Abstract
Context: Oxidative stress may contribute to lymphedema and subsequent tissue damage. However, the causal role of oxidative stress in lymphedema remains unclear.
Objective: We attempted to detect and identify the free radicals formed in lymphedema fluid and assessed the protective mechanisms and effects of specific enzyme inhibitors and natural antioxidants.
Materials and methods: To study the level of postsurgical oxidative stress with lymphedema in a mouse tail model, we used an electron spin resonance (ESR) method and an ascorbyl radical’s ESR spectrum as an oxidative stress biomarker. The drug-treatment group received an i.p. injection with indomethacin (2 mg/kg), baicalein (15 mg/kg), MK-886 (3 mg/kg), zileuton (6.25 mg/kg), diphenyleneiodonium (DPI; 1 mg/kg), sulforaphane (30 mg/kg), oryzanol (30 mg/kg) or sesamol (30 mg/kg) once daily for 14 d from the day of operation. All animals were sacrificed on day 14.
Results: Administration of indomethacin, sulforaphane, oryzanol and sesamol significantly suppressed both the tail volume (56.9%, 77.8%, 72.2% and 38.1% inhibition, respectively, p < 0.01) and ascorbyl radical signals (31.4%, 54.5%, 79.3% and 57.1% inhibition, respectively, p < 0.01), compared with the control mice. No significant differences were found between any of the baicalein, MK-886, or zileuton groups compared with the control. DPI suppressed the tail volume (25.9% inhibition, p < 0.01) but not the ascorbyl radical signals.
Conclusion: This study showed that COX-derived oxidative stress plays a major role in the pathological mechanisms of surgically induced lymphedema. Indomethacin, sulforaphane, oryzanol and sesamol exhibit potent protective properties against surgically induced lymphedema.
Introduction
Lymphedema can be conceptually divided into primary or congenital lymphedema and acquired or secondary lymphedema. The former condition is caused by an inherent defect in the lymphatic vessels or lymph nodes, whereas the latter condition is associated with the type of damage to the lymphatic system frequently caused by cancer or its treatment. Lymphedema involves the expansion of lymph capillaries, and morphological and functional damage to endothelial cells is observed (Knight et al., Citation1987). In addition, severe regional oxygen insufficiency, followed by reperfusion periods, can occur in lymphedematous tissue. Therefore, lymphedema can be regarded as a form of tissue damage associated with an ischemia-reperfusion process.
Free radical formation is generally thought to play a crucial role in tissue damage during ischemia-reperfusion. Siems et al. (Citation2002) suggested that oxidative stress might contribute to lymphedema and subsequent tissue damage. Antioxidants such as coumarin- and flavonoid-type compounds reduce the swelling in all types of lymphedema (Bruns et al., Citation2003), and selenium shows a beneficial effect in radiation-induced lymphedema (Bruns et al., Citation2004; Micke et al., Citation2003). These findings indicate that free radical formation might be involved in the pathogenic mechanisms of the lymphedema. However, the causal role of oxidative stress in this condition remains unclear. A greater knowledge of the molecular pathogenic mechanisms is imperative to provide novel perspectives for lymphedema treatment.
Numerous natural compounds obtained from the daily diet or plant-derived dietary supplements display antioxidant properties, and have been shown to prevent free-radical-mediated diseases by neutralizing cell oxidative stress. Sulforaphane, a constituent rich in cruciferous vegetables, has been extensively studied in recent decades as a potential antioxidant and chemopreventive compound (Liang & Yuan., Citation2011). Similarly, oryzanol, a phytochemical constituent of rice bran, has been widely studied for its antioxidant, anti-inflammatory, anti-ulcerogenic, hypolipidemic, antineoplastic, antidiabetic and anti-allergic activities in pathological processes (Islam et al., Citation2011). Another widely recognized natural antioxidant is sesamol, a constituent of sesame oil. Sesamol has been shown to possess anti-inflammatory (Chavali et al., Citation2001), neuroprotective (Hou et al., Citation2006), hepatoprotective (Hsu et al., Citation2006) and anti-aging properties (Sharma & Kaur., Citation2006).
Although several experimental animal models, including dog hindlimb (Han et al., Citation1985), rat hindlimb (Kanter et al., Citation1990) or rabbit ear (Szuba et al., Citation2002) have been used for the study of secondary lymphedema by disturbing lymphatic transport through the removal of a circumferential band of skin and subcutaneous tissue, a new mouse model of acquired lymphatic insufficiency, reported by Tabibiazar et al. (Citation2006), appears to be suited for molecular studies on this aspect. Therefore, in this study, we used a mouse lymphedema model to detect and identify the free radicals using electron spin resonance (ESR) and spin-trapping methods. We assessed the protective mechanisms and effects of specific enzyme inhibitors and several natural antioxidants, including sulforaphane, oryzanol and sesamol ().
Materials and methods
Materials
Baicalein, chloral hydrate, 5,5-dimethyl-1-pyrroline N-oxide (DMPO), dimethyl sulfoxide (DMSO), diphenyleneiodonium (DPI), indomethacin, sodium citrate and sulforaphane were purchased from Sigma Chemical (St. Louis, MO). Zileuton was purchased from Tocris Bioscience (Bristol, UK). Oryzanol was purchased from Wako Pure Chemical Industries (Osaka, Japan). MK866 was purchased from Cayman (Ann Arbor, MI). Sesamol was purchased from Aldrich Chemical (Milwaukee, WI).
Animal model
An experimental model of acute postsurgical lymphedema in the tails of mice was set up, as described by Tabibiazar et al. (Citation2006) with some modifications. In brief, male ICR mice (28–38 g) were randomly divided into three groups: a drug-treatment group, a sham-operated control group and a control group. Mice were anesthetized by an intraperitoneal (i.p.) injection of chloral hydrate (400 mg/kg). A ring of skin measuring 6–8 mm-wide was removed, 16 mm distal to the tail base. The major lymphatic trunks were identified through subcutaneous injection of methylene blue distal to the surgical incision, followed by controlled, limited cautery ablation of these structures. In the sham-operated control group, a skin incision alone was performed, with methylene blue injection but without lymphatic cautery. The normal control group underwent no surgical manipulation. The drug-treatment group received an i.p. injection of drug dissolved in solvent (0.5% DMSO in normal saline) once daily for 14 d from the day of operation. All animal subjects were sacrificed on day 14. Plasma and interstitial fluid were collected from lymphedematous mice for the ESR study.
All animal protocols were approved by the Laboratory Animal Center of Taipei Medical University.
Measurement of tail volume
Postsurgical lymphedema was experimentally created in the mouse tails. The tail volume, from the tip to the distal edge of the wound, was measured using the water displacement method (Rutkowski et al., Citation2006). In untreated mice, a circumferential mark was made on the tail, 16 mm from the tail base to mimic the injury site, so that the measurement could be replicated. The tails of all mice were measured daily until the mice were sacrificed on postsurgical day 14.
Preparation of mouse plasma
Blood was collected from normal and lymphedematous mice and was mixed with a solution of acid/citrate/dextrose (ACD) (blood:ACD, 9:1 v/v). The samples were centrifuged at 120 g for 10 min at room temperature, and then the supernatant (platelet-rich plasma) was collected for the ESR study.
Measurement of free radicals by ESR spectrometry
The ESR method was performed using a Bruker EMX ESR spectrometer, as described by Chou et al. (Citation2007) with some modifications. ESR spectra were recorded at room temperature using a quartz flat cell designed for aqueous solutions. We used DMPO as the spin-trap agent and measured ESR signals from the mouse interstitial fluid and plasma. The conditions of ESR spectrometry were as follows: 20 mW power at 9.78 GHz, with a scan range of 100 G and a receiver gain of 5 × 104. The modulation amplitude, sweep time and time constant are given in the figure legends.
Statistical analysis
The results of the analysis of experimental data are shown as the mean ± SEM and are accompanied by the number (n) of observations. We conducted analysis of variance to establish whether the differences among group means were statistically significant. If so, individual group comparisons were conducted using the Newman–Keuls method. A p value of < 0.05 was considered statistically significant.
Results
Acute experimental lymphedema: Degree and sustainability of swelling
This investigation involved studying 56 ICR mice. Of these, 48 underwent postsurgical lymphatic ligation, four served as surgical-sham controls, and the remaining four served as normal controls. Initial observation showed that in animals subjected to lymphatic ligation, the edema peaked on postsurgical day 14 and then gradually recovered (). During the experiment, we thus sacrificed the mice on day 14, for the ESR measurement. No statistically significant change was observed in tail volume for the surgical-sham or normal controls. In the lymphedema and solvent control groups, nearly equivalent swelling was observed ().
Measurement of free radicals in surgically induced lymphedema
We investigated free-radical generation in surgically induced lymphedema of the mouse tail by combining spin-trapping techniques with ESR spectroscopy. The mice were killed by dislocating the cervical spine. The interstitial fluid was immediately collected from the lymphedematous mice and incubated with DMPO (200 mM) for the ESR study. The g-value and hyperfine constant were analyzed using specialized analytical software installed in the ESR device. A typical ascorbyl radical obtained in our study is shown in . Its hyperfine constant (aH = 1.77 G) and g of 2.006 were extremely similar to those reported in previous studies (Duan et al., Citation2009; Ito et al., Citation2007, Citation2011; Masumizu et al., Citation2005). No significant amounts of oxygen-derived free radicals were detected in this study. It is possible that ascorbyl radical generation occurred secondarily to oxygen-derived free-radical-induced cellular damage (Pietri et al., Citation1990).
Figure 4. ESR spectra obtained from the (A) interstitial fluid and (B) plasma of a lymphedematous mouse in the presence of DMPO (100 mM). Instrument parameters were as follows: modulation amplitude, 1 G; time constant, 164 ms; and scanning for 42 s using three accumulated scans. The ESR spectra are labeled to show their components: DMPO-ascorbyl radical adduct (*).
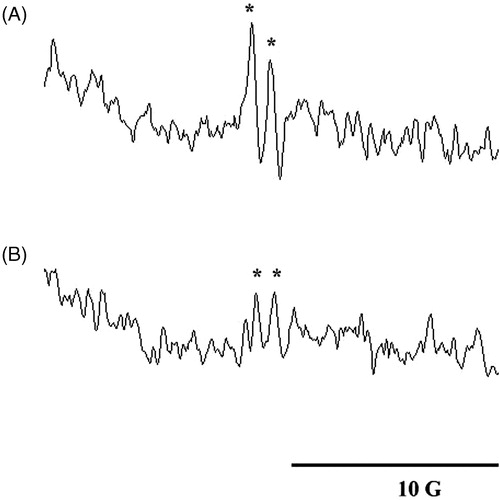
Effects of enzyme inhibitors on tail volume in lymphedematous mice
To study the mechanisms of surgically induced lymphedema of the mouse tail, we administered various enzyme inhibitors to the mice. These included indomethacin (a cyclooxygenase [COX] inhibitor; 2 mg/kg), baicalein (a 12/15-lipoxygenase [LOX] inhibitor; 15 mg/kg), MK-886 (a 5-LOX activating protein inhibitor; 3 mg/kg), zileuton (a 5-LOX inhibitor; 6.25 mg/kg) and DPI (an NADPH oxidase inhibitor; 1 mg/kg). The tail volumes of each group of animals are shown in . On postsurgical day 8, we observed protective effects associated with indomethacin, MK-886, zileuton, and DPI. However, on day 14 the only treatments yielding a significant reduction in tail volume were indomethacin and DPI (56.9% and 25.9% inhibition, respectively; p < 0.01 compared with the lymphedema group; ). These findings suggest that the COX and NADPH oxidase pathways play a major role in surgically induced lymphedema.
Effects of enzyme inhibitors on ascorbyl-radical signal in interstitial fluid
We monitored the formation of ascorbyl radicals following treatment with several enzyme inhibitors. The results showed that the formation of ascorbyl radicals was significantly reduced by indomethacin (31.4% inhibition; p < 0.01 compared with the lymphedema group). Treatment with baicalein, MK-886, zileuton, or DPI did not result in any significant changes (). The findings show that COX-derived free radicals might be the original free radicals produced in surgically induced lymphedema of the mouse tail. We defined the ascorbyl radical spectral amplitude by summing the signal height of the two absorption lines. shows the amplitude of the ESR ascorbate radical signals in each group.
Effects of enzyme inhibitors on ascorbyl-radical signal in plasma
We determined whether chronic lymphedema was associated with increased systemic oxidative stress or regional tail oxidative stress. The ascorbyl-radical signals in the plasma samples showed a reduction to approximately 45% of the level of the equivalent signals in the interstitial fluid samples (). Our results showed that treatment with indomethacin, MK-886, or DPI resulted in a slight decrease in ascorbyl-radical formation in the plasma, but without any statistical significance. Baicalein and zileuton showed no effects on ascorbyl-radical formation in the plasma ().
Figure 5. Effects of various enzyme inhibitors on ascorbyl-radical generation in mouse interstitial fluid. (A) ESR spectrum of ascorbyl radicals after pretreatment with or without each of the following: indomethacin, baicalein, MK-886, zileuton and DPI. (B) The amplitude of the ascorbate radical peak on the ESR signal. Data are expressed as the mean ± SEM. *p < 0.05 compared with the lymphedema group. (n = 4).
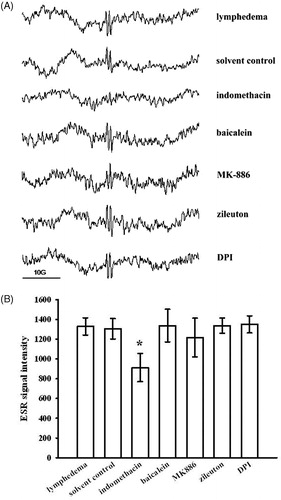
Effects of antioxidants on tail-volume changes in lymphedematous mice
To study the effects of antioxidants on surgically induced lymphedema of the mouse tail, we administered several natural dietary antioxidants to the mice. These included sesamol, sulforaphan, and oryzanol. The tail volumes of each group of animals are shown in . The results showed that sulforaphane (30 mg/kg), oryzanol (30 mg/kg) and sesamol (30 mg/kg) all individually and significantly decreased the tail volume on postsurgical day 14; the levels of inhibition were 77.8%, 72.2% and 38.1%, respectively ().
Figure 6. Effects of indomethacin, baicalein, MK-886, zileuton and DPI on ascorbyl-radical generation in the plasma of mice with surgically induced lymphedema. Data are expressed as the mean ± SEM (n = 4).
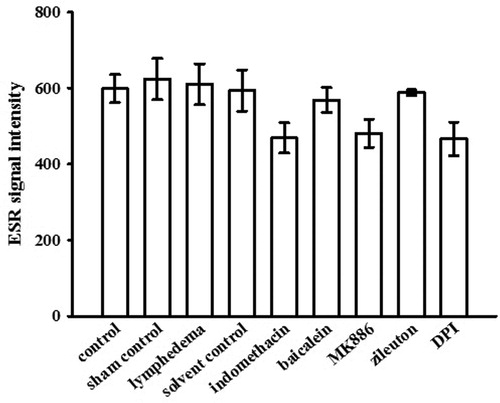
Figure 7. Effects of various antioxidants on a lymphedema mouse model. (A) Tail volume changes and (B) ascorbyl radical generation were measured after pretreatment with or without each of the following: oryzanol, sulforaphane and sesamol. Data are expressed as the mean ± S.E.M. (n = 4). ***p < 0.001 compared with the lymphedema group.
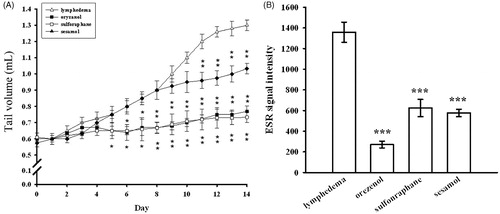
Effects of sesamol, sulforaphane, and oryzanol on ascorbyl-radical signal in interstitial fluid
The formation of ascorbyl radicals in surgically induced lymphedema of the mouse tails was monitored following treatment with several natural dietary antioxidants. Our results showed that the formation of ascorbyl radicals was significantly reduced by sulforaphane (30 mg/kg), oryzanol (30 mg/kg), and sesamol (30 mg/kg); the levels of inhibition were 54.5%, 79.3% and 57.1%, respectively (p < 0.001 compared with the lymphedema group; ).
Discussion
The exposure of plasma to oxidative stress leads primarily to oxidation of the endogenous ascorbate and sulfhydryl groups. This is followed by progressive reductions in the levels of bilirubin, urate and α-tocopherol (Frei et al., Citation1988). A previous study showed that the plasma concentration of lipid hydroperoxide was prevented by ascorbate, but not by sulfhydryl groups, urate and α-tocopherol (Frei et al., Citation1988). Thus, ascorbyl radicals provide an appropriate biomarker during oxidative stress. In accordance with this, Pietri et al. (Citation1994) reported that ascorbyl free radical is a noninvasive marker of oxidative stress in human open-heart surgery. Duan et al. (Citation2009) employ an ascorbyl radical ESR spectrum as a biomarker to study the oxidative stress level of the influenza virus A FM1 subset-infected mouse. Similarly, Ito et al. (Citation2011) showed that ascorbyl radical signals are a valuable indicator for estimating postmortem duration and oxidative damage in various tissues. To clarify the pathophysiological mechanisms of secondary lymphedema, we examined the changes in oxidative stress by using a mouse tail edema model. The results showed that the level of ascorbyl radical signals in the interstitial fluid was significantly higher than in plasma (). This finding is consistent with that of a previous study, which showed that plasma or serum was more resistant to lipid peroxidation than was interstitial fluid. The reason is thought to be differences in the composition of peroxidizable substrates versus antioxidative compounds (Kurtel et al., Citation1992).
In living organisms, the formation of reactive oxygen species (ROS) is approximately balanced by antioxidant defense systems, which maintain homeostatic control over a cell’s oxidative state. Siems et al. (Citation2002) reported an increased formation of ROS and enhanced lipid peroxidation processes in chronically lymphedematous tissue. However, no information on the mechanisms of ROS production in secondary lymphedema has been documented in the literature.
ROS can be produced by the mitochondria and certain enzymes, such as NADPH oxidase, COX, nitric oxide synthases and LOX (Halliwell & Gutteridge, Citation2007). We suggest that lymphedema pathology is associated with a hypoxic-ischemic pattern, which may activate phospholipase A2; this is followed by the degradation of membrane phospholipids, resulting in an accumulation of arachidonic acid (AA) within the tissues. Simultaneously, COX is activated and this leads to the formation of prostaglandins and free radicals (Armstead et al., Citation1988). However, other studies have shown that 5-LOX (Li et al., Citation2009), 12/15-LOX (Jin et al., Citation2008) and NADPH oxidase (Chen et al., Citation2011) may also contribute to ROS formation during ischemic damage.
We propose that AA-metabolized enzymes, such as COX, LOX and NADPH oxidase, can be regarded as candidates for ROS generation. We used a mouse tail edema model to investigate this hypothesis, and tested each of these candidates using the corresponding inhibitors in each pathway. As shown in , indomethacin significantly reduced the generation of ascorbyl radical signals, suggesting a major role of COX in ROS formation in this mouse tail edema model. By contrast, treatment with baicalein, MK-886, zileuton or DPI failed to induce similar inhibition, thereby ruling out the possibility that LOX or NADPH oxidase play a major role. This notion is consistent with previous work that showed COX activity to be required for neurotoxicity in hypoxic and ischemic injury (Li et al., Citation2010). The homogenates from cells exposed to hypoxia showed increased levels of ascorbyl radicals compared with the controls, and treatment with a COX inhibitor reduced the magnitude of the ascorbyl radical signal (Li et al., Citation2010). Considering all of the findings, we suggest that COX-derived oxidative stress plays a major role in the pathological mechanisms of secondary lymphedema. This hypothesis would be consistent with our results showing that indomethacin and natural antioxidants diminished oxidative stress and reduced the volume of lymphedema in the mouse tail edema.
It was surprising, however, that DPI did not inhibit the ascorbyl radical formation but significantly decreased the tail volume. One interpretation of this observation is that NADPH oxidase may play a partial role in surgically induced lymphedema. In accordance with this hypothesis, Tang et al. (Citation2005) reported that NADPH oxidase contributes to intracerebral hemorrhage induced by collagenase and promotes brain edema in mouse. In addition, Woodfin et al. (Citation2011) demonstrated that bradykinin released during cerebral ischemia leads to IL-1β release, which in turn activates NADPH oxidase leading to blood-brain barrier breakdown and promotes brain edema.
The results of a recent study by Kashiwagi et al. (Citation2011) indicate that COX-2 might play a key role in lymphangiogenesis and restoration of the lymphatic flow in secondary lymphedema. Furthermore, celecoxib, a COX-2 inhibitor, was found to ameliorate experimental mouse tail lymphedema (Kashiwagi et al., Citation2011). Prior research has established that COX exists in 2 isoforms, COX-1 and COX-2, and that indomethacin inhibits both isoforms. However, the use of indomethacin is restricted because of its high incidence of ulcerogenic side effects. Several selective COX-2 inhibitors were introduced for clinical use and displayed remarkable anti-inflammatory properties and reduced gastric toxicity compared with indomethacin; however, severe cardiovascular side effects have been detected after long-term use (Dogne et al., Citation2005). Recent studies have investigated the use of indomethacin combined with an antioxidant devoid of ulcerogenic side effects, and have shown that the anti-inflammatory properties were retained (Bandgar et al., Citation2011; Bhandari et al., Citation2010; Doulgkeris et al., Citation2006). Based on our observations, we suggest that natural antioxidants displaying COX inhibitory activity might serve as promising novel therapeutic agents to treat human patients with lymphedema.
This study showed that the volume of lymphedema in mouse tails was reduced by treatment with sulforaphane, oryzanol or sesamol. These treatment compounds were also individually shown to diminish oxidative stress. Among the tested compounds, sesamol appeared to be the least reactive agent that decreased the volume of lymphedema. Previous studies have shown that sulforaphane exerts its anti-inflammatory and chemopreventive effects mainly by suppressing COX-2 expression (Guo et al., Citation2012; Shan et al., Citation2009; Sharma et al., Citation2011; Shibata et al., Citation2010). A similar pattern of COX-2 inhibitory activity was shown for oryzanol (Islam et al., Citation2008, Citation2011). However, to date no evidence has been documented on the COX-inhibitory effect of sesamol. According to these data, the anti-edema capacity of sesamol seems to be related with its deficiency in COX-inhibitory activity.
Our results conclusively indicate that ROS formation in surgically induced lymphedema occurs mainly via the COX pathway. Treatment with DPI reduced the volume of mouse tails but not the ascorbyl radical signals; this finding suggests that COX-derived oxidative stress was an important contributor to the edematous pathology, but was not the sole mechanism underlying it. The central roles played by COX and ROS in surgically induced lymphedema suggest that natural products exhibiting antioxidant and anti-inflammatory properties may offer therapeutic potential for treating secondary lymphedema. Sulforaphane and oryzanol are two examples of these natural compounds.
Declaration of interest
The authors state that they have no conflicts of interest to declare.
This work was supported by grants from Wan Fang Hospital of Taipei Medical University (99TMU-WFH-02-1) and Chi-Mei Medical Center at Taipei Medical University (99CM-TMU-14).
References
- Armstead WM, Mirro R, Busija DW, Leffler CW. (1988). Postischemic generation of superoxide anion by newborn pig brain. Am J Physiol 255:H401–3
- Bandgar BP, Sarangdhar RJ, Viswakarma S, Ahamed FA. (2011). Synthesis and biological evaluation of orally active prodrugs of indomethacin. J Med Chem 54:1191–201
- Bhandari SV, Parikh JK, Bothara KG, et al. (2010). Design, synthesis, and evaluation of anti-inflammatory, analgesic, ulcerogenicity, and nitric oxide releasing studies of novel indomethacin analogs as non-ulcerogenic derivatives. J Enzym Inhib Med Chem 25:520–30
- Bruns F, Buntzel J, Mucke R, et al. (2004). Selenium in the treatment of head and neck lymphedema. Med Princ Pract 13:185–90
- Bruns F, Micke O, Bremer M. (2003). Current status of selenium and other treatments for secondary lymphedema. J Support Oncol 1:121–30
- Chavali SR, Utsunomiya T, Forse RA. (2001). Increased survival after cecal ligation and puncture in mice consuming diets enriched with sesame seed oil. Crit Care Med 29:140–3
- Chen H, Yoshioka H, Kim GS, et al. (2011). Oxidative stress in ischemic brain damage: Mechanisms of cell death and potential molecular targets for neuroprotection. Antioxid Redox Sign 14:1505–17
- Chou DS, Lee JJ, Hsiao G, et al. (2007). Baicalein induction of hydroxyl radical formation via 12-lipoxygenase in human platelets: An ESR study. J Agric Food Chem 55:649–55
- Dogne JM, Supuran CT, Pratico D. (2005). Adverse cardiovascular effects of the coxibs. J Med Chem 48:2251–7
- Doulgkeris CM, Galanakis D, Kourounakis AP, et al. (2006). Synthesis and pharmacochemical study of novel polyfunctional molecules combining anti-inflammatory, antioxidant, and hypocholesterolemic properties. Bioorg Med Chem Lett 16:825–9
- Duan S, Gu L, Wang Y, et al. (2009). Regulation of influenza virus-caused oxidative stress by Kegan Liyan oral prescription, as monitored by ascorbyl radical ESR signals. Am J Chin Med 37:1167–77
- Frei B, Stocker R, Ames BN. (1988). Antioxidant defenses and lipid peroxidation in human blood plasma. Proc Natl Acad Sci USA 85:9748–52
- Guo S, Qiu P, Xu G, et al. (2012). Synergistic anti-inflammatory effects of nobiletin and sulforaphane in lipopolysaccharide-stimulated RAW 264.7 cells. J Agric Food Chem 60:2157–64
- Halliwell B, Gutteridge JMC. (2007). Free Radicals in Biology and Medicine. New York: Oxford University Press Inc
- Han LY, Chang TS, Hwang WY. (1985). Experimental model of chronic limb lymphedema and determination of lymphatic and venous pressures in normal and lymphedematous limbs. Ann Plast Surg 15:303–12
- Hou RC, Chen YS, Chen CH, et al. (2006). Protective effect of 1,2,4-benzenetriol on LPS-induced NO production by BV2 microglial cells. J Biomed Sci 13:89–99
- Hsu DZ, Chen KT, Li YH, et al. (2006). Sesamol delays mortality and attenuates hepatic injury after cecal ligation and puncture in rats: Role of oxidative stress. Shock 25:528–32
- Islam MS, Murata T, Fujisawa M, et al. (2008). Anti-inflammatory effects of phytosteryl ferulates in colitis induced by dextran sulphate sodium in mice. Br J Pharm 154:812–24
- Islam MS, Nagasaka R, Ohara K, et al. (2011). Biological abilities of rice bran-derived antioxidant phytochemicals for medical therapy. Curr Top Med Chem 11:1847–53
- Ito S, Mori T, Kanazawa H, Sawaguchi T. (2007). Differential effects of the ascorbyl and tocopheryl derivative on the methamphetamine-induced toxic behavior and toxicity. Toxicology 240:96–110
- Ito S, Mori T, Kanazawa H, Sawaguchi T. (2011). Estimation of the postmortem duration of mouse tissue by electron spin resonance spectroscopy. J Toxicol 2011:973172
- Jin G, Arai K, Murata Y, et al. (2008). Protecting against cerebrovascular injury: Contributions of 12/15-lipoxygenase to edema formation after transient focal ischemia. Stroke 39:2538–43
- Kanter MA, Slavin SA, Kaplan W. (1990). An experimental model for chronic lymphedema. Plast Reconstr Surg 85:573–80
- Kashiwagi S, Hosono K, Suzuki T, et al. (2011). Role of COX-2 in lymphangiogenesis and restoration of lymphatic flow in secondary lymphedema. Lab Invest 91:1314–25
- Knight KR, Collopy PA, McCann JJ, et al. (1987). Protein metabolism and fibrosis in experimental canine obstructive lymphedema. J Lab Clin Med 110:558–66
- Kurtel H, Granger DN, Tso P, Grisham MB. (1992). Vulnerability of intestinal interstitial fluid to oxidant stress. Am J Physiol 263:G573–8
- Li W, Wu S, Ahmad M, et al. (2010). The cyclooxygenase site, but not the peroxidase site of cyclooxygenase-2 is required for neurotoxicity in hypoxic and ischemic injury. J Neurochem 113:965–77
- Li CT, Zhang WP, Lu YB, et al. (2009). Oxygen-glucose deprivation activates 5-lipoxygenase mediated by oxidative stress through the p38 mitogen-activated protein kinase pathway in PC12 cells. J Neurosci Res 87:991–1001
- Liang H, Yuan Q. (2011). Natural sulforaphane as a functional chemopreventive agent: Including a review of isolation, purification and analysis methods. Crit Rev Biotechnol [Online]. Available from: http://informahealthcare.com/doi/abs/10.3109/07388551.2011.604838 [last accessed 27 Sep 2011]
- Masumizu T, Noda Y, Mori A, Packer L. (2005). Electron spin resonance assay of ascorbyl radical generation in mouse hippocampal slices during and after kainate-induced seizures. Brain Res Brain Res Protoc 16:65–9
- Micke O, Bruns F, Mucke R, et al. (2003). Selenium in the treatment of radiation-associated secondary lymphedema. Int J Radiat Oncol Biol Phys 56:40–9
- Pietri S, Culcasi M, Stella L, Cozzone PJ. (1990). Ascorbyl free radical as a reliable indicator of free-radical-mediated myocardial ischemic and post-ischemic injury. A real-time continuous-flow ESR study. Eur J Biochem 193:845–54
- Pietri S, Séguin JR, d’Arbigny PD, Culcasi M. (1994). Ascorbyl free radical: A noninvasive marker of oxidative stress in human open-heart surgery. Free Radic Biol Med 16:523–8
- Rutkowski JM, Moya M, Johannes J, et al. (2006). Secondary lymphedema in the mouse tail: Lymphatic hyperplasia, VEGF-C upregulation, and the protective role of MMP-9. Microvasc Res 72:161–71
- Shan Y, Wu K, Wang W, et al. (2009). Sulforaphane down-regulates COX-2 expression by activating p38 and inhibiting NF-kappaB-DNA-binding activity in human bladder T24 cells. Int J Oncol 34:1129–34
- Sharma S, Kaur IP. (2006). Development and evaluation of sesamol as an antiaging agent. Int J Dermatol 45:200–8
- Sharma C, Sadrieh L, Priyani A, et al. (2011). Anti-carcinogenic effects of sulforaphane in association with its apoptosis-inducing and anti-inflammatory properties in human cervical cancer cells. Cancer Epidemiol 35:272–8
- Shibata A, Nakagawa K, Yamanoi H, et al. (2010). Sulforaphane suppresses ultraviolet B-induced inflammation in HaCaT keratinocytes and HR-1 hairless mice. J Nutr Biochem 21:702–9
- Siems WG, Brenke R, Beier A, Grune T. (2002). Oxidative stress in chronic lymphoedema. QJM 95:803–9
- Szuba A, Skobe M, Karkkainen MJ, et al. (2002). Therapeutic lymphangiogenesis with human recombinant VEGF-C. FASEB J 16:1985–7
- Tabibiazar R, Cheung L, Han J, et al. (2006). Inflammatory manifestations of experimental lymphatic insufficiency. PLoS Med 3:e254
- Tang J, Liu J, Zhou C, et al. (2005). Role of NADPH oxidase in the brain injury of intracerebral hemorrhage. J Neurochem 94:1342–50
- Woodfin A, Hu DE, Sarker M, et al. (2011). Acute NADPH oxidase activation potentiates cerebrovascular permeability response to bradykinin in ischemia-reperfusion. Free Radic Biol Med 50:518–24
Notice of Correction
In the version of this article published online ahead of print on 4th February 2013, the grant number for Chi-Mei Medical Center at Taipei Medical University contained incorrect information. The data has been corrected for this version.