Abstract
Context: Recently, there has been renewed interest in barley (Hordeum vulgare L. Poaceae) as a functional food and for its medicinal properties.
Objective: This study examines the anti-inflammatory potential of the active fractions of barley and the mechanisms involved.
Materials and methods: The macrophages were exposed to 100 μg/mL of each of the barley extracts in the presence of 1 μg/mL lipopolysaccharide (LPS) and after 24 or 48 h of incubation, cells or culture supernatants were analyzed by various assays. The anti-inflammatory potential of barley fractions was also investigated using the LPS-injected septic mouse model. The active constituents in the fractions were identified using gas chromatography-mass spectrometry (GC-MS).
Results: The active fractions, named F4, F7, F9 and F12, inhibited almost completely the LPS-induced production of nitric oxide (NO) and inducible NO synthase. Pre-treatment with these fractions at 100 μg/mL diminished the tumor necrosis factor-α (TNF-α) levels to 19.8, 3.5, 1.2 and 1.7 ng/mL, respectively, compared to LPS treatment alone (41.5 ng/mL). These fractions at 100 μg/mL also suppressed apparently the secretion of interleukin (IL)-6 and IL-1β and the DNA-binding activity of nuclear factor-κB in LPS-stimulated cells. Mice injected intraperitoneally with LPS (30 mg/kg BW) showed 20% survival at 48 h after injection, whereas oral administration of the fractions improved the survival rates to 80%. GC-MS analysis revealed the presence of the derivatives of benzoic and cinnamic acids and fatty acids in the fractions.
Discussion and conclusion: The aerial parts of barley are useful as functional food to prevent acute inflammatory responses.
Introduction
Inflammation is a complex immune response to harmful stimuli including various pathogens, damaged cells and/or irritants, and is closely associated with the progression of chronic disease (Nakagawa & Maeda, Citation2012). While, macrophages are activated by various inflammatory mediators including nitric oxide (NO), prostaglandins (PGs) and pro-inflammatory cytokines, the activated macrophages also produce these mediators. In particular, macrophages play critical roles in producing interferon-γ (IFN-γ), interleukin (IL)-1β, IL-6 and the tumor necrosis factor-α (TNF-α), all of which are important inflammation mediators (Sung & Wong, Citation2007; Vuolteenaho et al., Citation2007).
The primary endotoxin, lipopolysaccharide (LPS), elicits immune responses through the activation of macrophages in order to produce a variety of inflammatory mediators (Hsieh et al., Citation2011). LPS stimulates the activation of nuclear factor-κB (NF-κB), which is an essential transcription factor for host defense in response to cellular stress and inflammation. The activation of NF-κB induces the production of inducible nitric oxide synthase (iNOS) and cyclooxygenase-2 (COX-2) as well as various pro-inflammatory cytokines (Kang et al., Citation2009). Therefore, chronic and/or persistent activation of this transcription factor and/or its dysregulation causes various pathologic conditions including cancer, septic shock, rheumatoid arthritis and autoimmune diabetes (Hesslinger et al., Citation2009; Kanwar et al., Citation2009; Kern et al., Citation2007).
There is increased interest in the application of dietary components in order to prevent inflammatory damage and/or to control immune response induction. Barley is a major cereal grain and has been cultivated for a long time for use as food and feed. Various studies have shown that barley leaves possess certain bioactive chemicals that are beneficial to health, including potential antiulcer, antioxidant, hypolipidemic, antidepressant and antidiabetic properties (Kamiyama & Shibamoto, Citation2012; Yamaura et al., Citation2012; Yu et al., 2002a). Barley is also known to contain an abundance of nutritional compounds and various secondary metabolites including flavonoids, phenolics and other nutrients (Quinde-Axtell & Baik, Citation2006). Therefore, renewed interest has been gained in barley as a functional food and for its medicinal properties. However, little information exists on its anti-inflammatory potential. Although β-glucans derived from barley are thought to be anti-inflammatory bioactive materials, their activities differ from their heterogeneity (Chanput et al., Citation2012). In addition, the effects of the aerial parts of barley on immune modulation have not been studied extensively.
In this study, we isolated active fractions from the methanol extract of the aerial parts of barley and investigated their potential to prevent inflammatory responses stimulated by LPS using in vitro and in vivo systems. We tried to identify the active constituents responsible for the anti-inflammatory action through gas chromatography-mass spectrometry (GC-MS) analysis.
Materials and methods
Mice, chemicals and plastics
Female BALB/c mice (≥20 g; Orient Co., Seoul, Korea) were housed in automatically controlled conditions with a 12 h light/dark cycle at 22 ± 1 °C and 45–55% relative humidity. All of the mice had free access to standard rodent food pellets and water. This study was approved by the Laboratory Animal Center (CBU 2012-0039) and all the experiments were carried out according to the guidelines of the Animal Care and Use Committee of Chonbuk National University. Unless otherwise specified, all the chemicals used in this study were purchased from Sigma Chemical Co. (St. Louis, MO) and all the plastics were purchased from Falcon Labware (Becton-Dickinson, Franklin Lakes, NJ).
Extraction and fractionation of barley methanol extracts
The dried powder of the aerial parts of barley was obtained from the Grassland and Forages Research Center (GFRC) at the National Institute of Animal Science (South Korea). The barley (Hordeum vulgare L.) was cultivated at the experimental farm of GFRC in 2009 and harvested at the yellow ripe stage. Barley and its aerial parts were authenticated by botanists at the National Institute of Animal Science. The extraction and fractionation of barley extracts were performed according to the methods described elsewhere (Fang et al., Citation2011) with slight modification. Initially, methanol crude extract was prepared from the dried aerial parts of the barley by shaking 500 g of the powder in 2000 mL of 70% methanol at room temperature for 24 h twice. The methanol extract was concentrated using a rotary vacuum evaporator (Rotavapor R110, Büchi, Flawil, Switzerland) and lyophilized to create a crude powder (42.6 g). The extract was serially eluted with 1000 mL of the following solvents stepwise twice: petroleum ether, chloroform, n-butanol and distilled water. The serial extracts were concentrated and lyophilized to create various amounts of the extracts as shown in . Based on the inhibitory activities of the LPS-induced inflammatory responses, the chloroform soluble extract (CSE) was further separated into 16 sub-fractions (F1–F16) by chromatography using a Sephadex LH-20 column. In brief, 100 g of Sephadex LH-20 resin (LH20100-100G, Sigma-Aldrich, St. Louis, MO) was hydrated in deionized water overnight at 4 °C and packed into a glass column (370 × 30 mm). The CSE (2.62 g) was loaded onto the top of a LH-20 cartridge and sequentially eluted with H2O (1/3 of the bed volume) to obtain one fraction (F1). Then, the CSE was serially fractionized with 2× (v/v) of the bed volume of 10%, 30%, 50%, 70% and 100% methanol in order to obtain a total of 15 fractions (F2–F16; three fractions per each methanol gradient elution). The organic solvent of each fraction was evaporated and then lyophilized until it reached dryness. These fractionized samples were processed for the analysis of the mechanisms involved in anti-inflammation. Four fractions (i.e., F4, F7, F9 and F12) were further analyzed in order to determine their anti-inflammatory potential and the related mechanisms.
Cell culture and treatment
RAW 264.7 macrophages were cultured in Dulbecco’s modified Eagle’s medium (DMEM) supplemented with 10% fetal bovine serum (FBS; HyClone, Logan, UT), 2 mM l-glutamine, and antibiotics. Cells (1 × 106/mL) in 2 mL or 200 μL media were seeded in 6- or 96-well flat-bottomed plates, respectively. When the cells had reached between 70 and 80% confluence, they were exposed to 100 μg/mL of each of the barley extracts in the presence and absence of 1 μg/mL LPS. Several cancer cell lines, including YD-38 human oral epithelial cancer cells, Hs 63.T human skin dermatofibrosarcoma protuberans and HepG2 human hepatoma cells, were also cultured in DMEM containing 10% FBS. These cells were used in order to determine the anti-tumor activity of the barley extracts in vitro. In addition, human gingival fibroblasts (HGF) were cultured according to previously described methods (Kook et al., Citation2009) and the primary cultures were used as a control for these cancer cells.
Measurement of NO and pro-inflammatory cytokines in cultures
The nitrite concentration in the culture medium of cells stimulated with 1 μg/mL LPS in the presence and absence of barley samples was measured as an indicator of the NO production using the Griess reagent as described previously (Fang et al., Citation2011). The levels of pro-inflammatory cytokines (IL-6, IL-1β and TNF-α) in the culture supernatants of these cells were also determined using mouse quantikine® ELISA kits (R&D systems, Minneapolis, MN) according to manufacturer instructions.
Western blot analysis
Preparation of whole protein lysates and quantification of the protein content in each sample were performed according to a method described elsewhere (Son et al., Citation2009). Equal protein amounts of each sample were separated using 12% SDS–PAGE and blotted onto PVDF membranes. The blots were probed with primary and secondary antibodies and then developed with enhanced chemiluminescence (Amersham Pharmacia Biotech, Buckinghamshire, UK) prior to exposure to X-ray film (Eastman-Kodak, Rochester, NY). Antibodies specific to iNOS and β-actin were obtained from Santa Cruz Biotechnology (Santa Cruz, CA).
Assays for NF-κB DNA-binding activity
The NF-κB binding activity in regards to its specific DNA sequence was determined using nuclear protein extracts from LPS-stimulated RAW264.7 cells with and without barley fractions using an electrophoretic mobility shift assay (EMSA). This assay was carried out according to the previously described method (Fang et al., Citation2011). In brief, the DNA–protein binding reactions were performed for 30 min at room temperature with 10–15 μg protein in a 20 μL buffer containing 1 μg/mL BSA, 0.5 μg/μL poly (dI-dC), 5% glycerol, 1 mM DTT, 1 mM PMSF, 10 mM Tris-Cl (pH 7.5), 50 mM NaCl, 30 000 cpm of [α-32P] dCTP-labeled oligonucleotides and the Klenow fragment of DNA polymerase. The samples were separated on 6% polyacrylamide gels and the dried gels were exposed to X-ray films (Eastman Kodak Co.) for 12 to 24 h at −70 °C. The oligonucleotide primer sequences specific to NF-κB were: 5′-AAG GCC TGT GCT CCG GGA CTT TCC CTG GCC TGG A-3′ and 3′-GGA CAC GAG GCC CTG AAA GGG ACC GGA CCT GGA A-5′. In addition, the DNA-binding activity of the NF-κB p65 subunit in the same nuclear protein extracts was examined using a TransAM® NF-κB p65 ELISA kit (Active Motif, Carlsbad, CA) according to manufacturer instructions. The peroxidase activity was determined by measuring the absorbance at 450 nm using a SpectraCountTM ELISA reader (Packard Instrument Co., Downers Grove, IL).
Survival assay
Female BALB/c mice were randomly divided into several groups of five mice each and caged separately before treatment with the barley extract and/or LPS. The mice were orally administered either 200 μL PBS alone or 200 μL PBS containing each of the barley fractions (F4, F7, F9 and F12; 30 mg/kg body weight; BW) every other day for 10 d. The oral concentration of these fractions was derived from their dose to suppress effectively LPS-induced production of inflammatory mediators. The mice received an intraperitoneal injection of LPS (30 mg/kg BW) 1 d after the last administration of the barley fractions and their survival was recorded every 6 h after the LPS injection for 4 d.
Assay for cytokine levels in serum
The serum levels of TNF-α, IL-1β and IL-6 in a murine sepsis BALB/c model were measured. The sera from the mice injected with LPS alone or pre-treated with barley fractions were briefly collected from five mice per group by retro-orbital bleeding at each time point of 2 and 8 h after the LPS injection. The levels of these cytokines were measured as described above.
GC-MS analysis
The four fractions (i.e., F4, F7, F9 and F12) were processed for GC-MS analysis. Briefly, The GC-MS was performed using an Agilent HP 6890 gas chromatography instrument coupled with an Agilent HP 5973 mass spectrometer and Agilent ChemStation software (Agilent Technologies, Palo Alto, CA). The compounds were separated on a DB-5 ms, 12 m × 0.20 mm i.d. capillary column coated with a 0.33 μm film. The oven temperature was 70 °C, which was maintained for 3 min, followed by an increase in temperature to 300 °C, which was held isothermally for 31 min. Helium was used as carrier gas at a 1.0 mL min−1 flow rate. The injector and detector temperatures were 70 and 280 °C, respectively. The parameters of the HP 5973 mass detector were: ion mass/charge ratio of 20–500 m/z; scan mode; and ionization energy of 70 eV.
Statistical analyses
Data are expressed as mean ± standard deviation (SD) from three different samples. Unless otherwise specified, all the experiments were performed in triplicate. A one-way analysis of the variance (SPSS version 12.0 software, Chicago, IL) followed by Scheffe’s test was applied in order to determine significant differences among the groups. A p value <0.05 was considered significant.
Results
CSE from barley methanol extract inhibits NO production in LPS-stimulated macrophage cells
We initially examined the effects of ether soluble extract (ESE), CSE, butanol soluble extract (BSE) and water soluble extract (WSE), which were serially extracted from barley methanol extracts (ME), on the NO production in LPS-stimulated macrophage cells (). When the cells were exposed to 1 μg/mL of LPS for 48 h, the nitrite concentration in the culture medium increased up to 10.43-fold (53.2 ± 3.1 μM) in comparison to the control value (5.1 ± 0.61 μM). Pre-treatment with ME and CSE significantly attenuated the LPS-stimulated NO production by 36.5 ± 2.5 (p < 0.05) and 23.9 ± 2.9 μM (p < 0.01), respectively. In contrast, ESE, BSE and WSE did not reduce NO levels in LPS-stimulated cells.
Figure 2. Effects of the serial extracts from the methanol extract of the aerial parts of barley on the NO production in LPS-stimulated RAW 264.7 cells. The cells were incubated for 48 h with 100 μg/mL of each extract in the presence of 1 μg/mL LPS and the levels of NO in the conditioned media were measured. *p < 0.05 and **p < 0.01 versus the LPS treatment alone.
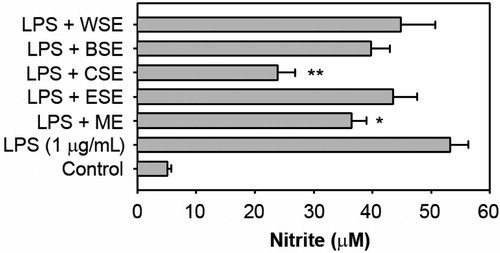
Inhibitory activity of sub-fractions from CSE on NO production and iNOS induction
Based on the activity that inhibited NO levels, we further isolated sub-fractions from the CSE using Sephadex LH-20 column chromatography and we obtained 16 fractions, as shown in . shows that, with the exception of F2 and F6, the fractions significantly suppressed LPS-stimulated NO production, although the activity differed according to the fractions. Pre-treatment with the fractions ranging from F7 to F14 at 100 μg/mL in particular reduced the LPS-stimulated NO levels to the basal level. Taking into consideration the inhibitory activities on the LPS-induced NO production and the chemical properties of the solution to elute, we finally selected the F4, F7, F9 and F12 fractions as the active fractions and further investigated their potential to suppress the LPS-induced inflammation and the mechanisms involved using in vitro and in vivo systems. reveals that the four fractions noted above at 100 μg/mL almost completely suppressed the iNOS expression in the LPS-stimulated macrophages. These fractions also showed a dose-dependent inhibition of the LPS-stimulated NO production (Supplement Figure 1).
Figure 3. Effects of the sub-fractions eluted from the chloroform soluble extract on the NO production and iNOS induction in LPS-stimulated RAW 264.7 cells. (A) The cells were incubated for 48 h with 100 μg/mL of each fraction in the presence of 1 μg/mL LPS and the levels of NO in the conditioned media were determined. *p < 0.05, **p < 0.01 and ***p < 0.001 versus the LPS treatment alone. (B) The cells were also treated with 100 μg/mL of each of the four active fractions in the presence of 1 μg/mL LPS for 24 h and then whole protein lysates from the cells were analyzed by Western blot analysis. The level of the iNOS protein was measured by densitometric analysis after normalizing it to that of β-actin. ***p < 0.001 versus the non-treated control cells. ###p < 0.001 versus the LPS treatment alone.
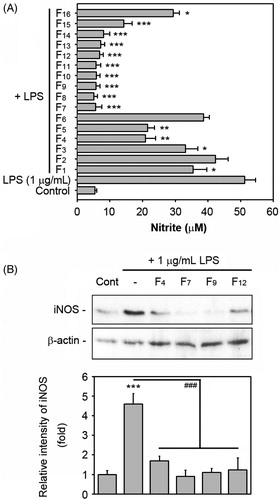
The four active fractions diminished LPS-induced production of pro-inflammatory cytokines in macrophages
We assessed the effects of the selected fractions on the production of TNF-α, IL-1β and IL-6 in LPS-exposed cells. The secretion of these cytokines was measured using the culture media of cells stimulated with 1 μg/mL LPS alone or in combination with the fractions for 48 h. The addition of LPS into the cells increased the level of TNF-α (41.5 ± 3.9 ng/mL), whereas pre-treatment with F4, F7, F9 and F12 at 100 μg/mL significantly diminished the TNF-α levels to 19.8 ± 2.5 (p < 0.01), 3.5 ± 1.1 (p < 0.001), 1.2 ± 1.2 (p < 0.001) and 1.7 ± 0.67 ng/mL (p < 0.001), respectively (). These fractions also showed a dose-dependent inhibition of the LPS-stimulated TNF-α secretion (Supplement Figure 2). In addition, the fractions suppressed the LPS-stimulated IL-6 production in the cells and F12 showed the most potent inhibitory activity (). Similarly, treatment with these fractions reduced the IL-1β levels in the LPS-stimulated cells and F9 and F12 at 100 μg/mL decreased the levels to that of the basal levels (). These fractions alone did not stimulate the production of cytokines in the cells (data not shown). When the effects of the active barley fractions on several cells in order to understand their biological properties, treatment with F4 at 100 μg/mL did not block the viability of cancer cells that were tested, while F7 showed mild inhibition of the HepG2 cells only (Supplement Figure 3). The addition of F9 or F12 to the cultures caused a dramatic increase in the inhibition of the viability of the cancer cells, when the inhibitory activity of F9 was higher than that of F12. However, F9 also revealed a cytotoxic effect on the primary cultured HGF.
Figure 4. Effects of the fractions F4, F7, F9 and F12 on the production of pro-inflammatory cytokines in LPS-stimulated RAW 264.7 cells. The cells were stimulated with 1 μg/mL LPS in the presence and absence of 100 μg/mL of each fraction. After 48 h of co-incubation, the levels of TNF-α, IL-6 and IL-1β in the culture supernatants were measured by ELISA. The data represent the mean values of three independent experiments. ***p < 0.001 versus the non-treated control cells. #p < 0.05, ##p < 0.01 and ###p < 0.001 versus the LPS treatment alone. ND = non-detectable.
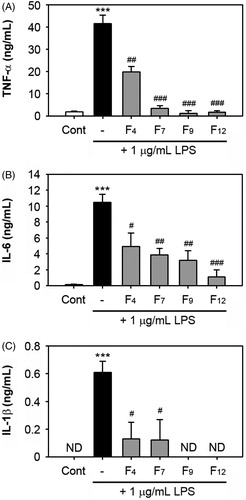
The active fractions inhibit NF-κB DNA-binding activity in LPS-stimulated RAW 264.7 cells
As shown in , LPS treatment at 1 μg/mL increased the DNA-NF-κB binding in macrophages, but this was almost completely inhibited by the addition of the fractions at concentrations of 100 μg/mL. F12 showed the most efficient effect on the inhibition of the NF-κB DNA-binding activity. Consistent with this result, an enzymatic assay revealed that all the tested fractions significantly suppressed the LPS-induced p65 binding activity.
Figure 5. Effects of the four active fractions on NF-κB DNA binding in LPS-stimulated RAW 264.7 cells. The cells were pre-treated with 100 μg/mL of each fraction for 1 h just before stimulation with 1 μg/mL LPS. After 1 h of stimulation, nuclear fractions were prepared from the cells and processed for the analyses of NF-κB binding activity by EMSA (A) and non-isotopic enzymatic assay (B). The results from the EMSA show a representative result from three different experiments. ***p < 0.001 versus the non-treated control cells. #p < 0.05 and ##p < 0.01 versus the LPS treatment alone.
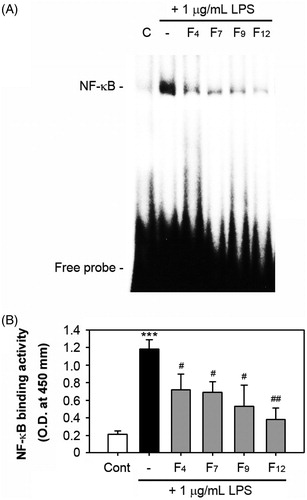
The active fractions improve survival in a sepsis model and decrease serum levels of pro-inflammatory cytokines
Next we examined how the active fractions isolated from the CSE affect the survival rate in a sepsis model (). The survival rate of the mice injected intraperitoneally with LPS gradually decreased, such that the LPS-injected mice showed 80, 60, 40 and 20% survival at 18, 24, 30 and 48 h after the LPS injection, respectively. In contrast, the survival of the mice pretreated with the active fractions was higher than that of the mice injected with LPS only. Oral administration of the F4, F7, F9 and F12 improved the mouse survival rates to 80, 80, 60 and 80%, respectively, at 48 h after the LPS injection. Since the pathogenic mechanism of sepsis involves intravascular inflammation mediated by various pro-inflammatory cytokines, the serum levels of the TNF-α, IL-1β and IL-6 concentrations in the mice were measured. The serum TNF-α levels rapidly increased to 4.12 ± 0.58 ng/mL at 2 h after the LPS injection, whereas the levels decreased to 1.8 ± 0.32 ng/mL at 8 h (). The serum TNF-α levels in the control mice were not detectable. Oral treatment with F7 only showed a preventive effect on the acute increase in the serum TNF-α levels, when the sera TNF-α levels were measured at 2 h after the LPS injection. In contrast, all the fractions showed significant activity that caused a decrease in the serum TNF-α levels, when the sera were measured at 8 h after the LPS injection. The serum concentrations of IL-6 and IL-1β dramatically increased in the mice only injected with the LPS compared to the concentrations in the control mice (). All of the active fractions reduced these cytokine levels in the sera measured at 2 h after the LPS injection. In contrast, when the sera were collected at 8 h after the LPS injection, both the IL-6 and IL-1β serum levels were significantly decreased in the mice co-treated with the fractions in comparison to the mice only injected with LPS.
Figure 6. Protective effects of the active fractions of barley in a murine sepsis model. As described in the materials and methods section, mice were injected with LPS (30 mg/kg BW) as an experimental sepsis model. The effects of the co-administration with the fractions F4, F7, F9 and F12, on survival rate were analyzed every 6 h after LPS injection for 4 d.
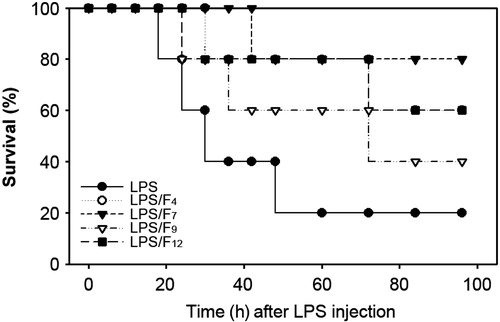
Figure 7. Modulation of the serum cytokine concentrations by oral administration of the active fractions of barley in a murine sepsis model. The sera from the mice that were orally administered each fraction were collected 2 and 8 h after the LPS injection and processed in order to determine the serum levels of TNF-α, IL-6 and IL-1β. #p < 0.05 and ##p < 0.01 versus the mice injected with LPS only. ND = non-detectable.
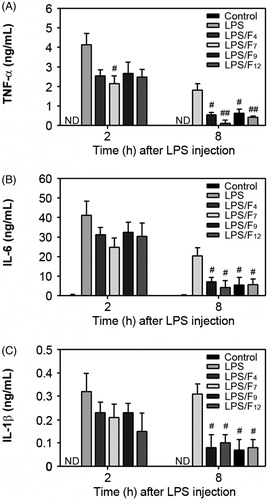
Identification of the compounds contained in the barley fractions through GC-MS analysis
The chemical compositions of the active fractions were analyzed by GC-MS chromatography and a total of 15 compounds were identified in the four fractions (). Identification of the constituents was performed by comparing the recorded mass spectra with the standard mass spectra from the Agilent ChemStation software (Agilent Technologies, Inc., Alpharetta, GA). The primary constituents that had mass spectral fit values of 83% or greater are presented in and their chemical structures are shown in . The major constituents of the F4 as determined by GC-MS analysis were found to be phenolic compounds, while benzenepropanoic acid 4-hydroxy-3-methoxy was the most abundant compound. The primary constituent of F7 was 45.74% 7-methoxy-4a-methyl-9,10-dihydro-2(4aH)-phenanthrenone, while its identity (%) was relatively lower than the other compounds. The GC-MS analysis also showed the presence of three different compounds in F9, but their constitution was only 1.06, 0.6 and 3.87%, respectively. Almost all the constituents of F9 did not show high-mass spectral fit values in comparison to the 15 identified compounds. We further demonstrated that the primary constituents of F12 were mostly fatty acids, in which hexadecanoic acid methyl ester (20.39%) was the major constituent.
Figure 8. The structures of the 15 identified compounds in the four active fractions isolated from the methanol extract of the aerial parts of barley.
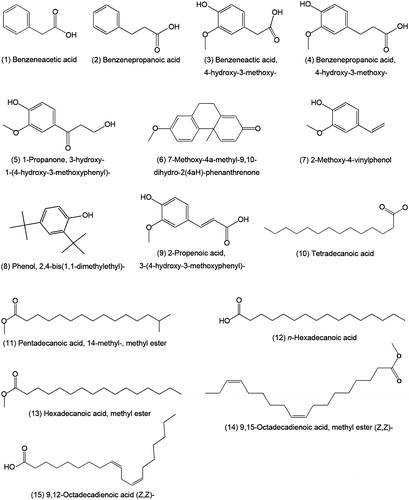
Table 1. The main compounds identified in the four active fractions isolated from barley aerial part methanol extract.
Discussion
Inflammation is an immune response to endotoxins that is mediated by complex processes with several classic signs. The prominent changes observed during inflammation are the increases in the levels of NO, cell adhesion molecules, COX2 and various cytokines including TNF-α, IL-6, IL-1β, IFN-γ and IL-12 (Munhoz et al., Citation2008). Over-production of these inflammatory mediators by macrophages stimulates inflammatory responses and leads to various pathological disorders such as carcinogenicity, cytotoxicity and autoimmune diseases. Therefore, the selective inhibition and/or modulation of these mediators are considered as the most efficient approach to alleviate a variety of disorders triggered by inflammation.
In this study, we showed that CSE, but not ESE, BSE and WSE, inhibited NO production in LPS-stimulated macrophage cells, in which its activity was higher than that for ME. After fractionation of CSE, we selected the F4, F7, F9 and F12 fractions as the active fractions. Subsequent experiments revealed a potential for inhibiting LPS-mediated stimulation of NO production and iNOS expression, as well as of pro-inflammatory cytokines such as TNF-α, IL-6 and IL-1β. F7, F9 or F12, in particular, almost completely prevented the LPS-induced production of NO and TNF-α. These results demonstrate for the first time that the methanol extracts from the aerial parts of barley have anti-inflammatory effect on LPS-stimulated macrophages.
In response to endotoxicity, the activation of the NF-κB transcription factor is essential for iNOS gene transcription and the subsequent production of NO with the production of pro-inflammatory cytokines (Doyle & O'Neill, Citation2006). The results from the EMSA showed that the four active fractions inhibited the LPS-induced activation of NF-κB DNA-binding. Based on the non-isotopic enzymatic assay, p65 is considered to be the primary subunit that causes dimerization with p50 or p52 in the NF-κB DNA-binding. These findings indicate that the active fraction-mediated suppression of LPS-induced NO and cytokine production is closely associated with their inhibitory potential of NF-κB DNA-binding activation. There are numerous studies demonstrating that the potential of bioactive substances to inhibit the production of inflammatory mediators in LPS-stimulated macrophages is related to their abilities to suppress NF-κB-mediated pathways (Hong et al., Citation2009; Lee et al., Citation2008; Shin et al., Citation2009; Wu et al., Citation2004).
It is important to note that NF-κB activation is tightly regulated by mitogen-activated protein kinases (MAPK). In particular, extracellular signal-regulated kinase (ERK) and p38 MAPK are known to primarily mediate the cellular signaling involved in the activation of macrophages in response to microbial pathogens (Gaestel et al., Citation2007; Xagorari et al., Citation2002). Our previous findings have shown the critical roles that ERK and p38 MAPK play in LPS-stimulated macrophages (Fang et al., Citation2011; Hwang et al., Citation2010). Although it is still unclear whether the activation of both or either of ERK1/2 or p38 MAPK are required for LPS-induced NO production in macrophages (Watters et al., Citation2002), it is believed that the active fractions from the aerial parts of barley inhibit the NF-κB-mediated pathways by preventing the activation of ERK and/or p38 MAPK in LPS-stimulated macrophages.
While chronic inflammation is related to various degenerative diseases, acute inflammation can cause death directly. Approximately 900 000 cases of sepsis per year are reported in the United States, 20% of which result in mortality (Martin et al., Citation2003). Many investigators have focused their efforts on the development of bioactive materials to combat the acute, severe inflammation that occurs during sepsis (Moore et al., Citation2008; Zeni et al., Citation1997). Our present results showed that the active fractions increased the survival rate (%) of mice injected with LPS. This may suggest that the active fractions of the aerial parts of barley exert a beneficial effect on acute inflammatory responses.
The pathogenic mechanism of sepsis primarily involves intravascular inflammation mediated by various cytokines and chemokines (Bone et al., Citation1997). Therefore, determining the serum levels of pro-inflammatory cytokines in a sepsis animal model is considered to be one of the primary approaches used to estimate the mechanisms by which an active material protects from acute and severe inflammation. In general, the serum levels of TNF-α and IL-1β in normal BALB/c mice without any treatment were barely detectable, while the IL-6 level was between 1 and 2 ng/mL (Blanque et al., Citation1996). These results were also in parallel with our present study in which IL-6 was only slightly detectable. Following LPS challenge, the TNF-α serum levels quickly and significantly increased with the attendant augmentation of IL-6 and IL-1β (Kotanidou et al., Citation2002; Moore et al., Citation2008). Although such increases in inflammatory cytokines differed according to the doses of LPS challenged and/or the animal models used, most previous findings support a negative correlation between survival rate and the serum levels of these inflammatory cytokines (Blanque et al., Citation1996; Jones et al., Citation2007; Lee et al., Citation2008). This indicates that the potential of an active material to reduce the serum levels of inflammatory cytokines is directly related to its ability to block LPS-mediated acute inflammation. Our current findings revealed that the serum levels of TNF-α, IL-6 and IL-1β rise acutely and persistently within 2 h after the LPS injection. These increases were maintained up to 8 h after the LPS challenge, although the levels of TNF-α and IL-6 were somewhat lower than those measured at 2 h. Oral administration of the active fractions significantly diminished the serum levels of these cytokines at 8 h after the LPS injection in comparison to the mice only challenged with LPS. However, almost none of the active fractions inhibited the increase in the serum cytokine levels at 2 h after the LPS injection. These results suggest that the serum levels of TNF-α, IL-6 and IL-1β at 8 h after the LPS challenge are negatively correlated with survival rate. To be exact, facilitating a decrease in the serum levels of inflammatory cytokines is also a beneficial effect of a bioactive compound on acute inflammation.
Another study on a murine model of LPS-induced sepsis reported the involvement of IFN-γ and IL-10. In patients with sepsis, IFN-γ levels are correlated with poor outcomes (Jain et al., Citation2010). The role of IFN-γ has been further elucidated by a study in which IFN-γ-specific antibodies attenuate the in vivo effects in animal models of sepsis (Nakane et al., Citation1995). While cytokines including TNF-α, IL-6, IL-1β and IFN-γ increase the inflammatory response during the process of sepsis, IL-10 is involved in the inhibition of the inflammatory production (Thijs & Hack, Citation1995; Moore et al., Citation2008). It has been reported that the IL-10-mediated decrease in these cytokines in serum is correlated with increased survival rates (Gérard et al., Citation1993; Oberholzer et al., Citation2002). Although further research is needed to clarify the underlying pathophysiologic mechanisms, we cannot rule out that the improved survival in a septic animal model treated with the active fractions of the aerial parts of barley is in part correlated with the modulation of the IFN-γ and IL-10 serum levels. Further, it is likely that the different anti-inflammatory effects of the fractions between in vitro and in vivo experimental systems are derived from the differed uptake and metabolisms within cells versus the body, as well as from the varied constituents in the fractions.
Many studies have identified the beneficial activities of phenolic compounds on anti-inflammation (Ríos et al., Citation2009; Wilson, Citation2009). Based on GC-MS analysis, the active fractions F4, F7 and F9 consist of primarily phenolic compounds. Benzoic acid derivatives, in particular, are the primary active components in F4. In fact, there are several classes of compounds in barley that have a phenolic structure, such as benzoic and cinnamic acid derivatives, proanthocyanidins, quinines and pholyphenolic compounds (Bonoli et al., Citation2004; Ferreres et al., Citation2009; Hernanz et al., Citation2001). Barley leaf extract has been found to contain a large amount of polyphenols and vitamins (Yu et al., 2002b). Roasted barley grains contain various antioxidative compounds, which are mostly benzoic and cinnamic acid derivatives (Omwamba & Hu, Citation2010). One of the benzoic acid derivatives, 3,4-dihydroxybenzaldehyde, was determined to be the active compound responsible for the antioxidant activity of barley seed extract (Jeong et al., Citation2009). The results of these studies support our present findings that the benzoic acid and cinnamic acid derivatives are one of the primary active compounds in the active fractions of the aerial parts of barley. However, we could not identify the active compounds of the F9, although the 3.87% ferulic acid was believed to be the active material for the anti-inflammation effects. Other non-volatile compounds that are not detectable by GC-MS chromatography might also have contributed to the bioactivity of the fraction. Interestingly, this fraction also showed anti-cancer effects in vitro, although it has a mild cytotoxic effect on primary cultured cells. Consequently, more detailed experiments will be needed in order to identify the active constituents in F9.
Fatty acids are known to have antibacterial and antifungal properties (Chandrasekaran et al., Citation2011) as well as anti-inflammatory potential (Leventhal et al., Citation1993). Fatty acids can modulate immune responses by acting directly on T lymphocytes (Aparna et al., Citation2012). Linoleic acid exerts anti-inflammatory effects by decreasing the production of inflammatory mediators including PGE2, IL-6, IL-1β, TNF-α, and NO (Yu et al., 2002c). The polyunsaturated fatty acids from seaweed, stearidonic acid and hexadecatetraenoic acid, were found to suppress eicosanoid production (Ishihara et al., Citation1998). The fatty acid n-hexadecanoic acid, is known to exert anti-inflammatory actions via the inhibition of phospholipase A2, and therefore the oils that are abundant in n-hexadecanoic acid are considered to be useful for the treatment of rheumatic symptoms (Aparna et al., Citation2012). It was also reported that the dried flower buds of Magnolia biondii Pamp have significant anti-inflammatory activities through the induction of a substantial and characteristic change in the metabolic profiles of various substances including hexadecanoic acid, linoleic acid, oleic acid, stearic acid and cholesterol (Qi et al., Citation2011). In addition, (10E,12Z,15Z)-9-hydroxy-10,12,15-octadecatrienoic acid methyl ester derived from Ehretia dicksonii is a potent anti-inflammatory fatty acid (Dong et al., Citation2000). These reports strongly support the fact that the F12 fraction showed potent anti-inflammatory activity in vitro and in vivo. It is also likely that the marked inhibition of the viability of several cancer cells by F12 is related to its constituents. However, we could not exclude a possibility that polyphenol compounds such as flavonoids which are undetectable by GC-MS analysis are related to anti-tumor effect of F9 and F12. Collectively, our current results indicate for the first time the anti-inflammatory potential of the fatty acids contained in the aerial parts of barley.
Conclusion
This study demonstrates that several active fractions from the methanol extract of the aerial parts of barley have an anti-inflammatory effect on LPS-activated macrophages. The primary mechanisms by which the active fractions exert anti-inflammatory activity are related to the suppression of the NF-κB DNA-binding activation. Overall, the results of our study indicated that phenolic compounds and fatty acids are the primary bioactive constituents contained in the active fractions isolated from the aerial parts of barley. These findings suggest that the aerial parts of barley may be useful as functional foods in a therapeutic approach for the treatment of inflammatory diseases.
Declaration of interest
The authors report no declarations of interest. This work was supported by a grant from the RDA, Ministry of Agriculture and Forestry, Republic of Korea (PJ007538).
Supplementary Material
Download PDF (143.5 KB)References
- Aparna V, Dileep KV, Mandal PK, et al. (2012). Anti-inflammatory property of n-hexadecanoic acid: Structural evidence and kinetic assessment. Chem Biol Drug Des 80:434–9
- Blanque R, Meakin C, Millet S, Gardner CR. (1996). Hypothermia as an indicator of the acute effects of lipopolysaccharides: Comparison with serum levels of IL-1, IL-6 and TNF-α. Gen Pharmacol 27:973–7
- Bone RC, Grodzin CJ, Balk RA. (1997). Sepsis: A new hypothesis for pathogenesis of the disease process. Chest 112:235–43
- Bonoli M, Verardo V, Marconi E, Caboni MF. (2004). Antioxidant phenols in barley (Hordeum vulgare L.) flour: Comparative spectrophotometric study among extraction methods of free and bound phenolic compounds. J Agric Food Chem 52:5195–200
- Chandrasekaran M, Senthilkumar A, Venkatesalu V. (2011). Antibacterial and antifungal efficacy of fatty acid methyl esters from the leaves of Sesuvium portulacastrum L. Eur Rev Med Pharmacol Sci 15:775–80
- Chanput W, Reitsma M, Kleinjans L, et al. (2012). β-Glucans are involved in immune-modulation of THP-1 macrophages. Mol Nutr Food Res 56:822–33
- Dong M, Oda Y, Hirota M. (2000). (10E,12Z,15Z)-9-hydroxy-10,12,15-octadecatrienoic acid methyl ester as an anti-inflammatory compound from Ehretia dicksonii. Biosci Biotechnol Biochem 64:882–6
- Doyle SL, O'Neill LA. (2006). Toll-like receptors: From the discovery of NFkappaB to new insights into transcriptional regulations in innate immunity. Biochem Pharmacol 72:1102–13
- Fang M, Lee SY, Park SM, et al. (2011). Anti-inflammatory potential of Phaseolus calcaratus Roxburgh, a oriental medicine, on LPS-stimulated RAW 264.7 macrophages. J Pharm Pharmacol 63:120–8
- Ferreres F, Krsková Z, Gonçalves RF, et al. (2009). Free water-soluble phenolics profiling in barley (Hordeum vulgare L.). J Agric Food Chem 57:2405–9
- Gaestel M, Mengel A, Bothe U, Asadullah K. (2007). Protein kinases as small molecule inhibitor targets in inflammation. Cur Med Chem 14:2214–34
- Gérard C, Bruyns C, Marchant A, et al. (1993). Interleukin 10 reduces the release of tumor necrosis factor and prevents lethality in experimental endotoxemia. J Exp Med 177:547–50
- Hernanz D, Nuñez V, Sancho AI, et al. (2001). Hydroxycinnamic acids and ferulic acid dehydrodimers in barley and processed barley. J Agric Food Chem 49:4884–8
- Hesslinger C, Strub A, Boer R, et al. (2009). Inhibition of inducible nitric oxide synthase in respiratory diseases. Biochem Soc Trans 37:886–91
- Hong YH, Chao WW, Chen ML, Lin BF. (2009). Ethyl acetate extracts of alfalfa (Medicago sativa L.) sprouts inhibit lipopolysaccharide-induced inflammation in vitro and in vivo. J Biomed Sci 16:64
- Hsieh IN, Chang AS, Teng CM, et al. (2011). Aciculatin inhibits lipopolysaccharide-mediated inducible nitric oxide synthase and cyclooxygenase-2 expression via suppressing NF-κB and JNK/p38 MAPK activation pathways. J Biomed Sci 18:28
- Hwang JM, Yu JY, Jang YO, et al. (2010). A phenolic acid phenethyl urea compound inhibits lipopolysaccharide-induced production of nitric oxide and pro-inflammatory cytokines in cell culture. Int Immunopharmacol 10:526–32
- Ishihara K, Murata M, Kaneniwa M, et al. (1998). Inhibition of icosanoid production in MC/9 mouse mast cells by n-3 polyunsaturated fatty acids isolated from edible marine algae. Biosci Biotechnol Biochem 62:1412–15
- Jain V, Singh PP, Silawat N, et al. (2010). A preliminary study on pro- and anti-inflammatory cytokine profiles in Plasmodium vivax malaria patients from central zone of India. Acta Trop 113:263–8
- Jeong JB, Hong SC, Jeong HJ. (2009). 3,4-Dihydroxybenzaldehyde purified from the barley seeds (Hordeum vulgare) inhibits oxidative DNA damage and apoptosis via its antioxidant activity. Phytomedicine 16:85–94
- Jones KL, Mansell A, Patella S, et al. (2007). Activin A is a critical component of the inflammatory response, and its binding protein, follistatin, reduces mortality in endotoxemia. Proc Natl Acad Sci USA 104:16239–44
- Kamiyama M, Shibamoto T. (2012). Flavonoids with potent antioxidant activity found in young green barley leaves. J Agric Food Chem 60:6260–7
- Kang EH, Gebru E, Kim MH, et al. (2009). EstA protein, a novel virulence factor of Streptococcus pneumoniae, induces nitric oxide and pro-inflammatory cytokine production in RAW 264.7 macrophages through NF-kappaB/MAPK. Microb Pathog 47:196–201
- Kanwar JR, Kanwar RK, Burrow H, Baratchi S. (2009). Recent advances on the roles of NO in cancer and chronic inflammatory disorders. Cur Med Chem 16:2373–94
- Kern TS. (2007). Contributions of inflammatory processes to the development of the early stages of diabetic retinopathy. Exp Diabetes Res 2007:95–103
- Kook SH, Son YO, Choe Y, et al. (2009). Mechanical force augments the anti-osteoclastogenic potential of human gingival fibroblasts in vitro. J Periodontal Res 44:402–10
- Kotanidou A, Xagorari A, Bagli E, et al. (2002). Luteolin reduces lipopolysaccharide-induced lethal toxicity and expression of pro-inflammatory molecules in mice. Am J Respir Crit Care Med 165:818–23
- Lee HJ, Oh TH, Yoon WJ, et al. (2008). Eutigoside C inhibits the production of inflammatory mediators (NO, PGE(2), IL-6) by down-regulating NF-kappa B and MAP kinase activity in LPS-stimulated RAW 264.7 cells. J Pharm Pharmacol 60:917–24
- Leventhal LJ, Boyce EG, Zurier RB. (1993). Treatment of rheumatoid arthritis with gamma linolenic acid. Ann Intern Med 119:867–73
- Martin GS, Mannino DM, Eaton S, Moss M. (2003). The epidemiology of sepsis in the United States from 1979 through 2000. N Engl J Med 348:1546–54
- Moore CC, Martin EN, Lee GH, et al. (2008). An A2A adenosine receptor agonist, ATL313, reduces inflammation and improves survival in murine sepsis models. BMC Infect Dis 8:141
- Munhoz CD, García-Bueno B, Madrigal JL, et al. (2008). Stress-induced neuroinflammation: Mechanisms and new pharmacological targets. Braz J Med Biol Res 41:1037–46
- Nakagawa H, Maeda S. (2012). Inflammation- and stress-related signaling pathways in hepatocarcinogenesis. World J Gastroenterol 18:4071–81
- Nakane A, Okamoto M, Asano M, et al. (1995). Endogenous gamma interferon, tumor necrosis factor, and interleukin-6 in Staphylococcus aureus infection in mice. Infect Immun 63:1165–72
- Oberholzer A, Oberholzer C, Moldawer LL. (2002). Interleukin-10: A complex role in the pathogenesis of sepsis syndromes and its potential as an anti-inflammatory drug. Crit Care Med 30:S58–63
- Omwamba M, Hu Q. (2010). Antioxidant activity in barley (Hordeum vulgare L.) grains roasted in a microwave oven under conditions optimized using response surface methodology. J Food Sci 75:C66–73
- Qi Y, Qu L, Wu Y, Fan G. (2011). A plasma metabonomic investigation into the intervention of volatile oil of Magnolia biondii Pamp on rat model of acute inflammation. J Ethnopharmacol 137:487–94
- Quinde-Axtell Z, Baik BK. (2006). Phenolic compounds of barley grain and their implication in food product discoloration. J Agric Food Chem 54:9978–84
- Ríos JL, Recio MC, Escandell JM, Andújar I. (2009). Inhibition of transcription factors by plant-derived compounds and their implications in inflammation and cancer. Curr Pharm Des 15:1212–37
- Shin KM, Shen L, Park SJ, et al. (2009). Bis-(3-hydroxyphenyl) diselenide inhibits LPS-stimulated iNOS and COX-2 expression in RAW 264.7 macrophage cells through the NF-kappa B inactivation. J Pharm Pharmacol 61:479–86
- Son YO, Jang YS, Heo JS, et al. (2009). Apoptosis-inducing factor plays a critical role in caspase-independent, pyknotic cell death in hydrogen peroxide-exposed cells. Apoptosis 14:796–808
- Sung CS, Wong CS. (2007). Cellular mechanisms of neuroinflammatory pain: The role of interleukin-1beta. Acta Anaesthesiol Taiwan 45:103–9
- Thijs LG, Hack CE. (1995). Time course of cytokine levels in sepsis. Intensive Care Med 21:S258–63
- Vuolteenaho K, Moilanen T, Knowles RG, Moilanen E. (2007). The role of nitric oxide in osteoarthritis. Scand J Rheumatol 36:247–58
- Watters JJ, Sommer JA, Pfeiffer ZA, et al. (2002). A differential role for the mitogen-activated protein kinases in lipopolysaccharide signaling: The MEK/ERK pathway is not essential for nitric oxide and interleukin 1beta production. J Biol Chem 277:9077–87
- Wilson JX. (2009). Mechanism of action of vitamin C in sepsis: Ascorbate modulates redox signaling in endothelium. Biofactors 35:5–13
- Wu MJ, Wang L, Ding HY, et al. (2004). Glossogyne tenuifolia acts to inhibit inflammatory mediator production in a macrophage cell line by downregulating LPS-induced NF-kappa B. J Biomed Sci 11:186–99
- Xagorari A, Roussos C, Papapetropoulos A. (2002). Inhibition of LPS-stimulated pathways in macrophages by the flavonoid luteolin. Br J Pharmacol 136:1058–64
- Yamaura K, Nakayama N, Shimada M, et al. (2012). Antidepressant-like effects of young green barley leaf (Hordeum vulgare L.) in the mouse forced swimming test. Pharmacognosy Res 4:22–26
- Yu YM, Chang WC, Chang CT, et al. (2002a). Effects of young barley leaf extract and antioxidative vitamins on LDL oxidation and free radical scavenging activities in type 2 diabetes. Diabetes Metab 28:107–14
- Yu YM, Wu CH, Tseng YH, et al. (2002b). Antioxidative and hypolipidemic effects of barley leaf essence in a rabbit model of atherosclerosis. Jpn J Pharmacol 89:142–8
- Yu Y, Correll PH, Vanden Heuvel JP. (2002c). Conjugated linoleic acid decreases production of pro-inflammatory products in macrophages: Evidence for a PPAR gamma dependent mechanism. Biochim Biophys Acta 1581:89–99
- Zeni F, Freeman B, Natanson C. (1997). Anti-inflammatory therapies to treat sepsis and septic shock: A reassessment. Crit Care Med 25:1095–100