Abstract
Context: Cnestis ferruginea Vahl ex DC (Connaraceae) (CF) is used in traditional African medicine in the management of CNS disorders. The degeneration and dysfunction of cholinergic neurons is closely associated with the cognitive deficits of Alzheimer’s disease (AD) and oxidative stress has been implicated in its pathogenesis. However, the influence of C. ferruginea on the cholinergic system and oxidative stress parameters has not been explored.
Objective: The present study investigates the effect of methanol root extract of C. ferruginea and its active constituent amentoflavone (CF-2) on memory, oxidative stress and acetylcholinesterase (AChE) activity in scopolamine-induced amnesia.
Materials and methods: Mice were orally treated with CF (25–200 mg/kg), CF-2 (6.25–25 mg/kg) for three days and memory impairment was induced by intraperitoneal injection of scopolamine (3 mg/kg). Memory function was evaluated by passive avoidance and Morris water maze tests. Biochemical parameters of oxidative stress and cholinergic function were estimated in brain after the completion of behavioral studies.
Results: Scopolamine caused memory impairment along with increased AChE activity and oxidative stress in mice brain. Oral administration of CF and CF-2 significantly prevented scopolamine-induced memory impairment, inhibited AChE and enhanced antioxidant enzyme activity in the brain following scopolamine injection as compared to vehicle administration in scopolamine (i.p.)-treated mice that were comparable to the effect of tacrine.
Discussion and conclusion: The study demonstrated that C. ferruginea and its constituent have significant protective effect against scopolamine-induced memory deficits in mice that can be attributed to their antioxidant and antiAChE activity.
Introduction
Alzheimer’s disease (AD) is a progressive neurodegenerative disorder with memory loss as one of the earliest symptoms. It has been estimated to account for 50–60% of dementia cases in persons over 65 years of age worldwide (Francis et al., Citation1999). An estimated 5.4 million Americans of all ages have AD in 2012. This figure includes 5.2 million people aged 65 and older (Alzheimer’s Association, Citation2012; Hebert et al., Citation2003) and 200,000 individuals under age 65 who have early-onset AD (Alzheimer’s Association, Citation2006). One in eight people aged 65 and older (13%) have AD, and nearly half the people aged 85 and older (45%) have AD. In addition, of those with AD, an estimated 4% are under age 65, 6% are 65–74 while 44% are 75–84, and 46% are 85 or older (Alzheimer’s Association, Citation2012).
The pathological hallmarks of this disease are numerous senile plaques composed of beta amyloid (Aβ) peptide, neurofibrillary tangle formation, aberrant oxidative and inflammatory processes, neurotransmitter disturbances and cell loss in the affected brain regions, particularly in the areas that are important for learning and memory including the hippocampus and the prefrontal cortex (Hyman et al., Citation1989).
Cholinergic deficits are neuropathological occurrences that are consistently associated with memory loss and are correlated with the severity of AD (Giacobini, Citation2002). It has been shown that there are selective and excessive loss of cholinergic neurons, decreased acetylcholine (ACh) levels and reduced number of ACh receptors in AD brains (Francis et al., Citation1999; Guan et al., Citation2000). Blockade of central muscarinic ACh receptors disrupts learning and memory functions in rodents (Agrawal et al., Citation2009), nonhuman primates (Taffe et al., Citation1999) and humans (Riedel et al., Citation1995). Scopolamine, a muscarinic receptor antagonist, interferes with memory in animals and humans, particularly the processes of learning acquisition and short-term memory (Kulkarni et al., Citation2011; Sharma et al., Citation2010). The restoration of cholinergic function remains a rational target for developmental programs targeting the treatment of Alzheimer’s symptoms. Prolongation of the availability of ACh released into the neuronal synaptic cleft has been used as a means of enhancing cholinergic function in AD. This prolongation may be achieved by inhibiting ACh hydrolysis by acetylcholinesterase (AChE). AChE inhibitors, including rivastigmine, tacrine, donepezil and galantamine, are well-accepted pharmacological therapies for AD. However, AChE inhibitors present some limitations, such as their short half-lives and severe side effects (e.g., bradycardia, hypotension and convulsions), as well as hepatotoxicity, which is the most frequent and important side effect of these therapies (Bores et al., Citation1996).
However, at this point, there is no approved treatment with a proven disease-modifying effect in AD (Citron, Citation2010). There is therefore dire need for effective therapies. Before the development of modern medicine, people relied on a large arsenal of natural remedies for the treatment of CNS-related maladies. In both developed and developing societies, there has been increasing interest in herbal medicines, which are often perceived as more “natural” and “soft” treatments compared to synthetic drugs (WHO, Citation2006). Although the mechanisms of the antiamnesic effects of most herbal extracts and constituents are not yet fully understood, one or more of the components could be responsible for the activation of the central ACh function through the inhibition of AChE and activation of ACh synthesis (Zhang, Citation2004). Recent studies have pointed out that AD is associated with inflammatory processes. Reactive oxidative species (ROS) are able to damage cellular constituents and act as secondary messenger in inflammation. The use of antioxidants may be useful in the treatment of AD (Gilgun-Sherki et al., Citation2002).
Cnestis ferruginea Vahl ex DC (Connaraceae) is highly ubiquitous in the southern part of Nigeria. It was reported to have significant antioxidant activity (Oke & Hamburger, Citation2002). Recently, we reported the antistress potential (Ishola & Ashorobi, Citation2007) and analgesic and anti-inflammatory properties (Ishola et al., Citation2011) of C. ferruginea in rodents. However, the effect of C. ferruginea on memory function and central cholinergic system has not been explored. An activity-guided assay of the analgesic and anti-inflammatory effects of C. ferruginea has led to the isolation of amentoflavone (Ishola et al., Citation2012), a biflavone belonging to a large group of chemically related compounds known as flavonoids. These are polyphenolic compounds derived from dietary source and widely distributed in plant kingdom. Flavonoids are of major interests in the antioxidant-related research field due to their versatility and beneficial properties such as anti-inflammatory, cytostatic, anticarcinogenic, apoptotic, antiangiogenic, antioxidant and estrogenic properties (Banerjee et al., Citation2002). Growing lines of evidence have suggested that flavonoids have neuroprotective effects in many models of neurodegenerative diseases in vitro and in vivo (Kang et al., Citation2004; Youdim et al., Citation2002). Amentoflavone possesses neuroprotective effects on oxidative stress and amyloid β peptide-induced cell death in neuronal cells (Kang et al., Citation2005). In addition, amentoflavone was reported to exhibit significant β-site APP-cleaving enzyme inhibitory activity (Kang et al., Citation2005).
Scopolamine has been used to induce experimental models of AD (Beatty et al., Citation1986; Kopelman & Corn, Citation1988). Scopolamine significantly increases AChE and malondialdehyde (MDA) levels in the cortex and hippocampus (Fan et al., Citation2005; Jeong et al., Citation2008), and has been used to screen antiamnesic drugs for the age-related CNS dysfunction. The elevation of brain oxidative status after administration of amnesic doses of scopolamine further substantiates the value of scopolamine-induced amnesia as an animal model to test for drugs with potential therapeutic benefits in dementia (El-Sherbiny et al., Citation2003).
Based on our literature search, no study has been carried out to investigate the effect of C. ferruginea on memory function and central cholinergic system. We investigated whether the neuroprotective effects of C. ferruginea and amentoflavone ameliorated learning and memory impairment induced by scopolamine (muscarinic antagonist) in mice. To assess the antiamnesic effects of C. ferruginea and amentoflavone in mice, we evaluated the effect of C. ferruginea and amentoflavone on scopolamine-induced learning and memory deficits in the passive avoidance and Morris water maze tests. This study also evaluated the effect of C. ferruginea and its constituent on the biochemical marker of oxidative stress and AChE activity in the brains of mice with scopolamine-induced dementia.
Materials and methods
Animals
Male Swiss albino mice (25–35 g) were obtained from the Laboratory Animal Services Division of Central Drug Research Institute, Lucknow, India. The animals were kept in polyacrylic cages (22.5 cm × 37.5 cm) and maintained under standard housing conditions (room temperature 24–27 °C) and humidity (60–65%) with a 12 h light and dark cycle. Food (CDRI, laboratory animal feed) and water were available ad libitum but food was not allowed from 1 h prior to and until completion of the behavioral study. All procedures described were reviewed and approved by the Institutional Animal Ethics Committee (IAEC), Central Drug Research Institute, India. Adequate measures were taken to minimize pain or discomfort with animal experimental procedures.
Chemicals and reagents
Scopolamine, sodium hydroxide, Triton X-100, acetylthiocholine iodide, sodium chloride (NaCl), sodium nitrate (NaNO2), sulphanilamide, napthaylamine diamine dihydrochloric, bovine serum albumin (BSA), 5,5-dithiobis(2-nitro-benzoic acid) (DTNB), Folin-reagent, trichloroacetic acid (TCA) and 2-thiobabituric acid (TBA) were purchased from Sigma-Aldrich (St. Louis, MO).
Plant material
C. ferruginea dried roots were obtained from a local herb market in Mushin, Lagos State, Nigeria. Mr. Joseph Ariwaodo of the Forestry Research Institute of Nigeria (FRIN), Ibadan, Nigeria, and Prof. J.D. Olowokudejo of the Department of Botany and Microbiology, Faculty of Science, University of Lagos, Lagos, Nigeria, identified and authenticated the plant. A voucher specimen of the plant (Reference number: FHI 108219) was deposited at the herbarium of FRIN.
Preparation of extract
Powdered root of C. ferruginea (5.2 kg) was loaded into a glass percolator containing methanol (20 l). It was allowed to stand at room temperature (28 °C) for about 16 h (overnight). The percolate was collected and the process of extraction was repeated five times. The combined extract was filtered and concentrated on Buchi Rotavapor at 40 °C and was further dried under vacuum pump. The weight of the extract obtained was 560 g (brownish extract).
Isolation and identification of active compounds
The dried extract was suspended in water followed by successive partitioning with CHCl3, EtOAc and n-butanol, respectively. The aqueous/n-butanol fraction (160 g) was chromatographed on silica gel column, eluted with a gradient of CHCl3:MeOH (100:00–00:100). Fractions with similar Rf were pooled together and gave seven subfractions. Fr.4, being the most active, was rechromatographed as described above with the addition of 5% H2O, given amentoflavone (CF-2) (350 mg) and an amino acid (2.2 g) whose structure is still under investigation. CF-2 structure was identified by spectral data (IR, 1H NMR and 13C NMR, HMBC, HSQC), which was in accordance with those previously described (Ishola et al., Citation2012; Markham et al., Citation1987).
Experimental protocol and drug administration
Mice were randomly allotted to groups of 6–8 animals each and treatment was carried out as outlined below:
Group I (control): Normal saline (10 ml/kg/bw, p.o.) 1 h prior to training session on day 1 only.
Group II: Scopolamine (3 mg/kg, i.p.) 5 min before the commencement of day 1 trial.
Group III–IX: CF (25, 50, 100 and 200 mg/kg/bw, p.o.) and CF-2 (6.25, 12.5, 25 mg/kg/bw, p.o.), respectively, for three days and 1 h before intraperitoneal injection of scopolamine (3 mg/kg/bw). Scopolamine was given 5 min before the first trial on day 1 to assess antiamnesic effect of the extract on scopolamine-induced amnesia.
Group X: Tacrine (5 mg/kg/bw, i.p.) 1 h before intraperitoneal injection of scopolamine (3 mg/kg), which was given 5 min before the first trial as standard antiamnesic agent.
For oral and intraperitoneal administration, drugs were given in a volume of 0.1 ml/10 g. The animals were subjected to Morris water maze or passive avoidance test 5 min after scopolamine injection to evaluate the memory function.
Tests employed for learning and memory functions
Passive avoidance test
Five minutes after the administration of scopolamine or vehicle, mice were subjected to the passive avoidance test by placement in a light compartment of computerized shuttle box with a software program PACS 30 (Columbus Instruments, OH). After an acclimatization period of 30 s, the guillotine door was opened and closed automatically after entry of the mouse into the dark compartment. The subject received a low-intensity foot shock (0.5 mA; 10 s) in the dark compartment. Infrared sensors monitored the transfer of the animal from one compartment to another, which was recorded as transfer latency time (TLT) in seconds. The duration of a trial was 270 s. The first trial was for acquisition and retention was tested in a second trial given 24 h after the first trial. The shock was not delivered in the retention trials to avoid reacquisition. The criterion for learning was taken as an increase in the TLT on retention trials as compared to acquisition trial (Awasthi et al., Citation2010; Tota et al., Citation2009, Citation2010).
Morris water maze
Morris water maze with a video tracking was used to assess learning and memory in experimental mice (Columbus instruments, OH). It consists of a circular water tank (120 cm diameter and 50 cm height) located in a darkened test room, filled with water (26 ± 2 °C) to a depth of 30 cm. Four equally spaced points around the edge of the pool were designed as N (North), E (East), S (South) and W (West). A black-colored round platform of 8 cm diameter was placed 1 cm below the surface of water in a constant position in the middle of the NE quadrant in the pool. The water was colored with nontoxic black dye to hide the location of the submerged platform. The animal was released into the pool from the SW quadrant in all the trials. The mice were given a maximum time of 60 s (cut-off time) to find the hidden platform and were allowed to stay on it for 30 s. The time taken for the mouse to find the escape platform was measured by the video tracking system. In the event the animal was unable to locate the hidden platform within 60 s, it was gently guided to it. Each animal was subjected to a daily session of three trials per day (with a rest period of 30–45 min (in a small heated cage in a different room) inter trial periods) for five consecutive days. Escape latency time (ELT) to locate the hidden platform in water maze was noted as an index of learning. Mean ELT of all three trials is shown in the results. Note a significant decrease in latency time from that of first session indicates learning in water maze test (Tota et al., Citation2011).
Spontaneous locomotor activity
On day 2, 60 min post-methanol root extract and CF-2 treatment, animals were placed in Optovarimex activity meter (Columbus, OH) and locomotor activity was monitored for 10 min taking readings at every 2 min. Results were expressed as mean counts/2 min.
Estimation of biochemical parameters
AChE and biochemical parameters of oxidative stress, MDA, glutathione (GSH) and nitrite, were measured in the brain of animals after the completion of behavioral studies.
Brain tissue preparation
The mice were decapitated under ether anesthesia. The skull was cut open and the brain was exposed from its dorsal side. The whole brain was quickly removed and cleaned with chilled normal saline on ice. A 10% (w/v) homogenate of brain samples (0.03 M sodium phosphate buffer, pH 7.4) was prepared by using an Ultra-Turrax T25 (Groton, CT) homogenizer at a speed of 9500 rpm.
Acetylcholinesterase assay in brain
The brain homogenate in volume of 500 μl was mixed with 1% Triton X-100 (1% w/v in 0.03 M sodium phosphate buffer, pH 7) and centrifuged at 100,000 g at 4 °C in a Beckman Ultracentrifuge LE 80 (Beckman Coulter, Carlsbad, CA), using a fixed angle rotor (80 ti) for 60 min. The supernatant was collected and stored at 4 °C for AChE estimation. The kinetic profile of enzyme activity was measured spectrophotometrically (Shimadzu, OR) at 412 nm with an interval of 15 s. The specific activity of AChE is expressed in micromoles per minute per milligram of protein (Awasthi et al., Citation2010; Tota et al., Citation2010, Citation2011).
Measurement of MDA
MDA (nmol/mg protein), which is a measure of lipid peroxidation, was measured spectrophotometrically by the method of Colado et al. (Citation1997), using 1,1,3,3-tetraethoxypropane as standard. To 500 µl of tissue homogenate in phosphate buffer (pH 7.4), 300 µl of 30% TCA, 150 µl of 5% N HCl and 300 µl of 2% w/v 2-thiobarbituric acid (TBA) were added and then the mixture was heated for 15 min at 90 °C. The mixture was centrifuged at 12,000 g for 10 min. Pink-colored supernatant was obtained, which was measured spectrophotometrically at 532 nm.
Measurement of GSH
GSH (µg/mg protein) was determined by its reaction with 5,5′-dithiobis (2-nitrobenzoic acid) to yield a yellow chromophore which was measured spectrophotometrically (Ellman, Citation1959). The brain homogenate was mixed with an equal amount of 10% TCA and centrifuged (Remi cold centrifuge) at 2000 g for 10 min at 4 °C. The supernatant was used for GSH estimation. To 0.1 ml of processed tissue sample, 2 ml of phosphate buffer (pH 8.4), 0.5 ml of 5,5′-dithiobis (2-nitrobenzoic acid) (DTNB) and 0.4 ml of double-distilled water were added and the mixture was shaken vigorously on vortex. The absorbance was read at 412 nm within 15 min.
Nitrite estimation
Nitrite was estimated in the mice brain using the Greiss reagent and served as an indicator of nitric oxide production. One hundred microliter of Greiss reagent (1:1 solution of 1% sulphanilamide in 5% phosphoric acid and 0.1% napthaylamine diamine dihydrochloric acid in water) was added to 100 μl of the supernatant and absorbance was measured at 542 nm (Green et al., Citation1982). Nitrite concentration was calculated using a standard curve for sodium nitrite. Nitrite levels were expressed as microgram per milligram protein.
Protein estimation
Protein was measured in all brain samples for MDA, GSH and nitrite by the method of Lowry et al. (Citation1951) and for AChE activity by the method of Wang and Smith (Citation1975). BSA (1 mg/ml) was used as a standard and measured in the range of 0.01–0.1 mg/ml.
Statistical analysis
The results are expressed as mean ± S.E.M. The statistical analysis of passive avoidance data was done by Student’s t-test. The Morris water maze and biochemical values were analyzed by the one-way analysis of variance (ANOVA) followed by the Tukey post hoc multiple comparison test (p < 0.05).
Result
Effect of C. ferruginea and amentoflavone on scopolamine-induced memory impairment in passive avoidance test
Control mice showed clear retention as indicated by the significant increase (p < 0.05) in TLT of retention trial in comparison to acquisition trial while administration of scopolamine (i.p.) 5 min before acquisition trial caused no significant change in TLT of retention trial when compared to the acquisition trial (). However, CF (25, 50, 100 and 200 mg/kg, p.o.) had no significant effect (p > 0.05) on TLT in acquisition trial but caused a significant increase (p < 0.05) in TLT in the retention test in a dose-dependent manner suggesting antagonism of scopolamine-induced amnesia. Similar effect was observed with tacrine (). Oral administration of CF-2 (6.25, 12.5 and 25 mg/kg, p.o.) for three days dose-dependently ameliorated the scopolamine-induced amnesia in mice. CF-2 exerted maximum effect at a dose of 12.5 mg/kg at which the retention latencies were comparable to the control group. There was no significant difference (p > 0.05) in the retention latencies of the extracts and tacrine-treated groups ( and ).
Effect of C. ferruginea and amentoflavone on scopolamine-induced memory impairment in Morris water maze
Saline-treated mice rapidly acquired the spatial task as indicated by a gradual, session-dependent decrease in ELT [F(4, 29) = 46.79, p < 0.001] and path length [F(4, 29) = 19.28, p < 0.01]. Administration of scopolamine (3 mg/kg, i.p.) 5 min before water maze trial caused spatial memory impairment as indicated by no significant change [F(4, 29) = 1.86, p > 0.05] in ELT of session 2–5 in comparison to that of session 1. Further, there was no significant change [F(4, 29) = 0.34, p > 0.05] in the path length of the scopolamine-pretreated group. Treatment with tacrine (5 mg/kg, i.p.) followed by scopolamine produced a significant decrease [F(4, 29) = 10.59, p < 0.05] in ELT and path length [F(4, 29) = 7.3, p < 0.05] from third session onward when compared to the first session. Oral administration of CF showed dose-dependent effect on scopolamine-induced memory deficit in mice. As shown in , CF 100 mg/kg significantly decreased mean ELT from fourth session [F(4, 29) = 9.35, p < 0.05] when compared to first session, while 200 mg/kg CF reduced the mean ELT significantly from second session onward [F (4, 29) = 17.26, p < 0.001]. There was a comparable decrease in ELT in CF- and tacrine-treated groups.
Figure 3. Effect of methanolic root extract of CF on scopolamine-induced amnesia in Morris water maze test. Data are expressed as mean ELT (sec) ± S.E.M. Significant decrease (*p < 0.05, **p < 0.01 and ***p < 0.001) versus session 1.
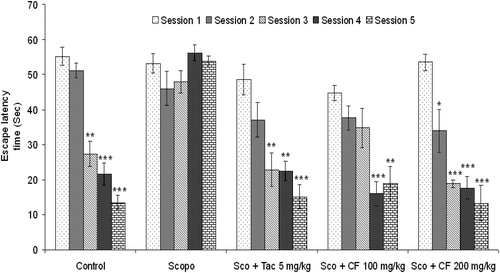
Investigation of path length revealed a significant decrease in the path length in the CF-treated group [100 mg/kg: F(4, 29) = 10.3, p < 0.01; 200 mg/kg: F(4, 29) = 20.78, p < 0.001] (). Further, statistical analysis showed a significant correlation (Pearson r = 0.986; R2 = 0.971 and p < 0.05) between mean latency time and mean path length of all the groups in all sessions (). The representative swimming pattern of mice of different groups is shown in .
Figure 4. Effect of methanolic root extract of CF on mean distance traveled (path length) in Morris water maze test. Data are expressed as mean path length (cm) ± S.E.M. Significant decrease (*p < 0.05, **p < 0.01 and ***p < 0.001) versus session 1.
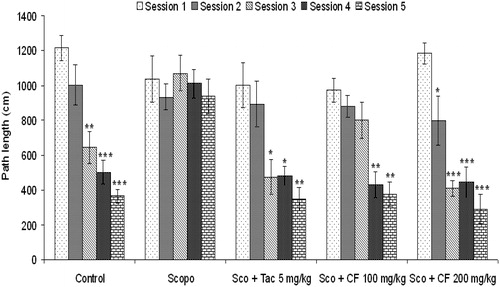
Figure 6. Representative Morris water maze tracing of different groups of animals during last trial of session 5.

Further, CF-2 also prevented scopolamine-induced memory deficit in mice dose-dependently. As shown in , CF-2 significantly reduced mean ELT from second session onward [12.5 mg/kg: F(4, 29) = 16.73, p < 0.001]; 25 mg/kg: [F(4, 29) = 27.57, p < 0.001]. In addition, investigation of path length showed that the CF-2 treated group had significant reduction in path length when compared to session 1 (). There was a significant correlation (Pearson r = 0.985; R2 = 0.969 and p < 0.01) between ELT and the mean path length of all the treated groups in all the sessions (). The representative swimming pattern of mice of different groups is shown in .
Figure 7. Effect of CF-2 on scopolamine-induced amnesia in Morris water maze test. Data are expressed as mean ELT (sec) ± S.E.M. Significant decrease (**p < 0.01 and ***p < 0.001) versus acquisition trial.

Spontaneous motor activity
There were no significant differences between treatments groups (p > 0.05) in comparison to control (data not shown).
Estimation of biochemical parameters
Acetylcholinesterase activity
AChE activity (µmol/min/mg protein) was estimated on day 5 after the first dose of scopolamine. There was significant increase (p < 0.05) in AChE activity in scopolamine-treated group when compared to the control group. The AChE activity was significantly decreased in CF-treated groups (100 and 200 mg/kg) when compared to the scopolamine group [F(2, 15) = 27.39, p < 0.01].
Similarly, CF-2 (12.5 and 25 mg/kg) significantly reduced AChE activity at both doses [F(2, 15) = 91.56, p < 0.01] in comparison to the scopolamine group. Tacrine also significantly reduced (p < 0.05) AChE activity in mice brain with comparable effect relative to CF and CF-2 ().
Estimation of parameters of oxidative stress in the brain
Malondialdehyde level
The MDA level (nmol/mg protein) in the brain was measured after the completion of behavioral tests. The MDA level was significantly higher (p < 0.05) in scopolamine-treated mice as compared to control, while in the tacrine-treated group a significant reduction in the level of MDA was observed. CF (100 and 200 mg/kg) ameliorated scopolamine-induced lipid peroxidation as shown by significantly reduced MDA level in mice brain [F(2, 15) = 47.12, p < 0.01] (). Similarly, CF-2 showed significant [F(2, 15) = 37.56, p < 0.01] antioxidant effect in scopolamine-injected mice as indicated by significant decrease in the MDA level ().
GSH estimation
A significant reduction (p < 0.05) in the levels of GSH was observed in the scopolamine group as compared to the control. The GSH level in CF-treated (100 and 200 mg/kg) mouse brain was significantly higher [F(2, 15) = 24.63, p < 0.01] in comparison to scopolamine group indicating amelioration of scopolamine-induced oxidative stress (). The active constituents of CF and CF-2 also prevented scopolamine-induced reduction in the GSH level [F(2, 15) = 29.68, p < 0.01] in mouse brain (). The standard drug tacrine also reversed the effect of scopolamine on the GSH level.
Nitrite levels
As shown in , there was no significant change (p > 0.05) in nitrite level in the brain of scopolamine-treated mice in comparison to control. Further, CF [F(2, 15) = 1.2, p > 0.05], CF-2 [F(2, 15) = 1.66, p > 0.05] did not affect the nitrite level in mice brain.
Discussion
The central cholinergic system plays a major role in the process of learning and memory and has been carefully articulated over the last several decades. Whitehouse et al. (Citation1982) reported that cholinergic cell bodies are destroyed by the disease process in AD patients, leading to a deficiency of the neurotransmitter ACh. On the other hand, several studies have shown that blockade of ACh effects by muscarinic receptor antagonist scopolamine caused memory deficits in both normal (Drachman & Leavitt, Citation1974) and elderly groups (Sunderland et al., Citation1987). These observations led to the experimental strategies for antiamnesic studies that use the scopolamine-induced amnesia model, which also has become the common manipulation for antiamnesic drug evaluations.
The cognitive-enhancing activity of C. ferruginea and amentoflavone against scopolamine-induced memory impairments in mice was investigated using the passive avoidance test, the Morris water maze test and biochemical assessments. The passive avoidance test is generally used to evaluate the treatments on the three stages of memory, such as learning acquisition, memory retention and the retrieval process (Lorenzini et al., Citation1996). In this test, it was shown that scopolamine significantly reduced the step-through latency of the retention trial, demonstrating that the central cholinergic neuronal system plays an important role in learning acquisition. Cnestis ferruginea and amentoflavone prolonged the step-through latency reduced by scopolamine, indicating the facilitating effect of the extract on cognitive functions in the acquisition and retrieval stages of learning and memory. These results suggest that the antiamnesic effects of C. ferruginea and amentoflavone against scopolamine-induced memory impairment may be related to mediation of the cholinergic nervous system.
In order to confirm the effects of C. ferruginea and amentoflavone on spatial memory, the Morris water maze test on spatial learning was carried out. Mice treated with scopolamine showed more prolonged ELT than mice from the control group. Preventive treatment with C. ferruginea and amentoflavone for three days ameliorated scopolamine-induced amnesia in mice as indicated by significant decrease in ELT in the Morris water maze test. Similarly, C. ferruginea and amentoflavone produced significant reduction in the path length (distance traveled) in the Morris water maze test. There was a significant correlation between mean path length and mean ELT of all the groups in all the sessions. This indicates that scopolamine and/or drug administration did not alter the swimming speed or motor performance of the animals. Further, there was no significant difference in locomotor activity among different groups excluding possibility that the disturbances in the motor function may have contributed to the performance of the animals in behavioral tests. An accurate direction of the swimming behavior provides evidence that the mice have learned the spatial location of the platform relative to the available external cues. Therefore, these results suggest that C. ferruginea and amentoflavone can repair the long-term memory in scopolamine-induced memory impairments.
To elucidate the underlying mechanisms of memory-enhancing effects of C. ferruginea and amentoflavone, activity of AChE as cholinergic marker was assessed using brain homogenates. Neurochemical studies suggested that the cholinergic system plays an important role in learning and memory (Blokland, Citation1995). In an in vivo study as a model of dementia treated with scopolamine, cholinergic neurotransmission was obstructed leading to an increase of the AChE and impaired cognition (Levey, Citation1996). According to the cholinergic hypothesis, memory impairments in patients with senile dementia are due to a selective and irreversible deficiency in the cholinergic functions in the brain (Giacobini, Citation2002). Therefore, cholinesterase inhibitors may compensate for reduced ACh levels in brains with AD disease. In this study, C. ferruginea and amentoflavone treatment significantly inhibited AChE activity.
Thus, it could be explained that the antiamnesic effect of C. ferruginea and amentoflavone on scopolamine-induced impairment of learning and memory may be related to modification of cholinergic neuronal systems. This study further evaluated whether such impaired cognition by scopolamine is associated with altered oxidative stress indices. Scopolamine-treated mice had elevated MDA and nitrite levels with reduced GSH activity. Increased MDA levels have been shown to be an important marker for in vivo lipid peroxidation. Oxidative stress results from a marked imbalance between free radical production and elimination by antioxidant systems. Recently, many studies have reported the strong positive correlation that memory impairments in the scopolamine-induced amnesic mice show with patterns of oxidative damage in patients with amnesic mild cognitive impairment (El-Sherbiny et al., Citation2003; Fan et al., Citation2005). Moreover, many clinical studies have reported that oxidative stress is closely involved in the pathogenesis of AD (Marcus et al., Citation1998). GSH is the principal intracellular nonprotein thiol and plays a major role in the maintenance of the intracellular redox state. The level of GSH diminishes with an increase in the generation of free radicals (Dringen, Citation2000). In the scopolamine model of dementia, MDA and GSH were estimated on the fifth day after the first injection of scopolamine. Scopolamine-treated mice showed a significant increase in MDA and decrease in the GSH level in the brain compared to control values, indicating elevated oxidative stress. However, C. ferruginea and amentoflavone did not affect the scopolamine-induced nitrite elevation.
The administration of C. ferruginea and amentoflavone produced significant reduction in MDA level, and restored the activities of GSH in mice brain. These observations suggest that C. ferruginea and amentoflavone produced significant antioxidant activity against scopolamine-induced oxidative stress. We suggest that the restoration of the activities of GSH by C. ferruginea and amentoflavone might promote scavenging of free radicals by GSH. The potent antiamnesic effect of C. ferruginea and amentoflavone might result, in part, from the reduction in oxidative stress and more importantly, inhibition of AChE activity which is the cornerstone of AD management (Citron, Citation2010).
The findings in this study showed that C. ferruginea and amentoflavone are potent neuroprotective agents which could be linked to the inhibition of both AChE activity and MDA generation in the brain of mice with scopolamine-induced amnesia. The antiAChE, antioxidant and antiamnesic effects of C. ferruginea and amentoflavone on scopolamine-induced cognitive impairments ex vivo suggest that they may be viable candidates for the treatment of neurodegenerative diseases, like AD.
Declaration of interest
The authors have declared that there is no conflict of interest.
Acknowledgements
We sincerely acknowledge TWAS and CSIR for the postgraduate Fellowship given to Ishola Ismail O. Also, the provision of research facilities by Central Drug Research Institute (CDRI), India, and Sophisticated Analytical Instrument Facility (SAIF) for spectral data are highly appreciated.
References
- Agrawal R, Tyagi E, Saxena G, Nath C. (2009). Cholinergic influence on memory stages: A study on scopolamine amnesic mice. Indian J Pharmacol 41:192–6
- Alzheimer’s Association (2006). Early-Onset Dementia: A National Challenge, a Future Crisis. Washington, DC: Alzheimer’s Association
- Alzheimer’s Association (2012). 2012 Alzheimer’s disease, facts and figures. Available from: www.alz.org/downloads/facts_figures_2012.pdf [last accessed 18 Jun 2012]
- Awasthi H, Tota S, Hanif K, et al. (2010). Protective effect of curcumin against intracerebral streptozotocin induced impairment in memory and cerebral blood flow. Life Sci 86:87–94
- Banerjee T, Valacchi G, Ziboh VA, van der Vliet A. (2002). Inhibition of TNF-α-induced cyclooxygenase-2 expression by amentoflavone through suppression of NF-κB activation in A549 cells. Mol Cell Biochem 238:105–10
- Beatty WW, Butters N, Janowsky DS. (1986). Patterns of memory failure after scopolamine treatment: Implications for cholinergic hypotheses of dementia. Behav Neural Biol 45:196–211
- Blokland A. (1995). Acetylcholine: A neurotransmitter for learning and memory? Brain Res Rev 21:285–300
- Bores GM, Huger FP, Petko W, et al. (1996). Pharmacological evaluation of novel Alzheimer’s disease therapeutics: Acetylcholinesterase inhibitors related to galanthamine. J Pharmacol Exp Ther 277:728–38
- Citron M. (2010). Alzheimer’s disease: Strategies for disease modification. Nat Rev Drug Discov 9:387–98
- Colado MI, O’Shea E, Granados R, et al. (1997). A study of the neurotoxic effect of MDMA (‘ecstasy’) on 5-HT neurones in the brains of mothers and neonates following administration of the drug during pregnancy. Br J Pharmacol 121:827–33
- Drachman DA, Leavitt J. (1974). Human memory and the cholinergic system: A relationship to aging? Arch Neurol 30:113–21
- Dringen R. (2000). Glutathione metabolism and oxidative stress in neurodegeneration. Eur J Biochem 267:4903
- Ellman GL. (1959). Tissue sulfhydryl groups. Arch Biochem Biophys 82:70–7
- El-Sherbiny DA, Khalifa AE, Attia AS, Eldenshary EES. (2003). Hypericum perforatum extract demonstrates antioxidant properties against elevated rat brain oxidative status induced by amnesic dose of scopolamine. Pharmacol Biochem Behav 76:523–33
- Fan Y, Hu J, Li J, et al. (2005). Effect of acidic oligosaccharide sugar chain on scopolamine-induced memory impairment in rats and its related mechanisms. Neurosci Lett 374:222–6
- Francis PT, Palmer AM, Snape M, Wilcock GK. (1999). The cholinergic hypothesis of Alzheimer's disease: A review of progress. J Neurol Neurosurg Psychiatry 66:137–47
- Giacobini E. (2002). Long-term stabilizing effect of cholinesterase inhibitors in the therapy of Alzheimer’s disease. J Neural Transm Suppl 62:181–7
- Gilgun-Sherki Y, Melamed E, Offen D. (2002). Antioxidant treatment in Alzheimer’s disease: Current state. J Mol Neurosci 21:1–11
- Green LC, Wagner DA, Glogowski J, et al. (1982). Analysis of nitrate, nitrite, and [15N] nitrate in biological fluids. Anal Biochem 126:131–8
- Guan ZZ, Zhang X, Ravid R, Nordberg A. (2000). Decreased protein levels of nicotinic receptor subunits in the hippocampus and temporal cortex of patients with Alzheimer’s disease. J Neurochem 74:237–43
- Hebert LE, Scherr PA, Bienias JL, et al. (2003). Alzheimer disease in the U.S. population: Prevalence estimates using the 2000 Census. Arch Neurol 60:1119–22
- Hyman BT, Damasio H, Damasio AR, Van Hoesen GW. (1989). Alzheimer’s disease. Ann Rev Public Health 10:115–40
- Ishola IO, Agbaje OE, Narender T, et al. (2012). Bioactivity guided isolation of analgesic and anti-inflammatory constituents of Cnestis ferruginea Vahl ex DC (Connaraceae) root. J Ethnopharmacol 142:383–9
- Ishola IO, Akindele AJ, Adeyemi OO. (2011). Analgesic and anti-inflammatory activities of Cnestis ferruginea Vahl ex DC (Connaraceae) methanol root extract. J Ethnopharmacol 135:55–62
- Ishola IO, Ashorobi RB. (2007). Anti-stress potential of aqueous root extract of Cnestis ferruginea. Int J Pharmacol 3:295–8
- Jeong EJ, Lee KY, Kim SH, et al. (2008). Cognitive-enhancing and antioxidant activities of iridoid glycosides from Scrophularia buergeriana in scopolamine treated mice. Eur J Pharmacol 588:78–84
- Kang SS, Lee JY, Choi YK, et al. (2004). Neuroprotective effects of flavones on hydrogen peroxide-induced apoptosis in SH-SY5Y neuroblastoma cells. Bioorg Med Chem Lett 14:2261–4
- Kang SS, Lee JY, Choi YK, et al. (2005). Neuroprotective effects of naturally occurring biflavonoids. Bioorg Med Chem Lett 15:3588–91
- Kopelman MD, Corn TH. (1988). Cholinergic ‘blockade’ as a model for cholinergic depletion. A comparison of the memory deficits with those of Alzheimer-type dementia and the alcoholic Korsakoff syndrome. Brain 111:1079–110
- Kulkarni KS, Kasture SB, Mengi SA. (2011). Efficacy study of Prunus amygdalus (almond) nuts in scopolamine-induced amnesia in rats. Indian J Pharmacol 42:168–73
- Levey AI. (1996). Muscarinic acetylcholine receptor expression in memory circuits: Implications for treatment of Alzheimer disease. Proc Natl Acad Sci USA 93:13541–6
- Lorenzini CA, Baldi E, Bucherelli C, et al. (1996). Role of dorsal hippocampus in acquisition, consolidation and retrieval of rat’s passive avoidance response: A tetrodotoxin functional inactivation study. Brain Res 730:32–9
- Lowry OH, Rosebrough NJ, Farr AL, Randall RJ. (1951). Protein measurement with the Folin phenol reagent. J Biol Chem 193:265–75
- Marcus DL, Thomas C, Rodriquez C, et al. (1998). Increased peroxidation and reduced antioxidant enzyme activity in Alzheimer’s disease. Exp Neurol 150:40–4
- Markham KR, Sheppard C, Geiger H. (1987). 13C NMR studies on some naturally occurring amentoflavone and hinokiflavone biflavonoids. Phytochemistry 26:3335–7
- Oke JM, Hamburger MO. (2002). Screening of some Nigerian medicinal plants for antioxidant activity using 2,2,diphenyl-picryl-hydrazyl radical. Afr J Biomed Res 5:77–9
- Riedel W, Hogervorst E, Leboux R, et al. (1995). Caffeine attenuates scopolamine-induced memory impairment in humans. Psychopharmacol (Berl) 122:158–68
- Sharma D, Puri M, Tiwary AK, et al. (2010). Antiamnesic effect of stevioside in scopolamine-treated rats. Indian J Pharmacol 42:164–7
- Sunderland T, Tariot PN, Cohen RM, et al. (1987). Anticholinergic sensitivity in patients with dementia of the Alzheimer type and age-matched controls: A dose-response study. Arch Gen Psychiatry 44:418–26
- Taffe MA, Weed MR, Gold LH. (1999). Scopolamine alters rhesus monkey performance on a novel neuropsychological test battery. Brain Res Cogn Brain Res 8:203–12
- Tota S, Awasthi H, Kamat PK, et al. (2010). Protective effect of quercetin against intracerebral streptozotocin induced reduction in cerebral blood flow and impairment of memory in mice. Behav Brain Res 209:73–9
- Tota S, Kamat PK, Awasthi H, et al. (2009). Candesartan improves memory decline in mice: Involvement of AT1 receptors in memory deficit induced by intracerebral streptozotocin. Behav Brain Res 199:235–40
- Tota S, Kamat PK, Shukla R, Nath C. (2011). Improvement of brain energy metabolism and cholinergic functions contributes to the beneficial effects of silibinin against streptozotocin induced memory impairment. Behav Brain Res 221:207–15
- Wang C, Smith RL. (1975). Lowry determination of protein in the presence of Triton X-100. Anal Biochem 63:414–17
- Whitehouse PJ, Price DL, Struble RG, et al. (1982). Alzheimer’s disease and senile dementia: Loss of neurons in the basal forebrain. Science 215:1237–39
- WHO (2006). Traditional medicine. Available from http://www.who.int/mediacentre/factsheets/fs134/en/ [last accessed on 29 Oct 2011]
- Youdim KA, Spencer JP, Schroeter H, Rice-Evans C. (2002). Dietary flavonoids as potential neuroprotectants. Biol Chem 383:503–19
- Zhang ZJ. (2004). Therapeutic effects of herbal extracts and constituents in animal models of psychiatric disorders. Life Sci 75:1659–99