Abstract
Context: Phytochemicals are produced by desert plants to protect themselves against stressful environments. They have been shown to be useful in preventing and fighting adverse pathophysiological conditions and complex diseases, including cancer. Although many desert plants have been investigated for their antitumor properties, a large number of them still remain to be explored for possible therapeutic applications in oncologic diseases.
Objective: To screen the antitumor effects of selected desert plants, namely Achillea fragrantissima (Forssk.) Sch. Bip. (Compositae), Ochradenus baccatus Delile (Resedaceae), Origanum dayi Post (Lamiaceae), Phlomis platystegia Post (Lamiaceae) and Varthemia iphionoides Boiss (Compositae), against an in vitro tumor model utilizing HepG2 human hepatocellular carcinoma cells.
Materials and methods: The aqueous extracts of aerial parts of the aforementioned plants were prepared and used for the in vitro experiments. The HepG2 cells were exposed to varying concentrations (0–4 mg/mL) of each plant extract for 24 or 48 h and the cytotoxicity was measured by the MTT assay.
Results: Following 24 h exposure, O. dayi extract exhibited a substantial antiproliferative effect in HepG2 cells (IC50 = 1.0 mg/mL) followed by O. baccatus (IC50 = 1.5 mg/mL). All plant extracts displayed cytotoxicity following 48 h exposure. Nevertheless, a substantial effect was observed with O. dayi (IC50 = 0.35 mg/mL) or O. baccatus (IC50 = 0.83 mg/mL).
Conclusion: The aqueous extracts from aerial parts of O. dayi and O. baccatus possess antitumor effects against human liver cancer cells. These desert plants represent valuable resources for the development of potential anticancer agents.
Introduction
Cancer represents one of the leading causes of death in both economically developed as well as developing countries (WHO, Citation2008). Globally, approximately 12.7 million cancer cases and 7.6 million cancer-related deaths are estimated to have occurred in 2008 (Jemal et al., Citation2011). More than 1.6 million new cases and about 577 190 deaths from cancer are projected to occur in the United States in 2012 (Siegel et al., Citation2012). By 2030, approximately 26 million new cancer cases and 17 million cancer deaths per year are expected worldwide (Boyle & Levin, 2008). The growth and aging of populations coupled with adoption of lifestyle with a multitude of risk factors, including smoking, physical inactivity and the “western diet”, contribute to the increasing burden of cancer (Jemal et al., Citation2011).
Despite tremendous advancements in medicine, there are still no effective drugs to cure most cancers. This necessitates the development of new anticancer drugs with high efficacy and low toxicity without major environmental impact. Plants have a rich history of use in cancer therapy. Traditional medicinal plants and herbs have been used in treating cancer for several millennia in Asia, including India, China, Japan and Thailand, and are currently being used for cancer prevention and therapy worldwide (Efferth et al., Citation2007; Olaku & White, Citation2011). The use of natural products from plant origin as adjunct therapy alongside conventional cancer treatments is also on the rise (Dennis et al., Citation2009). There has been an increasing interest in identifying and isolating natural compounds from medicinal plants with the objective of developing novel anticancer drugs (Bishayee, Citation2012; Cragg et al., Citation2009).
Owing to harsh environmental stress conditions, including water scarcity, strong solar radiation, temperature variations and low nutrient soils, desert plants have developed effective defense systems allowing them to successfully mitigate the stressful conditions for survival. These defense systems include phytochemicals (secondary metabolites) endowed with remarkable bioactivities (Chawla et al., Citation2012; Croteau et al., Citation2000; Harlev et al., Citation2012a; Wang et al., Citation2007). The high concentrations of defensive metabolites in desert plants are known to impart antioxidant, anti-inflammatory, antimutagenic, antiviral, antifungal and antimicrobial capabilities to the plant, and also block detrimental UV irradiation.
Desert plant phytochemicals that protect the plants against stressful environments could also be useful in preventing and fighting adverse pathophysiological conditions and complex diseases, including cancer. Emerging preclinical studies have provided convincing evidence that desert plant-derived extracts, fractions and isolated pure phytochemicals display potent anticancer activities against a wide spectrum of tumor model systems (reviewed by Harlev et al., Citation2012b). Although many desert plants have been investigated for their antitumor properties, a large number of them still remain to be explored for possible therapeutic applications in several oncologic diseases.
We have initiated a comprehensive program to evaluate the cancer preventive and therapeutic potential of previously unexplored desert plants with the objective of developing novel agents for cancer prevention and therapy. The objective of the present investigation was to screen antitumor effects of selected desert plants, namely Achillea fragrantissima (Forssk.) Sch. Bip. (Compositae), Ochradenus baccatus Delile (Resedaceae), Origanum dayi Post (Lamiaceae), Phlomis platystegia Post (Lamiaceae) and Varthemia iphionoides Boiss (Compositae), against an in vitro cancer model utilizing the HepG2 human hepatocellular carcinoma cell line.
Materials and methods
Plant materials
The aerial parts of all plants were collected in February 2011 at the upper Quidod wadi by the town of Arad, located at the western edge of the Judean Desert, Israel (elevation 600 m). The plant materials were identified by Dr. Eli Harlev and confirmed by Dr. Hagar Leshner, Israel National Herbarium, Hebrew University, Jerusalem, Israel. Voucher specimens (Nos. HUJ-HERB-VP-102647, HUJ-HERB-VP-102648, HUJ-HERB-VP-102649, HUJ-HERB-VP-102650 and HUJ-HERB-VP-102651 for A. fragrantissima, P. platystegia, O. baccatus, and O. dayi, and V. iphionoides, respectively) have been deposited in the herbarium of the Hebrew University, Jerusalem, Israel.
Preparation of aqueous extracts
The fresh plant material was boiled with distilled water, cooled and paper filtered. Subsequently, the clear filtrate was evaporated to dryness using a laboratory evaporator. The biological tests were carried out using the crude dry water extracts.
Cell culture
HepG2 cells were obtained from the American Tissue Culture Collection (Manassas, VA) and maintained in Dulbecco’s modified Eagle’s medium (Sigma-Aldrich, St. Louis, MO) supplemented with 10% fetal bovine serum, 100 units/mL penicillin and 100 µg/mL streptomycin (all from HyClone, Logan, UT). The cells were grown at 37 °C under an atmosphere containing 5% CO2 in a humidified incubator (Napco Series 8000 WJ, ThermoFisher Scientific, Waltham, MA). Cells were subcultured at preconfluent densities using 0.25% trypsin-EDTA (Millipore, Phillipsburg, NJ).
Cell proliferation assay
The cell proliferation was assessed by the 3-(4,5-dimethyl thiazol-2-yl)-2,5-diphenyl tetrazolium bromide (MTT) assay as previously described (Bishayee et al., Citation2010). In short, HepG2 cells were plated onto 96-well tissue culture plates at a density of 4 × 103 cells/well in 100 µL of aforementioned media. After 24 h of culture, the medium was removed carefully and replaced with 100 µL fresh medium, or medium with various concentrations of desert plant extracts for additional 24 or 48 h. Subsequently, 50 µL of MTT (5 mg/mL) was added to the wells containing 100 µL of media and cells were incubated for 4 h. The culture medium was removed and the formazan crystals (produced by the metabolic activities of cells) were dissolved by adding 100 µL of DMSO and 15 µL of glycine. The absorbance was measured at 570 nm with a SpectraMax 340 PC microplate spectrophotometer (Molecular Devices, Sunnyvale, CA). Each desert plant extract was tested for three to five times for each time point. For each experiment, four identical wells were used for each concentration.
Statistical analysis
Data are presented as mean ± standard deviation of the mean unless indicated otherwise. The one-way analysis of variance followed by the Student–Newman–Keuls test was performed for statistical analysis. The commercial software SigmaStat 3.1 (Systat software, Inc., San Jose, CA) was used for all statistical analysis. A value of p less than 0.05 was considered as statistically significant.
Results
In this study, aqueous extracts of aerial parts of five desert plants were prepared and used for in vitro experiments. The HepG2 cells were exposed to varying concentrations of each plant extract for 24 or 48 h and the cytotoxicity was measured by the MTT assay. The cytotoxic profiles are displayed in , and the half maximal inhibitory concentration (IC50) values are presented in . The addition of A. fragrantissima extract to the cell culture medium exhibited a marginal cell killing effect following 24 h () and moderate cytotoxicity after 48 h (). Nevertheless, results are statistically significant (p < 0.05) for the extract at a concentration equal to or more than 2.5 mg/mL for 48 h (). Ochradenus baccatus was found to possess moderate cytotoxicity after 24 h (), but a substantial growth inhibitory effect following 48 h (). A statistically significant (p < 0.05) result was observed for the extract at most of the concentrations for each time-point. The aqueous extract of O. dayi exhibited substantial antiproliferative effects at each time point (), with a maximum effect observed after 48 h (IC50 = 0.35 mg/mL), which is superior to that of any other plant extract at any time point. Interestingly, all concentrations of the extract yielded statistically significant (p < 0.05) results irrespective of the treatment period. Phlomis platystegia extract was found to be moderately effective only after 48 h (). Nevertheless, these results are not statistically significant. Similar results were obtained with V. iphionoides albeit slightly better activity compared to A. fragrantissima and P. platystegia following 48 h treatment (). At 48 h, the extract at 2.5 mg/mL or above exhibited statistically significant (p < 0.05) result.
Figure 1. Effects of aqueous extract of A. fragrantissima on the proliferation of HepG2 cells. Cells were plated into 96-well dishes (4000 cells/well) 24 h prior to the addition of the extract. Following 24 h (A) or 48 h (B) incubation with the extract, cell proliferation was determined by the MTT assay. Each data point represents mean ± standard deviation based on quadruplicate determinations in three to five independent experiments. *p < 0.05 compared to control.
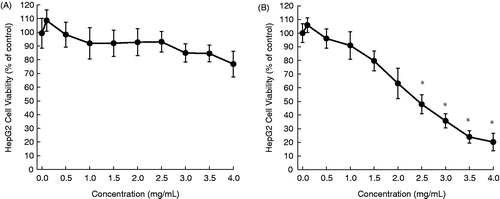
Figure 2. Effects of aqueous extract of O. baccatus on the proliferation of HepG2 cells. Cells were plated into 96-well dishes (4000 cells/well) 24 h prior to the addition of the extract. Following 24 h (A) or 48 h (B) incubation with the extract, cell proliferation was determined by the MTT assay. Each data point represents mean ± standard deviation based on quadruplicate determinations in three to five independent experiments. *p < 0.05 compared to control.
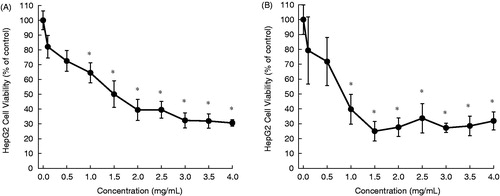
Figure 3. Effects of aqueous extract of O. dayi on the proliferation of HepG2 cells. Cells were plated into 96-well dishes (4000 cells/well) 24 h prior to the addition of the extract. Following 24 h (A) or 48 h (B) incubation with the extract, cell proliferation was determined by the MTT assay. Each data point represents mean ± standard deviation based on quadruplicate determinations in three to five independent experiments. *p < 0.05 compared to control.
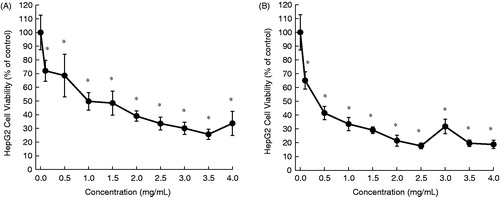
Figure 4. Effects of aqueous extract of P. platystegia on the proliferation of HepG2 cells. Cells were plated into 96-well dishes (4000 cells/well) 24 h prior to the addition of the extract. Following 24 h (A) or 48 h (B) incubation with the extract, cell proliferation was determined by the MTT assay. Each data point represents mean ± standard deviation based on quadruplicate determinations in three to five independent experiments.
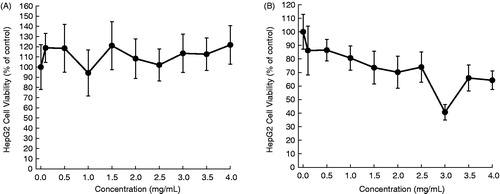
Figure 5. Effects of aqueous extract of V. iphionoides on the proliferation of HepG2 cells. Cells were plated into 96-well dishes (4000 cells/well) 24 h prior to the addition of the extract. Following 24 h (A) or 48 h (B) incubation with the extract, cell proliferation was determined by the MTT assay. Each data point represents mean ± standard deviation based on quadruplicate determinations in three to five independent experiments. *p < 0.05 compared to control.
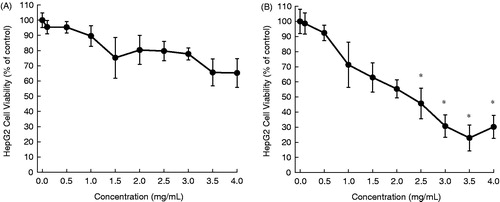
Table 1. Cytotoxicity of aqueous extracts of desert plants in HepG2 cells.
Discussion
Achillea fragrantissima () is a small perennial herb that grows in natural habitats in limestone wadies of Middle Eastern deserts. It has been used for many years in traditional medicine in the Arabia region for the treatment of gastrointestinal complications (Segal et al., Citation1987). The extracts and phytoconstituents from this plant have been shown to possess antioxidant (Al-Mustafa & Al-Thunibat, Citation2008), anti-inflammatory (Ageel et al., Citation1989; Elmann et al., Citation2011), antispasmodic (Mustafa et al., Citation1992), antimicrobial (Abutbul et al., Citation2005; Barel et al., Citation1991; El-Shazly et al., Citation2004), antiviral (Soltan & Zaki, Citation2009) and hypoglycemic (Yaniv et al., Citation1987) activities. Sathiyamoorthy et al. (Citation1999) reported a strong cytotoxic effect of an aqueous extract of the A. fragrantissima against cultured melanoma cell lines. A moderate antiproliferative effect of an aqueous extract from A. fragrantissima plant has been observed in our present study.
Figure 6. Deserts plants studied for anticancer potential – A. fragrantissima (A), O. baccatus (B), O. dayi (C), P. platystegia (D) and V. iphionoides (E).
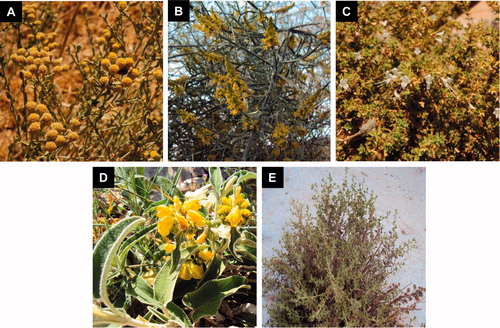
Ochradenus baccatus (), a phanerophyte shrub, grows in Mediterranean and Sudanian-African regions. Although this plant is used in folk medicine for the treatment of male sexual disorders, a recent study did not show any improvement of reproductive activity in male rats following chronic administration of O. baccatus extract (Soliman et al., Citation2012). Another study has reported hypoglycemic activity of O. baccatus extract in alloxan-induced diabetic rats (Shabana et al., Citation1990). An aqueous extract of O. baccatus has been found to exert antibacterial activity (Abutbul et al., Citation2005). Here, we have shown for the very first time that an aqueous extract of O. baccatus inhibits the growth of HepG2 tumor cells.
Origanum dayi () is another desert plant found in Mediterranean and Sudanian-African regions, especially in the northern Negev and Judean Desert in Israel. This endemic subshrub is known for its high yield of essential oils (Dudai et al., Citation2003). Origanum dayi has not yet been extensively studied for its biological activities. Results of our present investigation reveal a striking antitumor potential of this plant.
Phlomis platystegia () is an herbaceous plant native to Mediterranean and Irano-Turanian regions. Very limited information is available regarding biological activity of this plant. Based upon our results, an aqueous extract of this plant possesses moderate antitumor effect in vitro against HepG2 cells.
Varthemia iphionoides (), a bushy perennial with many branched aromatic and sticky stems, grows wild in rocky habitats of the Mediterranean, Saharo-Arabian and Irano-Turanian regions. The aqueous extract of this herb is commonly used in Jordanian folk-medicine for the treatment of gastrointestinal ailments and diabetes mellitus (Afifi et al., Citation1997). Various extracts and pure compounds isolated from this plant have been shown to exert antioxidant (Al-Dabbas et al., Citation2006a,Citationb; Al-Mustafa & Al-Thunibat, Citation2008), antibacterial (Al-Dabbas et al., Citation2005, Citation2006b; Abu-Hijleh et al., Citation2009; Darwish & Aburjai, Citation2010), antifungal (Afifi et al., Citation1991), antiplatelet (Afifi & Aburjai, Citation2004) and antihyperglycemic (Afifi et al., Citation1997; Kasabri et al., Citation2011) activities. A solitary study has shown pronounced cytotoxic effects of hexane, chloroform and ethanol extracts of aerial parts of V. iphionoides against HL-60 human leukemia cells (Al-Dabbas et al., Citation2006b). The results of our present investigation confirm the in vitro antitumor activity of this plant.
We have used the MTT assay to measure the effects of desert plant extracts on cell proliferation. Tetrazolium salt reduction assays are widely used to monitor metabolic activity or cell proliferation. Nevertheless, a number of phytochemicals can interfere with the procedure (Elisia et al., Citation2008; Jaszczyszyn & Gasiorowski et al., Citation2008). Hence, it may be a particular problem with extracts that are likely to have antioxidant activity as in the present work. Moreover, effects on cytotoxicity tend to correlate with cell proliferation, but that is not always the case. In view of these challenges, it would be a prudent approach to use an additional technique to measure cell proliferation, such as cell counting or the sulforhodamine B staining procedure.
Although preliminary in nature, results presented in this communication strongly underscore the value of desert plants in developing potential anticancer drugs. The exact phytochemicals responsible for the observed antiproliferative effects are precisely not known at the present time. Nevertheless, previous investigations have identified various groups of phytoconstituents, including flavones (e.g., cirsiliol), flavonols (quercetin glycosides) and triterpenoids (1,8-cineole, fragranol and terpenen-4-ol), present in the desert plants we studied (Afifi et al., Citation1991; Al-Dabbas et al., Citation2006a; Barakat et al., Citation1991; Dudai et al., Citation2003; El-Shazly et al., Citation2004; Mustafa et al., Citation1992). Several aforementioned compounds have demonstrated potent antitumor activities in several in vitro and in vivo models of liver cancer (Bishayee et al., Citation2012; Thoppil & Bishayee, Citation2011). These compounds could explain, at least in part, the present findings.
Applying the “ecology-based theory” of plants’ chemical defense systems may furnish a guide when screening plants for their antineoplastic activities. This theory uses plant growth patterns (e.g., annual, herbaceous perennial, woody perennial and evergreen) to suggest plant species that produce beneficial biologically active secondary metabolites. In line with this approach, studies confirmed that evergreen and woody perennials are on the average significantly more capable in exerting anticancer properties than the others (Donaldson & Cates, Citation2004). The results obtained in the present research are in line with this premise. Origanum dayi, the plant displaying the best antitumor results, is an evergreen perennial, and O. baccatus is an evergreen, woody perennial, while none of the other plants (A. fragrantissima, P. platystegia and V. iphionoides) are evergreen or woody. However, more studies on plants with differential growth patterns are warranted to confirm the results of our observation.
Future research will focus on continuing the screening of desert plants for their potential anticancer attributes, while the most promising will be subjected to an in-depth investigation. Thus, plant screening will be followed by fraction screening. Fractionation according to varying polarity seems to be a promising approach. Scientific literature describes the dependency of biological effects on fraction polarity. For example, the antiproliferative activity of the hexane extract of V. iphionoides proved to be 6-fold higher than that of the ethyl acetate fraction, while the antioxidative activity of the hexane fraction was the smallest, reflecting the very low concentration of phenolic ingredients in the latter. On the other hand, fractions of medium polarity (e.g., ethyl acetate and chloroform) exhibited much higher antibacterial activities than either the low polar or the high polar fractions (Al-Dabbas et al., Citation2006b). In the third stage, the most active fraction will be subjected to chemical profiling, meaning, qualitative and quantitative analysis. Prominent components in this fraction will be isolated and their bioactivity tested with underlying mechanisms of action.
Conclusions
Based upon our results, we conclude differential antitumor responses of aqueous extracts of the aerial parts of selected desert plants, namely A. fragrantissima, O. baccatus, O. dayi, P. platystegia and V. iphionoides. Origanum dayi and O. baccatus exhibited substantial cytotoxicity against HepG2 human liver cancer cells, V. iphionoides and A. fragrantissima produced moderate cell killing activity and minimal activity was observed with P. platystegia. Our results also indicate that the “ecology-based theory” of plants’ chemical defense systems may provide a vital clue while screening plants for their antitumor efficacy. The encouraging preliminary data on antiproliferative effects of O. dayi and O. baccatus may lead to the development of potential anticancer agents based on plants of desert origin.
Acknowledgements
We sincerely thank Richard d’Assalenaux, Pharm.D. for carefully editing the manuscript and Deepak Bhatia, Ph.D. (Northeast Ohio Medical University) for providing HepG2 cells.
Declaration of interest
A part of this work was performed at the Northeast Ohio Medical University (Rootstown, OH) supported by a new faculty start-up research grant to A. B. The authors declare no conflicts of interest.
References
- Abu-Hijleh A, Jarrar N, Adwan K. (2009). Antibacterial activity of common Varthemia, Varthemia iphionoides, ethanol extract alone and in combination with cefotaxime. Adv Biol Res 3:144–7
- Abutbul S, Golan-Goldhirsh A, Barazani O, et al. (2005). Screening of desert plants for use against bacterial pathogens in fish. Israeli J Aquaculture – Bamidgeh 57:71–80
- Afifi F, Al-Khalil S, Abdul-Haq BK, et al. (1991). Antifungal flavonoids from Varthemia iphionoides. Phytother Res 5:173–5
- Afifi F, Sakel M, Jaghabir M, Al-Eisawi D. (1997). Effect of Varthemia iphionoides on blood glucose level of normal rats and rats with streptozotocin-induced diabetes mellitus. Curr Therap Res 58:888–92
- Afifi FU, Aburjai T. (2004). Antiplatelet activity of Varthemia iphionoides. Fitoterapia 75:629–33
- Ageel AM, Mossa JS, Al-Yahya MA, et al. (1989). Experimental studies on antirheumatic crude drugs used in Saudi traditional medicine. Drugs ExptClin Res 15:369–72
- Al-Dabbas MM, Hashinaga F, Abdelgaleil SA, et al. (2005). Antibacterial activity of an eudesmane sesquiterpene isolated from common Varthemia, Varthemia iphionoides. J Ethnopharmacol 97:237–40
- Al-Dabbas MM, Kitahara K, Suganuma T, et al. (2006a). Antioxidant and α-amylase inhibitory compounds from aerial parts of Varthemia iphionoides Boiss. Biosci Biotechnol Biochem 70:2178–84
- Al-Dabbas MM, Suganuma T, Kitahara K, et al. (2006b). Cytotoxic, antioxidant and antibacterial activities of Varthemia iphionoides Boiss. extracts. J Ethnopharmacol 108:287–93
- Al-Mustafa AH, Al-Thunibat OY. (2008). Antioxidant activity of some Jordanian medicinal plants used traditionally for treatment of diabetes. Pak J Biol Sci 11:351–8
- Barakat HH, El-Mousallamy AMD, Souleman AMA, Awadalla S. (1991). Flavonoids of Ochradenus baccutus. Phytochemistry 30:3777–9
- Barel S, Segal R, Yashphe J. (1991). The antimicrobial assay of the essential oil from Achillea fragrantissima. J Ethnopharmacol 33:187–91
- Bishayee A. (2012). Editorial: Current advances in cancer prevention and treatment by natural products. Curr Pharm Biotechnol 13:115–16
- Bishayee A, Háznagy-Radnai E, Mbimba T, et al. (2010). Anthocyanin-rich black currant extract suppresses the growth of human hepatocellular carcinoma cells. Nat Prod Commun 5:1613–18
- Bishayee A, Thoppil RJ, Waghray A, et al. (2012). Dietary phytochemicals in the chemoprevention and treatment of hepatocellular carcinoma: In vivo evidence, molecular targets, and clinical relevance. Curr Cancer Drug Targets 12:1191--232
- Boyle P, Levin B. (2008). World Cancer Report 2008, International Agency for Research on Cancer. Available from: http://www.iarc.fr/en/publications/pdfs-online/wcr/2008/ [last accessed on 24 Sep 2012]
- Chawla P, Chawla A, Vasudeva N, Sharma SK. (2012). A review of chemistry and biological activities of the genus Aerva – a desert plant. Acta Pol Pharm 69:171–7
- Cragg GM, Grothaus PG, Newman DJ. (2009). Impact of natural products on developing new anti-cancer agents. Chem Rev 109:3012–43
- Croteau R, Kutchan TM, Lewis GT. (2000). Natural products (secondary metabolites). In: Buchanan BB, Gruissem W, Jones LR, eds. Biochemistry and Molecular Biology of Plants. Rockville: American Society of Plant Physiologists, 1250–68
- Darwish RM, Aburjai TA. (2010). Effect of ethnomedicinal plants used in the folklore medicine in Jordan as antibiotic resistant inhibitors of Escherichia coli. BMC Complement Altern Med 10:9
- Dennis T, Fanous M, Mousa S. (2009). Natural products for chemopreventive and adjunctive therapy in oncologic disease. Nutr Cancer 61:587–97
- Donaldson J, Cates R. (2004). Screening for anticancer agents from Sonorant desert plants: A chemical ecology approach. Pharm Biol 42:478–87
- Dudai N, Larkov O, Chaimovitsh D, et al. (2003). Essential oil compounds of Origanum dayi Post. Flavour Fragrance J 18:334–7
- Efferth T, Li PC, Konkimalla VS, Kaina B. (2007). From traditional Chinese medicine to rational cancer therapy. Trends Mol Med 13:353–61
- Elisia I, Popovich DG, Hu C, Kitts DD. (2008). Evaluation of viability assays for anthocyanins in cultured cells. Phytochem Anal 19:479–86
- Elmann A, Mordechay S, Erlank H, et al. (2011). Anti-neuroinflammatory effects of the extracts of Achillea fragrantissima. BMC Complement Altern Med 11:98
- El-Shazly AM, Hafez SS, Wink M. (2004). Comparative study of the essential oils and extracts of Achillea fragrantissima (Forssk.) Sch. Bip. and Achillea santolina L. (Asteraceae) from Egypt. Pharmazie 59:226–30
- Harlev E, Nevo E, Lansky EP, et al. (2012a). Anticancer potential of aloes: Antioxidant, antiproliferative, and immunostimulatory attributes. Planta Med 78:843–52
- Harlev E, Nevo E, Lansky EP, et al. (2012b). Anticancer attributes of desert plants: A review. Anticancer Drugs 23:255–71
- Jaszczyszyn A, Gasiorowski K. (2008). Limitations of the MTT assay in cell viability testing. Adv Clin Exp Med 17:525–9
- Jemal A, Bray F, Center MM, et al. (2011). Global cancer statistics. CA Cancer J Clin 61:69–90
- Kasabri V, Afifi FU, Hamdan I. (2011). Evaluation of the acute antihyperglycemic activity effects of four selected indigenous plants from Jordan used in traditional medicine. Pharm Biol 49:687–95
- Mustafa EH, Abu Zarga M, Abdalla S. (1992). Effects of cirsiliol, a flavone isolated from Achillea fragrantissima, on rat isolated ileum. Gen Pharmacol 23:555–60
- Olaku O, White JD. (2011). Herbal therapy use by cancer patients: A literature review. Eur J Cancer 47:508–514
- Sathiyamoorthy P, Lugasi-Evgi H, Schlesinger P, et al. (1999). Screening for cytotoxic and antimalarial activities in desert plants of the Negev and Bedouin market plant products. Pharm Biol 37:188–95
- Segal RA, Duddeck DH, Kajtar M. (1987). The sesquiterpene lactones from A. fragrantissima. Tetrahedron 43:4125–32
- Shabana MM, Mirhom YW, Genenah AA, et al. (1990). Study into wild Egyptian plants of potential medicinal activity. Ninth communication: Hypoglycaemic activity of some selected plants in normal fasting and alloxanised rats. Arch Expt Vet Med 44:389–94
- Siegel R, Naishadham D, Jemal A. (2012). Cancer statistics, 2012. CA Cancer J Clin 62:10–29
- Soliman GA, Donia Ael R, Awaad AS, et al. (2012). Effect of Emex spinosa, Leptadenia pyrotechnica, Haloxylon salicornicum and Ochradenus baccatus extracts on the reproductive organs of adult male rats. Pharm Biol 50:105–12
- Soltan MM, Zaki AK. (2009). Antiviral screening of forty-two Egyptian medicinal plants. J Ethnopharmacol 126:102–7
- Thoppil RJ, Bishayee A. (2011). Terpenoids as potential chemopreventive and therapeutic agents in liver cancer. World J Hepatol 3:228–49
- Wang W, Chen J, Li J, et al. (2007). Extraordinary accumulations of antioxidants in Ammopiptanthus mongolicus (Leguminosae) and Tetraena mongolica (Zygophyllaceae) distributed in extremely stressful environments. Bot Stud 48:55–61
- World Health Organization (2008). The Global Burden of Disease: 2004 Update. Geneva: World Health Organization
- Yaniv Z, Dafni A, Friedman J, Palevitch D. (1987). Plants used for the treatment of diabetes in Israel. J Ethnopharmacol 19:145–51