Abstract
Context: Sulforaphane (SFN) [1-isothiocyanato-4-(methylsulfinyl)butane] is a naturally occurring isothiocyanate found in cruciferous vegetables such as broccoli [Brassica oleracea L. var. italica Plenck. (Brassicaceae)]. Since it is among the most potent bioactive components with antioxidant and antitumor properties, it has received intense attention in the recent years for its chemopreventive properties.
Objective: The present work determined the rehabilitating role in alleviating the oxidative damage caused by benzo(a)pyrene [B(a)P] to biomolecules and the apoptotic cascade mediated by orally administered isothiocyanate-SFN (9 µmol/mouse/day) against B(a)P (100 mg/kg body weight, i.p.) induced pulmonary carcinogenesis in Swiss albino mice.
Materials and methods: Oxidative damage was assessed by measuring lipid peroxidation, 8-hydroxydeoxyguanosine, hydrogen peroxide (H2O2) production, glycoprotein components, protein carbonyl levels and DNA-protein crosslinks. DNA fragmentation by agarose gel electrophoresis and caspase-3 activity by ELISA proved apoptotic induction by SFN along with the protein expression of Bcl-2, Bax and Cyt c.
Results: SFN treatment was found to decrease the H2O2 production (p < 0.001) in cancer induced animals, proving its antioxidant potential. Apoptosis was induced by increasing the release of Cyt c (p < 0.001) from mitochondria, decreasing and increasing the expression of Bcl-2 (p < 0.01) and Bax (p < 0.001), respectively. Caspase-3 activity was also enhanced (p < 0.001) which leads to DNA fragmentation in SFN treated groups.
Conclusion: Our results reflect the rehabilitating role of SFN in B(a)P induced lung carcinogenesis.
Introduction
Lung cancer arises as the result of numerous genetic lesions often caused by exposure to cigarette smoke or other environmental carcinogens. Benzo(a)pyrene [(B(a)P)] a major carcinogen in the cigarette smoke is a member of polycyclic aromatic hydrocarbon (PAH) family, and is often used as a model compound for PAH toxicity studies and in animal models of lung cancer (Silva et al., Citation2006). Equilibrium existing between reactive oxygen species (ROS) formation and endogenous antioxidant defense mechanisms can be disturbed by various chemical agents like B(a)P causing oxidative stress. This can result in injury to all the important cellular biocomponents contributing to the development of several pathological conditions including cancer. Cell recognition patterns are altered in a transformed cell, which is indicated by abnormal glycosylation pattern. The reactions of DNA with free radicals have been well established, which include strand breaks, DNA-protein cross-links and genomic base modifications (Paz-Elizur et al., Citation2003). These lead to the formation of DNA adducts which, if left unrepaired leads to mutation in genes involved in proliferation, growth and apoptosis and finally to a disease condition like cancer.
Two major mechanisms have been proposed for the chemopreventive effects of isothiocyanates. One mechanism involves isothiocyanate binding and inactivation of the phase I enzyme cytochrome P450s (Hecht, Citation2000) and induction of phase II enzymes, through activation of activator protein (Kong et al., Citation2001). The other mechanism emerging in recent years involves the induction of apoptosis (Yang et al., Citation2002) resulting in the deletion of genetically damaged cells, thus aborting clonal expansion. Sulforaphane (SFN), a potent cancer preventive agent, is a dietary isothiocyanate compound found in cruciferous vegetables such as broccoli [Brassica oleracea L. var. italica Plenck. (Brassicaceae)], and Brussels sprouts (Fahey et al., Citation2001). Oral administration of SFN inhibited or retarded experimental multistage carcinogenesis models including cancers of the stomach (Fahey et al., Citation2002), and lung (Hecht et al., Citation2002). The present study was undertaken to understand the protection afforded by low and dietary dose of SFN and its significance in mediating the apoptotic cascade against the toxicological effects of B(a)P in Swiss albino mice.
Materials and methods
Drugs and chemicals
B(a)P was purchased from Sigma Chemical Company, USA. SFN (>99% purity) was purchased from LKT Laboratories (St. Paul, MN). All other chemicals used were of analytical grade obtained from Sisco Research Laboratories Pvt. Ltd, Mumbai, India and Glaxo Laboratories, CDH division, Mumbai, India.
Animals
Healthy female Swiss albino mice (4–6 weeks old) weighing 15–20 g were used throughout the study. They were acclimated to laboratory condition with regular temperature control ranging from 23 ± 2 °C and were given ad libitum access to balanced diet (Gold Mohor rat feed, Ms. Hindustan Lever Ltd, Mumbai, India) and water. All the experiments were performed in compliance with the regulation of our institutional Animal Care and Use Committee. This research work on female Swiss albino mice was sanctioned and approved by our Institutional animal ethical committee (IAEC/No-02/077/2007).
Experimental design
The animals were divided into five groups with six mice in each group. Group I served as negative control animals treated with corn oil (vehicle) intraperitoneally. Group II animals (positive control) were treated with B(a)P (100 mg/kg body weight dissolved in corn oil, intraperitoneally) three times a week and left for 20 weeks to induce lung cancer. The method of cancer induction was adopted from Wattenberg et al. (Citation1997). Group III comprised of animals pre-treated orally with SFN (9 μmol/mouse/day) on alternate days for two weeks prior to the first dose of the carcinogen and continued until the 12th week and sacrificed (pre-treatment group). Group IV were cancer induced animals, treated with SFN from the 12th week to the 20th week (post-treatment group). Control animals treated with SFN alone served as group V (drug control). At the end of the experimental period, the animals were sacrificed and their blood, lung and liver tissues were used for further analyses. A 10% homogenate of lung and liver tissues were prepared in appropriate buffers as necessitated by the protocols. Dilutions were decided based on the protein concentrations determined by Lowry et al. (Citation1951).
Estimation of protein bound carbohydrates in serum and tissues
To 0.1 mL of serum, 2 mL of alcohol was added and centrifuged. The supernatant was decanted. The precipitate was hydrolyzed with acid to liberate protein-bound carbohydrate according to the method of Tettamant et al. (Citation1973) and expressed as mg/dL in serum. For the tissues a known amount of lung and liver tissues were defatted according to Folch et al. (Citation1957) and then hydrolyzed with 2 mL of 3 N HCl at 80 °C for 4 h. The hydrolyzed materials were neutralized and used for the analysis of hexose (Niebes, Citation1972), hexosamine (Wagner, Citation1979) and fucose (Dische & Shettles, Citation1948). The estimation of sialic acid by the method of Warren (Citation1959) with modification by Niebes (Citation1972) was adopted. All the individual glyco-conjugates were expressed as mg/100 mg defatted tissue.
Assay of lipid peroxidation and hydrogen peroxide
Lipid peroxidation (LPO) was estimated by using the method of Ohkawa et al. (Citation1979) and is expressed as nmol of malondialdehyde formed/h/mg protein in tissue. The hydrogen peroxide (H2O2) generation was assayed by the method of Pick & Keisari (Citation1981) and is calculated as μmoles of H2O2 consumed/min/mg protein.
Protein carbonyl and DNA protein crosslinks
The method of Levine et al. (Citation1994) was adopted for the determination of protein carbonyl content using 2,4-dinitrophenylhydrazine (2,4-DNPH) and the absorbance at 360 nm was measured. DNA protein crosslinks (DPCLs) were measured as described by Zhitkovich & Costa (Citation1992).
Measurement of 8-hydroxydeoxyguanosine
The DNA from the lung tissue samples was isolated using a commercial DNA extraction kit from Chromous Biotech, India. The extracted DNA was digested and centrifuged; the supernatant was used for the 8-hydroxydeoxyguanosine (8-OHdG) ELISA as per manufacturer’s protocol-Cell Biolabs, Inc. The 8-OHdG content in unknown samples was determined by comparison with predetermined 8-OHdG standard curve with the absorbance read at 450 nm as the primary wavelength.
Western blot analysis
Samples corresponding to 50 µg of protein, extracted from cytosolic fractions of the lung tissues were boiled with sample solubilising buffer for 5 min. Proteins were separated on 10% SDS-polyacrylamide gel electrophoresis and transferred on to a nitrocellulose membrane (Hybond CT, Amersham Life Sciences, Little Chalfont, UK) at 30 V for 3 h. The transferred membranes were incubated with appropriate primary antibodies and horseradish peroxidase-conjugated secondary antibodies. The immune complexes were detected by Super Signal West Pico Chemiluminescent substrate kit (Pierce, Appleton, WI) and captured in X-ray film and the bands were photographed. The relative density of the band was calculated by comparing the ratio of intensity of our band of interest to that of β-actin.
Assay of caspase 3
Caspase-3 enzyme activity was measured using the Sigma caspase-3 assay kit. The caspase-3 colorimetric assay kit is based on the hydrolysis of acetyl-Asp-Glu-Val-Asp p-nitroanilide (Ac-DEVD-pNA) by caspase-3, resulting in the release of the p-nitroaniline (pNA) moiety. The concentration of the pNA released from the substrate is calculated from the absorbance values at 405 nm (εmM = 10.5).
DNA fragmentation
Agarose gel electrophoresis is an effective method for the identification of purified DNA molecules. A 100 bp molecular weight marker DNA was simultaneously loaded in the first lane followed by samples in other lanes. The power supply was turned on and the current was adjusted to 40 mA. The run continued until the dye reached the end of the gel. Then the gel containing DNA was visualized in a UV transilluminator and photographed using the gel documentation system.
Statistical analysis
Data are presented as the mean ± standard deviation (SD). One-way analysis of variance was used to detect the significant changes between the groups with values of p < 0.05 indicating significance.
Results
Effect of SFN on glyco-conjugates
summarizes a significant increased for fucose and sialic acid with a significance of p < 0.001 and p < 0.01 for hexose and hexosamine in the cancer induced group II animals when compared with control animals (group I). Pre-treatment with SFN in group III decreased the levels of hexose and fucose signifying with p < 0.01 and hexosamine with p < 0.05 and sialic acid with p < 0.001, when compared with group II animals. Similarly, on post-treatment, there was a significant reduction (p < 0.01) for hexose and (p < 0.05) in all the other fractions of glycoproteins when compared to group II animals. In the liver tissue, the entire fraction was found to be increased (p < 0.05) in the group II animals when compared with group I animals. On pre-treatment with SFN, the levels were found to be decreased (p < 0.05) in group III for all the glyco-conjugates with sialic acid alone signifying p < 0.01. In group IV animals, a similar decrease (p < 0.05) was observed with insignificant changes with fucose when compared to group II B(a)P treated animals. Likewise, a significant increase in hexose (p < 0.01), hexosamine (p < 0.05), fucose and sialic acid (p < 0.001) was seen in the serum of cancer induced animals. A decrease signifying (p < 0.05) for hexose and hexosamine on pre- and post-treatment and (p < 0.01) and (p < 0.05) for fucose and sialic acid on pre- and post-treatment with SFN was obtained in the serum and are presented in .
Figure 1. Effect of SFN on the (a) circulating glyco-conjugates, (b) membrane LPO, and (c) H2O2 levels in B(a)P and SFN treated animals. Values are expressed as mean ± SD (n = 6 mice); a: as compared with group I; b and c: as compared with group II; Statistical significance: *p < 0.05; #p < 0.01; $p < 0.001; NS = not significant.
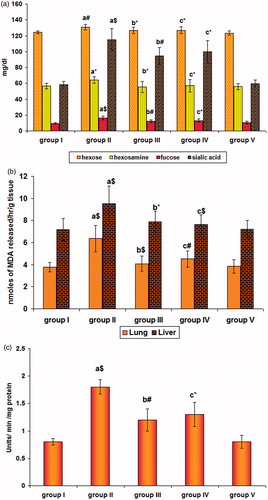
Table 1. Effect of SFN on the level of glyco-conjugates in lung and liver of control and experimental mice.
Effect of SFN on LPO and H2O2 levels
shows a significant (p < 0.001) increase in the extent of LPO in the lung and liver of cancer bearing group II animals when compared with the control animals (group I). Treatment with SFN in group III reduced the same, signifying with p < 0.001 in lung and p < 0.05 in liver. With group IV animals the reduction was signified with p < 0.01 in lung and p < 0.001 in liver when compared with B(a)P treated animals (group II). shows a significant increase (p < 0.001) in the level of H2O2 in the lung of cancer induced group II animals when compared with the control animals (group I). Treatment with SFN reduced the levels signifying with p < 0.01 and p < 0.05, respectively.
Effect of SFN on protein oxidation
represents the effect of SFN on the levels of protein carbonyls in the lung and liver of control and experimental mice. There was a significant increase (p < 0.001) and (p < 0.05) in the protein carbonyls in lung and liver of group II cancer bearing animals when compared with group I controls. Their levels significantly decreased in SFN-treated groups III (p < 0.01) and IV (p < 0.05) of lung tissue and had a similar effect in liver tissue with a significance of p < 0.001 and p < 0.01, respectively.
Figure 2. (a) Levels of protein carbonyls, (b) % of DNA-protein crosslinking, and (c) 8-OHdG levels in cancer induced and SFN-treated mice. Values are expressed as mean ± SD (n = 6 mice); a: as compared with group I; b and c: as compared with group II; Statistical significance: *p < 0.05; #p < 0.01; $p < 0.001; NS = not significant.
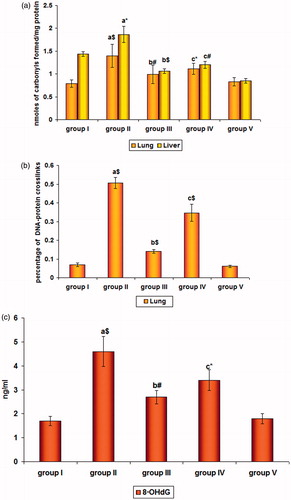
Effect of SFN on DNA-protein cross linking
illustrates the DNA damage assessed by the levels of % of DPCLs in the lung of control and experimental mice. There was a significant (p < 0.001) increase in the % of DPCL levels in the lung of group II animals when compared with group I controls. On treatment with SFN, their levels were significantly decreased (p < 0.001) in group III and group IV animals when compared with group II cancer induced animals.
Effect of SFN on the levels of 8-OHdG
The oxidative damage to DNA was reflected by the levels of 8-OHdG as shown in . The significant increase (p < 0.001) seen in group II animals dropped off in SFN pre-(p < 0.01) and post-treated (p < 0.05) groups III and IV indicating its protecting potential against the attack imposed by B(a)P which could be well correlated with the levels of H2O2 in our study.
Effect of SFN on caspase-3 activity
shows the activity of caspase-3 in the lung tissue extract of control and experimental mice. The activity was decreased (p < 0.001) in group II and profoundly increased (p < 0.001) in groups III and IV, respectively.
Figure 3. (a) Caspase-3 activity and (b) DNA fragmentation by agarose gel electrophoresis in cancer induced and SFN-treated mice. Lanes 1 and 5 – control and drug control group; Lane 2 – SFN pre-treated; Lane 3 – B(a)P treated, Lane 4 – SFN post-treated, Lane 6 – DNA ladder maker. Values are expressed as mean ± SD (n = 6 mice). (c) Immunoblot analysis of Bcl-2, Bax and Cyt c in lung homogenates of (1) control animals, (2) B(a)P treated animals, (3) SFN pre-treated and (4) SFN post-treated animals taking β-actin as the internal standard. Values are expressed as mean ± SD (n = 3); a: as compared with group I; b and c: as compared with group II; Statistical significance: *p < 0.05; #p < 0.01; $p < 0.001; NS = not significant.
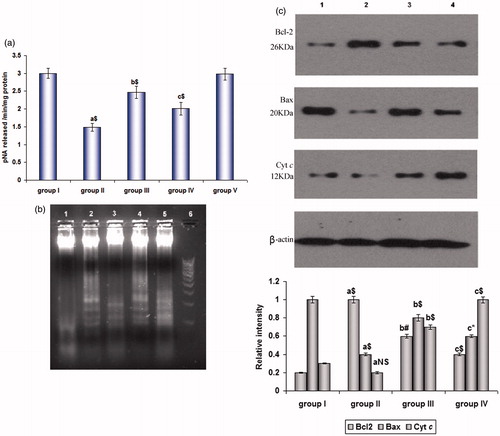
Effect of SFN on DNA fragmentation
depicts the DNA damage assessed by agarose gel electrophoresis in the extracted nuclear DNA of lung tissue. In group I animals (Lane 1), there was no significant intra-nucleosomal DNA fragmentation except for a shearing of the sample DNA. In Group II (Lane 3), a mild fragmentation was noticed in B(a)P treated animals, whereas the SFN-treated group III (Lane 2) and group IV (Lane 4) animals exhibited a significant DNA fragmentation.
Western blot analysis
represents a significant increase (p < 0.001) in the expression of Bcl-2 and decrease (p < 0.001) in the expression of Bax with insignificant changes in Cyt c was observed in the lung tissue of B(a)P-administered group II animals when compared with control. There was a significant decrease in the SFN pre- (p < 0.01) and post-treated (p < 0.001) groups in the level of Bcl-2 and a reflective increase in Bax as signified by p < 0.001 and p < 0.05 and for Cyt c as well (p < 0.001), respectively, in the cytosolic fractions of groups III and IV animals when compared to group I control animals. Group V, drug control animals did not show any significant changes when compared to the control group, hence were not included.
Discussion
Recent focus of cancer chemoprevention is on intermediate biomarkers capable of detecting early changes that can be correlated with inhibition of carcinogenic progression. Glycoproteins appear to serve many functions such as stabilizing protein structure (Pochec et al., Citation2003) and controlling cellular processes. Increased levels of hexose, hexosamine, fucose and sialic acid in cancer induced animals are indicative of the increased transferase activity. This is responsible for increased glycosylation due to the metabolic activation of B(a)P. A decrease in glycoprotein levels in both serum and the tissues seen in the SFN pre-treated group may be due to the blocking of metabolic activation of B(a)P hence preventing its transformation. This showed the definite involvement of carbohydrate moieties of the glycoprotein in malignancy.
LPO potential in B(a)P treated mice was exacerbated by increased generation of ROS, mainly H2O2. A differential elevation of superoxide dismutase caused by increased superoxide radicals leads to the inactivation of catalase leading to the accumulation of H2O2 levels. This was due to the decreased efficiency of the tissue antioxidant defense mechanisms, which led to breakdown of membrane structure and physiological function in the present study (Kalpana Deepa Priya et al., Citation2011b). The target specificity of B(a)P was found in the order of lungs > liver indicating it to be a lung specific carcinogen. In our study, SFN brought about a reduction in B(a)P-induced LPO level in pulmonary and hepatic tissues, suggesting its effectiveness to intercept the free radicals and protect cellular molecules from oxidative damage. Thus, a decreased H2O2 levels in groups III and IV animals were observed. The preservation of cellular membrane integrity by SFN depended on the protection or repair mechanisms capable of neutralizing the oxidative reactions.
ROS-induced formation of reactive carbonyl groups represent a major manifestation of oxidative modifications of proteins and amino acids in cells and tissues (England & Cotter, Citation2005) and reflects cellular damage. This might be the cause of increased protein carbonyls in tissue and serum of B(a)P-treated animals in our study. Removal of protective thiols, especially GSH (Kalpana Deepa Priya et al., Citation2011a), permit metabolically generated radicals to produce a higher level of DPC in B(a)P-treated mice during its metabolic activation. A protective action of GSH for the reduction of elevated DPC’s was reported earlier by Biaglow et al. (Citation1986) and is concurrent with our study. A subsequent decrease of carbonyl content by the treatment with SFN in groups III and IV was observed. This was due to a boost of the antioxidant pack, mainly the thioredoxin system (Kalpana Deepa Priya et al., Citation2011), which reduces oxidation of cysteine thereby protecting the cells from further damage. The DPCs dropped down simultaneously by SFN due to the replenishment of GSH levels. Further study on the role of GSH and other intracellular thiols in DPC formation and repair is needed.
The formation of 8-OHdG is a ubiquitous marker of oxidative stress, enhanced by chemical carcinogens which caused G → T and A → C transversions (Halliwell, Citation1999). This was reported as sites of spontaneous oncogene expression and ultimately cancer manifestation (Valko et al., Citation2004). The increase in 8-OHdG is mainly due to the disturbance in oxidant/antioxidant balance (Calişkan-Can et al., Citation2008) could enhance the mutation rate leading to lung cancer. This report is in accordance with our current results shown in in B(a)P-treated animals. Therefore, it is presumed that the oxygen radicals generated by B(a)P-induced toxicity in the lungs could be related to the formation of 8-OHdG. Their decreased levels in SFN-treated animals reflect its antioxidant potential that prevents the production of ROS or scavenge the excess free radicals or may be involved in DNA repair mechanisms.
The induction of apoptosis by isothiocyanates was first documented by Yu et al. (Citation1998). The Bcl-2 family of proteins, whose members may be anti-apoptotic or pro-apoptotic, regulate cell death (Burlacu, 2003) by controlling the mitochondrial membrane permeability during apoptosis. In our study, there is an alteration in the permeability of the membranes of the cell’s mitochondria, due to increased Bcl2 expression, which causes the translocation of the apoptogenic protein cytochrome c into the cytoplasm (). This, in turn, activates death-driving proteolytic proteins known as caspase, as is evident from caspase-3 activity () during the transduction of an apoptotic (death) signal into the cell. The shift in the balance of the cells towards their proliferative state is denoted by the inactivation of the caspase-3 in B(a)P-treated mice. The stimulation of apoptotic cascade by SFN is evident from the increased caspase-3 activity. The fragmentation seen in the induced group (, lane 3) might be an adaptive response to the toxic insult though not enough to overcome the condition. A significant DNA damage characteristic of apoptosis in the SFN-treated groups (, lanes 2 and 4) was seen in gel electrophoresis. Since, SFN was shown to disrupt tubulin polymerization (Jackson & Singletary, Citation2004), we reasoned that apoptosis induction by this phytochemical in the present study may be due to Bax activation which triggers the release of Cyt c as evidenced by its protein expression in different groups shown in . Our results also suggest that the upregulation of Bax and the downregulation of Bcl-2 might be another mechanism through which SFN-induced apoptosis.
Conclusion
We were able to demonstrate that orally administered SFN was capable of maintaining the cellular integrity and the homeostasis against the damage caused by B(a)P in vivo. SFN was able to shrink the production of ROS effectively, which further helped in maintaining the functional capability of the biological macromolecules by preventing aberrant glycosylation and genomic modifications. The apoptotic cascade mediated by SFN proves the modulation of Bcl2/Bax and the involvement of mitochondria-dependent pathway. Further studies are warranted to detect the molecular mechanisms involved in the process of carcinogenesis, which will be supportive for a targeted therapeutic strategy.
Declaration of interest
The authors declare that there are no conflicts of interest.
One of the authors, Ms. D. Kalpana Deepa Priya, gratefully acknowledges financial support in the form of a UGC scholarship under the UGC XI Plan Scheme of Research Fellowship in Sciences for Meritorious Students granted by the University Grants Commission, New Delhi. We also thank Mr. K.R. Anbu Chelian for his technical assistance in manuscript compilation.
References
- Biaglow JE, Varnes ME, Tuttle SW, et al. (1986). The effect of L-buthionine sulfoximine on the aerobic radiation response of A549 human lung carcinoma cells. Int J Radiat Oncol Biol Phys 12:1139–42
- Burlacu A. (2003). Regulation of apoptosis by Bcl-2 family proteins. J Cell Mol Med 7:249–57
- Calişkan-Can E, Firat H, Ardiç S, et al. (2008). Increased levels of 8-hydroxydeoxyguanosine and its relationship with lipid peroxidation and antioxidant vitamins in lung cancer. Clin Chem Lab Med 46:107–12
- Dische Z, Shettles LB. (1948). A specific colour reaction of CH3 pentose and a spectrophotometric micromethod for their determination. J Biol Chem 17:595–603
- England K, Cotter CT. (2005). Direct oxidative modifications of signaling proteins in mammalian cells and their effects on apoptosis. Redox Rep 10:237–45
- Fahey JW, Zalcmann AT, Talalay P. (2001). The chemical diversity and distribution of glucosinolates and isothiocyanates among plants. Phytochemistry 56:5–51
- Fahey JW, Haristoy X, Dolan PM, et al. (2002). Sulforaphane inhibits extracellular, intracellular, and antibiotic-resistant strains of Helicobacter pylori and prevents benzo[a]pyrene-induced stomach tumors. Proc Natl Acad Sci USA 99:7610–15
- Folch J, Lees M, Stanley GHS. (1957). A simple method for the isolation and purification of total lipids from animal tissues. J Biol Chem 226:497–509
- Halliwell B. (1999). Establishing the significance and optimal intake of dietary antioxidants: The biomarker concept. Nutr Rev 57:104–13
- Hecht SS. (2000). Inhibition of carcinogenesis by isothiocyanates. Drug Metab Rev 32:395–411
- Hecht SS, Kenney PM, Wang M, Upadhyaya P. (2002). Benzyl isothiocyanate: An effective inhibitor of polycyclic aromatic hydrocarbon tumorigenesis in A/J mouse lung. Cancer Lett 187:87–94
- Jackson SJT, Singletary KW. (2004). Sulforaphane: A naturally occurring mammary carcinoma mitotic inhibitor, which disrupts tubulin polymerization. Carcinogenesis 25:219–27
- Kalpana Deepa Priya D, Gayathri R, Gunassekaran GR, et al. (2011a). Chemopreventive role of sulforaphane by upholding the GSH Redox cycle in pre- and post-initiation phases of experimental lung carcinogenesis. Asian Pacific J Cancer Prev 12:103–10
- Kalpana Deepa Priya D, Gayathri R, Gunassekaran GR, Sakthisekaran D. (2011b). Inhibitory effect of sulforaphane against benzo(a)pyrene induced lung cancer by modulation of biochemical signatures in female Swiss albino mice. Asian J Biochem 6:395–405
- Kong AN, Owuor E, Yu R, et al. (2001). Induction of xenobiotic enzymes by the MAP kinase pathway and the antioxidant or electrophile response element (ARE/EpRE). Drug Metab Rev 33:255–71
- Levine RL, Williams JA, Stadtman ER, Shacter E. (1994). Carbonyl assays for determination of oxidatively modified proteins. Methods Enzymol 233:346–57
- Lowry OH, Rosenbrough NJ, Farr AL, Randall RJ. (1951). Protein measurement with the Folin phenol reagent. J Biol Chem 193:265–76
- Niebes P. (1972). Determination of enzyme and degradation products of GAG metabolism in the serum of healthy and varicose subjects. Clin Chim Acta 42:399–408
- Ohkawa H, Ohishi N, Yagi K. (1979). Assay for lipid peroxidation in animal tissues by thiobarbituric acid reaction. Anal Biochem 95:351–8
- Paz-Elizur T, Krupsky M, Blumenstein S, et al. (2003). DNA repair activity for oxidative damage and risk of lung cancer. J Natl Cancer Inst 95:1312–19
- Pick E, Keisari Y. (1981). Superoxide anion and H2O2 production by chemically elicited peritoneal macrophages-induction by multiple non-phagocytic stimuli. Cell Immunol 59:301–18
- Pochec E, Litynska A, Amoresano A, Casbarra A. (2003). Glycosylation profile of integrin alpha-3 beta-1 changes with melanoma progression. Biochim Biophys Acta 1643:113–23
- Silva BAK, Silva IS, Pereira DM, et al. (2006). Usefulness of argyrophilic nucleolar organizer regions in detection of lung cells alterations after benzo[a]pyrene instillation. Acta Cir Bras 2:136–9
- Tettamant G, Bonali F, Zambotti MV. (1973). A new procedure for the extraction, purification and fractionation of brain gangliosides. Biochim Biophys Acta 296:160–70
- Valko M, Izakovic M, Mazur M, et al. (2004). Role of oxygen radicals in DNA damage and cancer incidence. Mol Cell Biochem 266:37–56
- Wagner WD. (1979). A more sensitive assay, discriminating galactosamine and glucosamine in mixtures. Anal Biochem 94:394–6
- Warren L. (1959). The thiobarbituric acid assay of sialic acid. J Biol Chem 234:1971–5
- Wattenberg LW, Wiedmann TS, Estensen RD, et al. (1997). Chemoprevention of pulmonary carcinogenesis by aerosolized budesonide in female A/J mice. Cancer Res 57:5489–92
- Yang YM, Conaway CC, Chiao JW, et al. (2002). Inhibition of benzo(a)pyrene-induced lung tumorigenesis in A/J mice by dietary N-acetylcysteine conjugates of benzyl and phenethyl isothiocyanates during the post-initiation phase is associated with activation of mitogen-activated protein kinases and p53 activity and induction of apoptosis. Cancer Res 62:2–7
- Yu R, Mandlekar S, Harvey KJ, et al. (1998). Chemopreventive isothiocyanates induce apoptosis and caspase-3-like protease activity. Cancer Res 58:402–8
- Zhitkovich A, Costa M. (1992). A simple, sensitive assay to detect DNA-protein cross links in intact cells and in vivo. Carcinogenesis 13:1485–9