Abstract
Context: Neuroprotective therapy to rescue dopaminergic neurons is an important trait in the management of Parkinson’s disease (PD).
Objective: The present study identified and evaluated SFSE-T, a standardized hydroalcoholic extract of Trigonella foenum-graecum L. seeds (Fabaceae), in animal models of PD.
Materials and methods: The identification of SFSE-T was carried out by high-performance liquid chromatography for the marker compound trigonelline (TGN). The effects of single dose oral treatment of SFSE-T (10, 30 or 100 mg/kg) were studied using animal models of PD, namely, 6-hydroxydopamine (6-OHDA)-induced unilateral lesions in rats, and 4-phenyl-1,2,3,6-tetrahydropyridine (MPTP)-induced neurodegeneration in C57BL/6 mice. The effects of SFSE-T on monoamino oxidase (MAO) enzyme in vitro as well as possible side effects of SFSE-T in vivo were also evaluated.
Results: The concentration of TGN in a test sample of SFSE-T was found to be 82%. SFSE-T (30 mg/kg, oral) showed a significant increase in the number of ipsilateral rotations (45.67 rotations in 30-min period) as compared with vehicle control group (no rotations) when tested in 6-OHDA-induced unilateral lesioned rats. SFSE-T (30 mg/kg, oral) showed significant reversal of motor dysfunction (spontaneous motor activity scores, speed, distance traveled and number of square crossed) caused by MPTP induced lesions in C57BL/6 mice in pretreatment (1 h) schedule but not in post-treatment (1 h) schedule. SFSE-T neither showed anticholinergic effects nor showed selective MAO-B enzyme inhibition in vitro.
Discussion and conclusion: SFSE-T showed reversal of motor symptoms in an animal model of PD probably through neuroprotective properties.
Introduction
Parkinson’s disease (PD) is a progressive, neurodegenerative disorder affecting mainly dopaminergic neuronal systems with impaired motor function. However, other symptoms such as neuropsychiatric problems, autonomic dysfunction and sleep disorders may occur.
Despite the great number of ongoing investigations, neurodegenerative diseases including PD remain incurable. Current therapy for PD is essentially symptomatic. Levo-dopa (L-dopa), the direct precursor of dopamine, remains the primary treatment choice since first use over 30 years ago (Markham & Diamond, Citation1981; Silva et al., Citation1997). L-Dopa can relieve the major clinical symptom of PD, i.e., the inability to initiate voluntary movements (Cotzias et al., Citation1969). However, the success of long-term L-dopa therapy in PD is compromised by the almost invariable intrusion of dyskinesias during the periods of the day when medication is most effective (Cotzias et al., Citation1969; Ruberg et al., Citation1995). Even after treatment with L-dopa, with or without other dopaminergic drugs for more than 5 or 6 years, a majority of patients develop response fluctuations, dyskinesia or dystonia (Papapetropoulos et al., Citation2007).
Although the pathogenesis of PD is still unclear, various studies have uncovered important clues to the cause of the dopaminergic cell death. Accumulating evidence has suggested that both environmental and genetic factors collaborate and cause dopaminergic cell death. Aging, exposure to pesticides and endogenous toxic agents, such as dopamine derivatives, might induce oxidative stress and lead to neurodegeneration (Takeuchi & Takahashi, Citation2007). Recent advances in PD genetics have revealed a prominent role of impaired mitochondrial function and subsequent increase in oxidative stress and other related processes, including excitotoxicity, in the pathophysiology of PD (Henchcliffe & Beal, Citation2008).
Neuroprotective therapy to rescue dopaminergic neurons has been proposed as an important trait in the management of PD (Meissner et al., Citation2011). Neuroprotective agents can alter and prevent the progression of PD (Dunnett & Bjorklund, Citation1999). However, protective effects in humans are confirmed only after long-term administration of the substance. Moreover, treatments that provide neuroprotection and/or disease-modifying effects remain an urgent unmet clinical need (Meissner et al., Citation2011). Because of their long experience of consumption and safety profiles, the dietary ingredients (active constituents from food chain material) can be helpful in preventing progression of diseases such as PD and is a new hope in the disease management.
Fenugreek [Trigonella foenum-graecum L. (Fabaceae)] is commonly used as a dietary ingredient (spice) in India, Middle East, Egypt, North Africa and other parts of the world. Fenugreek seeds have been used as a traditional remedy for numerous conditions including gastrointestinal disorders, gout, wound healing, inflammation, hyperlipidemia and diabetes (Petropoulos, Citation2002). Fenugreek seeds are reported to have potent antioxidant effects and protect many vital organs in the body (Anuradha & Ravikumar, Citation2001; Devasena & Menon, Citation2002, Citation2007; Kaviarasan et al., Citation2006). In fact, antioxidant potential is one of the major contributors of antihyperglycemic effects of fenugreek seeds in diabetic rats (Anuradha & Ravikumar, Citation2001; Preet et al., Citation2005).
Trigonelline (TGN) is a major alkaloid in fenugreek seeds (Mishkinsky et al., Citation1967; Nadkarni, Citation1990) and coffee beans (Hong et al., Citation2008) with potent antioxidant properties (Saravanan & Pari, Citation2005; Yen et al., Citation2005). TGN has been shown to induce neuronal regeneration (Tohda et al., Citation2005) in vitro, and is alleged to be responsible for protective effects against diabetes-induced auditory neuropathy (Hong et al., Citation2008). Therefore, extracts of Trigonella foenum-graecum seeds that are identified and quantified to bioactive marker compound, TGN can be a useful agent to prevent or repair neuronal damage and associated symptoms during PD progression.
The objectives of the present study were (1) to identify and quantify the marker compound, TGN, in the hydroalcoholic extract of Trigonella foenum-graecum seeds, SFSE-T; (2) to evaluate anti-Parkinson’s activity of SFSE-T using 6-hydroxydopamine (6-OHDA) and 4-phenyl-1,2,3,6-tetrahydropyridine (MPTP)-induced neurodegeneration in rodents; (3) to study effects of SFSE-T as monoamino oxidase (MAO) enzyme inhibitor in vitro using human recombinant insect Hi5 cells, and (4) to record the anticholinergic side effects in vivo using oxotremorine-induced salivation and lacrimation in mice.
Materials and methods
Animals
Swiss Albino mice (20–25 g) and Wistar rats (225–300 g) were purchased from the National Toxicology Centre, Pune, Maharashtra, India. C57BL/6 mice (20–25 g) were purchased from the National Institute of Nutrition, Hyderabad, Andhra Pradesh, India. The mice were housed at a temperature of 25 ± 1 °C and relative humidity of 45–55% under 12:12 light:dark cycle. The animals had free access to feed pellets (Amrut Laboratory Animal Feed, Nav Maharashtra Chakan Oil Mills Ltd, Sangli, Maharashtra, India) and tap water. The experimental protocol was approved by the Institutional Animal Ethics Committee of Poona College of Pharmacy, Pune, Maharashtra, India. All observations were made between 9:00 and 17:00 h in a quiet room, at 23–26 °C.
Drugs and chemicals
Imipramine HCl was obtained as a gift sample from Torrent Pharmaceuticals Ltd., Gujarat. 6-OHDA hydrobromide, MPTP, ketamine hydrochloride/xylazine hydrochloride solution and lidocaine were purchased from Sigma-Aldrich (St Louis, MO). A solution of 6-OHDA was prepared by dissolving in 2% ascorbic acid in saline and was administered through the intracerebroventricular route. MPTP solution was prepared by dissolving MPTP hydrochloride in 0.9% saline to a make final concentration of 200 mg/ml. MPTP solution (2 mg/ml) was prepared in saline and administered by the intraperitoneal (i.p.) route. Apomorphine HCl solution was prepared by dissolving in 0.1% ascorbic acid solution and diluted with 0.9% saline.
The test compound, SFSE-T, is a hydroalcoholic extract (water:ethyl alcohol in proportion of 30:70) of fenugreek seeds enriched by column chromatography for marker compound TGN hydrochloride (HCl) using a reported method (Shah et al., Citation2006); the material was provided by Indus Biotech Private Limited, Pune, Maharashtra, India. Briefly, fenugreek seeds were flaked, loaded in an extractor and water:alcohol (30:70) was circulated in the extractor at room temperature for 8 h. The extract was drained and distilled to remove alcohol. The marc was extracted with hexane to remove oil and other lipids. The oil-free water extract was passed through strong acid cation exchange resin in gel form where all the zwitterions were bound on the column (amino acids and TGN). The resin was thoroughly washed with de-ionized water to remove adhering sugars and other contaminants. The column was eluted with 10% aqueous ammonia in which all the amino acids and TGN was eluted and appeared in ammonia solution. The solution was concentrated to remove ammonia and water. The resulting powder contained about 30% TGN. The powder was dissolved in 20 volumes of isopropyl alcohol and treated with activated charcoal to remove coloring impurities. The filtered solution was then cooled to 0 °C and slowly 20% hydrochloride gas in isopropyl alcohol was added in stoichometric quantity and stirred for 6 h at 0 °C where TGN was precipitated as TGN HCl. SFSE-T was dissolved in demineralized water and administered orally (10 mL/kg of body weight for mice and 1 mL/kg for rats) for pharmacological studies.
Identification of SFSE-T based on TGN by high-performance liquid chromatography
The identification of SFSE-T was done for TGN HCl as marker compound. The purity of SFSE-T was confirmed by comparison of high-performance liquid chromatography (HPLC) of sample with that of reference standard of TGN HCl (Sigma-Aldrich, ≥98.0%). Sample and reference standard solution was prepared with 10 mg in 100 ml of demineralized water. The HPLC was recorded with the following conditions: model – JASCO LC 2000 with UV-2075; column: reverse phase C-18 column L1 (250 mm × 4.6 mm) with 5 µ particle size; detector: UV–Vis, mobile phase: trifluroacetic acid (60) with water:acetonitrile mixture (40), injection volume: 20 µL, method time: 30 min, flow rate: 1.5 mL/min; Detector: UV (at 254 nm). The area under the curve (AUC) was recorded from the resultant chromatogram (at 254 nm) and content of marker compound (TGN HCl) was calculated by the following formulae: [(AUC in HPCL of sample × weight of the reference standard × sample dilution × purity of reference standard)/(AUC in HPCL of reference standard × dilution of reference standard × weight of the sample)].
Effect of SFSE-T on rotational behavior in rats with unilateral 6-OHDA lesions
Male Wistar rats (225–275 g) were pretreated with imipramine (25 mg/kg, i.p.), 1 h before surgery, for restricting possible interference in response by noradrenergic neuronal damage by 6-OHDA (McCall et al., Citation2005; Ungerstedt, Citation1971b). Rats were anesthetized with ketamine hydrochloride/xylazine hydrochloride solution given at 3 mL/kg, i.p. and placed in a stereotaxic apparatus with the incisor bar raised to 5 mm. A small hole was drilled through the skull. Through the hole, 30-gauge stainless tubing was lowered to the right substantia nigra using the following coordinates for rats (AP, −1.5 mm; L, +1.8 mm and V, −8.0 mm; for larger rats, AP, −2.8 mm; L, +2.0 mm and V, −8.0 mm) with reference to bregma (Pellegrino et al., Citation1979). 6-OHDA hydrobromide solution was injected into the substantia nigra at 12 µg/2 µL of free base in 0.9% saline/0.1% ascorbic acid at 1 µL/min, using a syringe pump (Hamilton Co., Reno, NV). After surgery, the scalp incision was closed with stainless steel wound clips, and neomycin ointment containing lidocaine was applied to the incision area. Rats were housed in individual cages.
Two weeks after surgery, clips were removed and rats were divided into six groups. They were treated as follows: (1) vehicle control, (2) SFSE-T (10 mg/kg, oral), (3) SFSE-T (30 mg/kg, oral), (4) SFSE-T (100 mg/kg, oral), (5) apomorphine (0.3 mg/kg, s.c.) and (6) L-dopa (10 mg/kg, oral). One hour after treatment, apomorphine HCl (0.3 mg/kg, s.c.) dissolved with 0.1% ascorbic acid in 0.9% saline) was administered to rats. The effects of the lesions were tested by monitoring the turning rate of rats at 5-min intervals for next 30 min in cages with floor covered with paper. The cage floor paper was changed for each animal.
Effect of SFSE-T on MPTP lesioned C57BL/6 mice
Male C57BL/6 mice (20–25 g) were used for the experiment. Mice were divided into groups consisting of six mice each. Each mouse received a total of four MPTP injections (0.4 ml each) by the i.p. route after a 2 h interval from 10:00 am to 4:00 pm. The concentration of MPTP solution was 200 mg/mL and the total dose of MPTP administration per mouse was 20 mg (Finnegan et al., Citation1987; Ricaurte et al., Citation1987).
The effects of SFSE-T were observed by two schedules. In the pre-treatment schedule, SFSE-T (30 mg/kg, oral) was administered 1 h before MPTP administration. In the post-treatment schedule, SFSE-T (30 mg/kg, oral) was administered 1 h after the last injection of MPTP (Jackson-Lewis et al., Citation1995). A separate control group (receiving neither MPTP nor SFSE-T) and a MPTP control group were also maintained. The mice were tested for the spontaneous motor activity (SMA) score in actophotometer (Inco, Ambala, Haryana, India). The mice were then placed individually in an open field (35 cm × 30 cm × 22 cm) with a black rubber floor. The mice were placed individually in the open field and allowed to habituate to the environment for 10 min. Subsequently, spontaneous movements were recorded automatically in the next 10 min. The parameters recorded were total number of squares crossed, total distance traveled, total movement time, and average speed in open field in 5 min. The mean reading of each parameter was calculated and compared.
In vitro MAO-A and MAO-B enzyme inhibition activity of SFSE-T
The enzyme inhibition activity of SFSE-T against MAO-A and MAO-B enzymes was performed using human recombinant insect Hi5 cells (Urban et al., Citation1991; Youdim & Finberg, Citation1991). The assay was performed using the following conditions: substrate (Kynuramine, 50 µM), vehicle (1% DMSO), pre-Incubation time (15 min), pre-incubation temperature (37 °C), incubation time (60 min), incubation temperature (37 °C), incubation buffer (100 mM potassium phosphate, pH 7.4), quantitation method (spectrofluorimetric quantitation of 4-hydroxyquinoline), clorgyline, and R(-)-deprenyl (selegiline) were selected as reference compounds for selective MAO-A and MAO-B enzyme inhibitor activity measurement, respectively.
Effects of SFSE-T on oxotremorine induced salivation and lacrimation
The muscarinic agonist, oxotremorine, induces Parkinsonism-like signs such as tremor, ataxia, spasticity, salivation and lacrimation. The anticholinergic potential of SFSE-T was evaluated by assessing effects on oxotremorine induced salivation and lacrimation (Duvoisin, Citation1976). The Swiss albino mice (20–25 g) were divided into three groups of six mice each. The groups were treated with either with vehicle (saline, oral), SFSE-T (30 mg/kg, oral) or atropine (1 mg/kg, s.c.). Thirty min after treatment, oxotremorine (0.5 mg/kg, s.c.) was administrated to all mice. Fifteen min after oxotremorine treatment, mice were observed and scored for salivation and lacrimation are scored as follows: absent (0), slight (1), medium (2), severe (3) and death (4). The mean score for lacrimation and salivation were calculated and analyzed.
Statistical analysis
The data from 6-OHDA induced rotational behavior was analyzed by two-way analysis of variance (ANOVA) followed by Bonferroni post-tests. The data of MPTP induced motor parameters were analyzed by one-way ANOVA followed by Dunnett’s multiple comparison test. The data of oxotremorine induced effects was analyzed by nonparametric Kruskal–Wallis ANOVA followed by Dunn’s test for salivation and lacrimation separately. The significance level of p < 0.05 was considered significant in all the tests.
Results
Identification of SFSE-T based on TGN by HPLC
At a wavelength of 254 nm, the HPLC of the sample of SFSE-T showed the presence of the marker compound, TGN HCl, at a retention time of 1.750 (). The reference standard of TGN HCl showed a peak at retention time 1.775 min (). The concentration of TGN HCl in the SFSE-T sample was found to be 82% w/w.
Effect of SFSE-T on rotational behavior in rats with unilateral 6-OHDA lesions
Induction of unilateral lesions by administration of 6-OHDA resulted into rotational behavior in rats. Mean rotations in vehicle treated rats were 1.00 ± 0.37 (ipsilateral) and 0.33 ± 0.21 (contralateral). Apomorphine (0.3 mg/kg) and L-dopa (10 mg/kg) treatment caused a significant (p < 0.001) increase (169.33 and 45.67, respectively) in contralateral rotations () but did not significantly change the number of ipsilateral rotations. On the other hand, SFSE-T at 30 mg/kg, but not at 10 or 100 mg/kg, caused a significant increase in ipsilateral (p < 0.001) rotations (30.67). However, SFSE-T did not show any contralateral rotations ().
Table 1. Effect of SFSE-T (10, 30 or 100 mg/kg) on number of rotations after 6-OHDA-induced unilateral lesions in rats.
Effect of SFSE-T on MPTP lesioned C57BL/6 mice
When tested in an actophotometer, administration of MPTP to C57BL/6 mice significantly decreased SMA as compared to the control group (). SFSE-T pretreatment significantly reversed MPTP-induced decrease. However, post-MPTP treatment of SFSE-T did not show change in SMA (). In the open field test, MPTP lesioned mice showed significant (p < 0.001) reduction in average speed (), total distance traveled (), and number of squares crossed () by mice. Pretreatment of SFSE-T (30 mg/kg) caused significant (p < 0.001) reversal of MPTP-induced locomotor changes in open field behavior (). However, MPTP-induced locomotion in open field did not change with post treatment of SFSE-T ().
Figure 2. Effect of SFSE-T at 10, 30 or 100 mg/kg on MPTP lesions in the C57BL/6 mouse model. The parameters assessed are (A) SMA in actophotometer, (B) average speed, (C) total distance traveled, and (D) number of squares crossed by mice in open field. n = 6, data were analyzed by one-way ANOVA followed by Dunnett’s Multiple Comparison Test. ###p < 0.001 as compared with vehicle control, ***p < 0.001 as compared with MPTP.
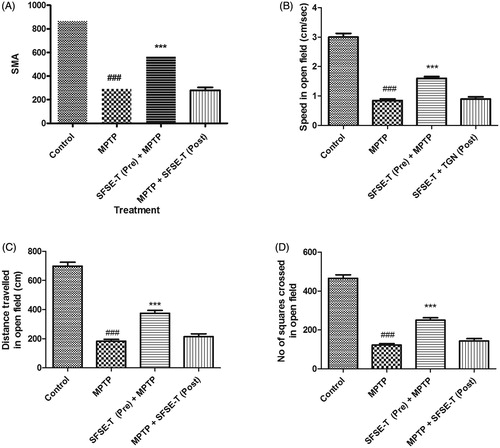
In vitro MAO-A and MAO-B enzyme inhibition activity of SFSE-T
The concentration response curve was plotted and calculation of the inhibitory concentration of 50% enzyme activity (IC50) was calculated. IC50 of SFSE-T against MAO-A was 528 µg/mL (2.81 µM) whereas the IC50 against MAO-B was found to be 391 µg/mL (2.1 µM). The reference compounds, clorgyline and R(-)-deprenyl, showed 0.365 nM and 6 nM activity against MAO-A and MAO-B, respectively. These results suggest that SFSE-T is not a selective inhibitor of MAO-B enzyme as compared with MAO-A enzyme.
Effects of SFSE-T on oxotremorine-induced salivation and lacrimation
SFSE-T did not cause any change in oxotremorine-induced salivation () or lacrimation () in rats. Atropine (1 mg/kg) caused a significant (p < 0.01) decrease in oxotremorine-induced salivation and lacrimation ()
Discussion
Neuroprotective therapy to rescue dopaminergic neurons, which could alter and prevent the progression of PD (Dunnett & Bjorklund, Citation1999), has been suggested as an alternative to symptomatic treatment in PD management. However, existing dopaminergic drugs do not prevent or reverse the underlying neurodegeneration.
TGN is an alkaloid found in fenugreek seeds (Mishkinsky et al., Citation1967; Nadkarni, Citation1990) and coffee beans (Hong et al., Citation2008). TGN showed neuroprotective potential in the past (Hong et al., Citation2008; Tohda et al., Citation1999) due to antioxidant properties (Saravanan & Pari, Citation2005; Yen et al., Citation2005). In the present study, we are reporting a novel method of preparation of SESE-T with TGN as a marker compound. The test extract (SFSE-T) contains 82% of marker compound, TGN.
SFSE-T (30 mg/kg, oral) showed significant ipsilateral rotations against 6-OHDA-induced unilateral lesions. Unilateral lesion of the dopaminergic nigrostriatal pathway in the rat by the neurotoxin 6-OHDA induces hypersensitivity of the postsynaptic dopaminergic receptors in the striatum of the lesioned side. The 6-OHDA model involves injection of the toxin directly in the striatum or in median forebrain bundle to cause retrograde degeneration of substantia nigra neurons. 6-OHDA lesions causes slow and partial lesion of these neurons over 2 weeks and mimic the slow progression of PD (Beal, Citation2001; Deumens et al., Citation2002). Noradrenergic neurons are required to transport the 6-OHDA inside the brain and after further traversal into the substantia nigra, it selectively accumulates in, and is toxic to, dopaminergic neurons probably by generation of free radicals. In our study, imipramine pretreatment avoided the interference of NA in neuronal damage by 6-OHDA.
In the present investigation, contralateral rotational response shown by apomorphine and L-dopa-treated rats are in agreement with an earlier report (Ungerstedt, Citation1971a). The rats rotate in a direction towards the lesioned side (ipsilateral) when an indirect acting compound such as amphetamine is administered but to the opposite direction (contralateral) when a direct acting dopamine agonist, e.g., apomorphine, or the dopamine precursor L-dopa is given (Ungerstedt, Citation1971a). These results ensures complete damage of DA neurons by 6-OHDA as reported earlier (Annett et al., Citation1992; Paxinos & Watson, Citation2007). In our study, SFSE-T treatment showed a significant rise in the number of ipsilateral rotations at doses of 10 and 30 mg/kg, but not at a higher dose (100 mg/kg), indicating dopamine releasing action. The results suggest lack of dopamine agonist activity for SFSE-T. TGN is a known carrier for dopamine to the brain (Anonymous, Citation1983) and may be responsible for facilitating endogenous dopamine release in the substantial nigra to improve symptoms. The 6-OHDA model mimics slow progressive changes (involves partial loss of dopaminergic neurons) and so the present results suggest efficacy of SFSE-T in slowing the progression of PD.
In the present study, SFSE-T showed reversal of MPTP-induced motor dysfunction in C57BL/6 mice. MPTP acts as neurotoxin, which preferentially affected dopaminergic cells in substantia nigra pars compacta. MPTP-induced lesions in C57BL/6 mice cause transient impairment of dopamine system function in mice. MPTP-induced neuronal impairment is caused by conversion of MPTP to 1-ethyl-4-phenyl-2, 3-dihydropyridium ion (MPP+) in astrocytes by MAO-B (Nicklas et al., Citation1985). MPP+ is selectively toxic to dopaminergic neurons because of high affinity for the dopamine transporter (DAT), at least in part (Javitch & Snyder, Citation1984). MPTP currently represents the most important and most frequently used Parkinsonian toxin applied in animal models and has several advantages over other neurotoxic PD models (Beal, Citation2001; Schober, Citation2004). In our study, all the motor parameter changes induced by MPTP are reversed by pre-treatment but not by post-treatment schedule of SFSE-T. SFSE-T perhaps activated DAT activating electron transport system of mitochondrial complex-I (NADPH-ubiquinone oxidoreductase I) and might prevent MPTP-induced neuronal damage.
In the brains of patients suffering from other neurodegenerative diseases, such as PD, neurite atrophy has been observed (Mattila et al., Citation1999). Such atrophy leads to the destruction of neuronal networks, and subsequently to the fatal dysfunction of brain systems. TGN has been shown to cause neurite outgrowth with human neuroblastoma SK–N–SH cells (Mattila et al., Citation1999) and induce neuronal regeneration (Tohda et al., Citation2005) in vitro. TGN-induced neurite outgrowth might have enhanced functional connectivity of neurons and helped to preserve synaptic connectivity in MPTP-induced mice in our study. TGN was also reported to have ability to prevent exclusion of, neurons against atrophy induced by Aß(25--35) in vitro (Mattila et al., Citation1999). Therefore, TGN has potential to prevent the progression of PD by exclusion of, or at least a decrease in the magnitude of, neuronal atrophy. The ability of TGN to induce neurite outgrowth and regeneration indicated the potential to protect vital organs against oxidative stress (Mattila et al., Citation1999; Tohda et al., Citation2005). TGN is a potent antioxidant (Saravanan & Pari, Citation2005; Yen et al., Citation2005) and functions as a signal transmitter in response to oxidative stress in plants (Berglund, Citation1994). Further, TGN is a major metabolite of nicotinic acid and is excreted unchanged in urine (Yuyama & Suzuki, Citation1985) and thus available for protective action on vital organs including neurons. Therefore, neuroprotective potential of SFSE-T shown in the present study can be attributed to TGN, a marker compound of SFSE-T.
In the past, some marketed MAO-B inhibitors, like selegiline or rasagiline, showed a limited amount of neuroprotection in animal models of PD (Tuite & Riss, Citation2003). Selective MAO-B inhibitors have been shown to provide relief from motor symptoms in some PD patients (Nayak & Henchcliffe, Citation2008). Therefore, SFSE-T was evaluated for MAO enzyme inhibition potential in vitro. However, SFSE-T did not show specificity towards MAO-B enzyme as compared with MAO-A enzyme in the present study.
Anti-cholinergic side effects at the therapeutic dose are the major problem for long-term management of PD. In the present study, SFSE-T did not show anticholinergic potential against oxotremorine (selective agonist of muscarinic cholinergic receptors) at an effective dose (30 mg/kg).
It is premature to propose the mechanism(s) involved in the observed anti-Parkinsonian effects of SFSE-T. We do not suggest any single mechanism for the observed neuroprotective effects of SFSE-T in animal models of PD. However, on the basis of the present findings and background literature on SFSE-T and TGN, neuroprotective action shown by SFSE-T can be attributed to antioxidant, free radical-scavenging, or DA enhancing properties. However, we consider the findings very encouraging with good anti-Parkinsonian potential and worthy of further investigation using various molecular, pharmacological and genetic approaches.
Conclusions
In conclusion, SFSE-T showed promising neuroprotective effects in laboratory animal models of PD without anticholinergic effects. However, further studies on SFSE-T are required to explore its potential mechanism and role towards long-term management of PD.
Declaration of interest
The authors report no declaration of interest. The authors alone are responsible for the content and writing of the paper.
Acknowledgements
The authors would like acknowledge Dr. S. S. Kadam, Vice-Chancellor and Dr. K. R. Mahadik, Principal, Poona College of Pharmacy, Bharati Vidyapeeth Deemed University, Pune, India and Sunil Bhaskaran, MD, Indus Biotech Private Limited, Pune, India for providing necessary support to carry out the study.
References
- Annett LE, Rogers DC, Hernandez TD, Dunnett SB. (1992). Behavioural analysis of unilateral monoamine depletion in the marmoset. Brain 115:825–56
- Anonymous. (1983). New hope for Parkinsonism. Chemical Week 27 April 1983, 331
- Anuradha CV, Ravikumar P. (2001). Restoration on tissue antioxidants by fenugreek seeds (Trigonella foenum graecum) in alloxan-diabetic rats. Indian J Physiol Pharmacol 45:408–20
- Beal MF. (2001). Experimental models of Parkinson’s disease. Nat Rev Neurosci 2:325–534
- Berglund T. (1994). Nicotinamide, a missing link in the early stress response in eukaryotic cells: A hypothesis with special reference to oxidative stress in plants. FEBS Lett 351:145–9
- Cotzias GC, Papavasiliou PS, Gellene R. (1969). Modification of Parkinsonism – chronic treatment with L-dopa. N Engl J Med 280:337–45
- Deumens R, Blokland A, Prickaerts J. (2002). Modeling Parkinson’s disease in rats: An evaluation of 6-OHDA lesions of the nigrostriatal pathway. Exp Neurol 175:303–17
- Devasena T, Menon VP. (2002). Enhancement of circulatory antioxidants by fenugreek during 1,2-dimethylhydrazine-induced rat colon carcinogenesis. J Biochem Mol Biol Biophys 6:289–92
- Devasena T, Menon VP. (2007). Fenugreek seeds modulate 1,2-dimethylhydrazine-induced hepatic oxidative stress during colon carcinogenesis. Ital J Biochem 56:28–34
- Dunnett SB, Bjorklund A. (1999). Prospects for new restorative and neuroprotective treatments in Parkinson’s disease. Nature 399:A32–9
- Duvoisin RC. (1976). Parkinsonism: animal analogues of the human disorder. Res Publ Assoc Res Nerv Ment Dis 55:293–303
- Finnegan KT, Irwin I, Delanney LE, et al. (1987). 1,2,3,6-Tetrahydro-1-methyl-4-(methylpyrrol-2-yl)pyridine: studies on the mechanism of action of 1-methyl-4-phenyl-1,2,3,6-tetrahydropyridine. J Pharmacol Exp Ther 242:1144–51
- Henchcliffe C, Beal MF. (2008). Mitochondrial biology and oxidative stress in Parkinson disease pathogenesis. Nat Clin Pract Neuro 4:600–9
- Hong BN, Yi TH, Park R, et al. (2008). Coffee improves auditory neuropathy in diabetic mice. Neurosci Lett 441:302–6
- Jackson-Lewis V, Jakowec M, Burke RE, Przedborski S. (1995). Time course and morphology of dopaminergic neuronal death caused by the neurotoxin 1-methyl-4-phenyl-1,2,3,6-tetrahydropyridine. Neurodegeneration 4:257–69
- Javitch JA, Snyder SH. (1984). Uptake of MPP(+) by dopamine neurons explains selectivity of Parkinsonism-inducing neurotoxin, MPTP. Eur J Pharmacol 106:455–6
- Kaviarasan S, Ramamurty N, Gunasekaran P, et al. (2006). Fenugreek (Trigonella foenum graecum) seed extract prevents ethanol-induced toxicity and apoptosis in Chang liver cells. Alcohol Alcohol 41:267–73
- Markham CH, Diamond SG. (1981). Evidence to support early levodopa therapy in Parkinson disease. Neurology 31:125–31
- Mattila PM, Rinne JO, Helenius H, Roytta M. (1999). Neuritic degeneration in the hippocampus and amygdala in Parkinson’s disease in relation to Alzheimer pathology. Acta Neuropathol (Berl) 98:157–64
- McCall RB, Lookingland KJ, Bedard PJ, Huff RM. (2005). Sumanirole, a highly dopamine D2-selective receptor agonist: In vitro and in vivo pharmacological characterization and efficacy in animal models of Parkinson’s disease. J Pharmacol Exp Ther 314:1248–56
- Meissner WG, Frasier M, Gasser T, et al. (2011). Priorities in Parkinson’s disease research. Nat Rev Drug Discov 10:377–93
- Mishkinsky J, Joseph B, Sulman FG. (1967). Hypoglycaemic effect of trigonelline. Lancet 2:1311–12
- Nadkarni AK. (1990). The Indian Materia Medica. Bombay, India: Popular Prakashan Pvt Ltd
- Nayak L, Henchcliffe C. (2008). Rasagiline in treatment of Parkinson’s disease. Neuropsychiatr Dis Treat 4:23–32
- Nicklas WJ, Vyas I, Heikkila RE. (1985). Inhibition of NADH-linked oxidation in brain mitochondria by 1-methyl-4-phenyl-pyridine, a metabolite of the neurotoxin, 1-methyl-4-phenyl-1,2,5,6-tetrahydropyridine. Life Sci 36:2503–8
- Papapetropoulos S, Basel M, Mash DC. (2007). Dopaminergic innervation of the human striatum in Parkinson’s disease. Mov Disord 22:286–8
- Paxinos G, Watson C. (2007). The Rat Brain in Stereotaxic Coordinates. 6th ed. Amsterdam, The Netherlands: Elsevier
- Pellegrino LJ, Pellegrino AS, Cushman AJ. (1979). A Stereotaxic Atlas of the Rat Brain. 2nd ed. New York, NY: Plenum Press
- Petropoulos GA. (2002). Fenugreek: The Genus Trigonella. New York, NY: Taylor and Francis
- Preet A, Gupta BL, Siddiqui MR, et al. (2005). Restoration of ultrastructural and biochemical changes in alloxan-induced diabetic rat sciatic nerve on treatment with Na3VO4 and Trigonella – a promising antidiabetic agent. Mol Cell Biochem 278:21–31
- Ricaurte GA, Irwin I, Forno LS, et al. (1987). Aging and 1-methyl-4-phenyl-1,2,3,6-tetrahydropyridine-induced degeneration of dopaminergic neurons in the substantia nigra. Brain Res 403:43–51
- Ruberg M, Scherman D, Javoy-Agid F, Agid Y. (1995). Dopamine denervation, age of onset, and Parkinson’s disease. Neurology 45:392
- Saravanan R, Pari L. (2005). Antihyperlipidemic and antiperoxidative effect of diasulin, a polyherbal formulation in alloxan induced hyperglycemic rats. BMC Complement Altern Med 5:1–8
- Schober A. (2004). Classic toxin-induced animal models of Parkinson’s disease: 6-OHDA and MPTP. Cell Tissue Res 318:215–24
- Shah S, Bodhankar SL, Badole SL, et al. (2006). Effect of trigonelline: An active compound from Trigonella foenum graecum Linn. in alloxan induced diabetes in mice. J Cell Tissue Res 6:585–90
- Silva MA, Mattern C, Hacker R, et al. (1997). Increased neostriatal dopamine activity after intraperitoneal or intranasal administration of L-DOPA: On the role of benserazide pretreatment. Synapse 27:294–302
- Takeuchi H, Takahashi R. (2007). Pathogenesis of Parkinson disease. Nippon Ronen Igakkai Zasshi 44:415–21
- Tohda C, Kuboyama T, Komatsu K. (2005). Search for natural products related to regeneration of the neuronal network. NeuroSignals 14:34–45
- Tohda C, Nakamura N, Komatsu K, Hattori M. (1999). Trigonelline-induced neurite outgrowth in human neuroblastoma SK-N-SH cells. Biol Pharm Bull 22:679–82
- Tuite P, Riss J. (2003). Recent developments in the pharmacological treatment of Parkinson’s disease. Expert Opin Investig Drugs 12:1335–52
- Ungerstedt U. (1971a). Adipsia and aphagia after 6-hydroxydopamine induced degeneration of the nigro-striatal dopamine system. Acta Physiol Scand Suppl 367:95–122
- Ungerstedt U. (1971b). Striatal dopamine release after amphetamine or nerve degeneration revealed by rotational behaviour. Acta Physiol Scand Suppl 367:49–68
- Urban P, Andersen JK, Hsu HP, Pompon D. (1991). Comparative membrane locations and activities of human monoamine oxidases expressed in yeast. FEBS Lett 286:142–6
- Yen WJ, Wang BS, Chang LW, Duh PD. (2005). Antioxidant properties of roasted coffee residues. J Agric Food Chem 53:2658–63
- Youdim MB, Finberg JP. (1991). New directions in monoamine oxidase A and B selective inhibitors and substrates. Biochem Pharmacol 41:155–62
- Yuyama S, Suzuki T. (1985). Isolation and identification of N1-methylnicotinic acid (trigonelline) from rat urine. J Nutr Sci Vitaminol (Tokyo) 31:157–67