMcRae et al., 2008) and Asplenium foreziense Legrand ex Hérib (CitationIwashina et al., 2000).
In conclusion, our study using a major carbohydrate digestion enzyme, α-glucosidase, revealed that Cassia alata displays antidiabetic potential. The reputed use of the plant could therefore be partly attributed due to this mechanism of action. A bioassay-guided fractionation study further revealed kaempferol and its glycoside derivative, kaempferol-gentiobioside were major components of the active fraction with moderate α-glucosidase activity. Further studies are required to demonstrate the in vivo activity of these isolated compounds.
Acknowledgments
The authors wish to thank the technical support of staff at the School of Science, University of Greenwich and Department of Botany, C.M.S. College, Kottayam, Kerala, India.
Declaration of interest
This work was supported by the University of Greenwich internal funding streams. The authors declared that there is no conflict of interest.
References
- Abo KA, Fred-Jaiyesimi AA, Jaiyesimi AE. (2008). Ethnobotanical studies of medicinal plants used in the management of diabetes mellitus in South Western Nigeria. J Ethnopharmacol, 115, 67–71.
- American Diabetes Association (2012). Diabetes Statistics. Available at: http://www.diabetes.org/diabetes-basics/diabetes-statistics/. Accessed on 20 June 2012.
- de Melo EB, Gomes AS, Carvalho I. (2006). α- and β-Glucosidase inhibitors: Chemical structure and biological activity. Tetrahedron, 62, 10277–10302.
- Donner TW. (2006). Tight control of hyperglycemia in type 2 diabetes mellitus. Insulin 1, 166–172.
- Girón LM, Freire V, Alonzo A, Cáceres A. (1991). Ethnobotanical survey of the medicinal flora used by the Caribs of Guatemala. J Ethnopharmacol, 34, 173–187.
- Habtemariam S. (2011). A-glucosidase inhibitory activity of kaempferol-3-O-rutinoside. Nat Prod Commun, 6, 201–203.
- Hennebelle T, Weniger B, Joseph H, Sahpaz S, Bailleul F. (2009). Senna alata. Fitoterapia, 80, 385–393.
- Iwashina T, López-Sáez JA, Herrero A, Kitajima J, Matsumoto S. (2000). Flavonol glycosides from Asplenium foreziense and its five related taxa and A. incisum. Biochem Syst Ecol, 28, 665–671.
- Juan-Badaturuge M, Habtemariam S, Thomas MJK. (2011). Antioxidant compounds from a South Asian beverage and medicinal plant, Cassia auriculata. Food Chem, 125, 221–225.
- Luo H, Rankin GO, Juliano N, Jiang BH, Chen YC. (2012). Kaempferol inhibits VEGF expression and in vitro angiogenesis through a novel ERK-NF?B-cMyc-p21 pathway. Food Chem, 130, 321–328.
- Luo H, Rankin GO, Li Z, Depriest L, Chen YC. (2011). Kaempferol induces apoptosis in ovarian cancer cells through activating p53 in the intrinsic pathway. Food Chem, 128, 513–519.
- Khan MH, Yadava PS. (2010). Antidiabetic plants used in Thoubal district Nanipur, Northeast India. Indian J Trad Knowl 9, 510–514.
- McRae JM, Yang Q, Crawford RJ, Palombo EA. (2008). Acylated flavonoid tetraglycoside from Planchonia careya leaves. Phytochemistry Lett, 1, 99–102.
- Moriyama H, Iizuka T, Nagai M, Murata Y. (2003). HPLC quantification of kaempferol-3-O-gentiobioside in Cassia alata. Fitoterapia, 74, 425–430.
- Palanichamy S, Nagarajan S, Devasagayam M. (1988). Effect of Cassia alata leaf extract on hyperglycemic rats. J Ethnopharmacol, 22, 81–90.
- Protabase Record display. Senna alata (L.). Available at: http://database.prota.org/. Accessed on 20 June 2012.
- Roselli M, Lentini G, Habtemariam S. (2012). Phytochemical, antioxidant and anti-α-glucosidase activity evaluations of Bergenia cordifolia. Phytother Res, 26, 908–914.
- USDA (2012)). Senna alata. Available at: http://plants.usda.gov. Accessed on 20 June 2012.
- Villaseñor IM, Canlas AP, Pascua MP, Sabando MN, Soliven LA. (2002). Bioactivity studies on Cassia alata Linn. leaf extracts. Phytother Res, 16 Suppl 1, S93–S96.
- WHO (World Health Organisation, Country Office for India). (2012). Atlas for diabetes mellitus in India. Available at: http://www.whoindia.org/en/Section20/Section385_1117.htm. Accessed on 20 June 2012.