Abstract
Context: For its variety of biological activities, Tamarix aucheriana (Decne.) Baum. (Tamaricaceae) has an extensive history as a traditional Arab medicine.
Objectives: Antimitogenic and chemo-sensitizing activities of syringic acid (SA) were studied against human colorectal cancer.
Materials and methods: Chromatographic and spectral data were used for the isolation and identification of SA. MTT, flow cytometry, in vitro invasion and angiogenesis assays, fluoremetry, ELISA and Real Time qPCR were used to test antimitogenic and chemo-sensitizing activities of SA, cell cycle, apoptosis, proteasome and NFκB-DNA-binding activities, cancer cell invasion and angiogenesis, and expression of cell cycle/apoptosis-related genes.
Results: SA showed a time- and dose-dependent (IC50 = 0.95–1.2 mg mL−1) antimitogenic effect against cancer cells with little cytotoxicity on normal fibroblasts (≤20%). SA-altered cell cycle (S/G2-M or G1/G2-M phases) in a time-dependent manner, induced apoptosis, inhibited DNA-binding activity of NFκB (p ≤ 0.0001), chymotrypsin-like/PGPH (peptidyl-glutamyl peptide-hydrolyzing) (p ≤ 0.0001) and the trypsin-like (p ≤ 0.002) activities of 26S proteasome and angiogenesis. SA also differentially sensitized cancer cells to standard chemotherapies with a marked increase in their sensitivity to camptothecin (500-fold), 5FU (20 000-fold), doxorubicin (210-fold), taxol (3134-fold), vinblastine (1000-fold), vincristine (130-fold) and amsacrine (107-fold) compared to standard drugs alone.
Discussion: SA exerted its chemotherapeutic and chemo-sensitizing effects through an array of mechanisms including cell-cycle arrest, apoptosis induction, inhibition of cell proliferation, cell migration, angiogenesis, NFκB DNA-binding and proteasome activities.
Conclusion: These results demonstrate the potential of SA as an antimitogenic and chemo-sensitizing agent for human colorectal cancer.
Introduction
Several studies have shown that natural products have a wide spectrum of biological activities including anti-inflammatory, antioxidant, antimutagenic and anticancer properties (Espin et al., Citation2007; Miyata, Citation2007). Phytochemicals can be as simple as phenolics to complex molecules such as taxol and vincristine (Nishino et al., Citation2007). Several epidemiological studies have validated the inverse relationship between the consumption of nutraceuticals and colon cancer (Lila, Citation2007).
The occurrence of colon cancer is correlated to age and gender, with 90% of the cases arising in 50 years or older subjects, predominantly males (Smith et al., Citation2008). Epidemiological studies suggest that colon cancer is a manifestation of a number of inherited cancer predisposition syndromes, including familial adenomatous polyposis and inflammatory bowel disease (Rowley, Citation2005). Other factors including obesity, lack of exercise, smoking, alcohol consumption and fat rich-diet have been associated with an increased risk of colon cancer (Cappell, Citation2007).
Although many studies have described the chemopreventive role of polyphenols such as flavonoids, less attention has been focused on simple phenolic acids. It is plain to understand that in food, polyphenols co-occurring with simple phenolic antioxidants may also occur in foods (Herrmann, Citation1989). Phenolic compounds are secondary metabolites derived from polyacetate/shikimate pathway in plants and due to their powerful antioxidant activities, many of these compounds have been associated with health benefits (Heim et al., Citation2002; Hertog et al., Citation1993; Randhir et al., Citation2004). However, little is known about the role of phenolic acids in cancer prevention and antigenotoxicity (Clifford, Citation2000).
Some Tamarix (Tamaricaceae) species are widely used as astringent, aperitif and diuretic in traditional medicine in Asia and Africa (Gaston, Citation1998; Ghazanfar, Citation1994). Thus, boiled leaves and young branches of Tamarix are used for treating spleen edema and when mixed with ginger the extract is used for uterus infections, prolonged and difficult labor, diverse sores and wounds (Boulos, Citation1983; Ghazanfar, Citation1994). Its tannins are used for the treatment of leucoderma, spleen troubles, eye diseases rheumatism, jaundice and hepatic disorders (Sharma & Parmar, Citation1998; Sehrawat & Sultana, Citation2006a,Citationb).
The present study focused on the bioactivity-guided fractionation of Tamarax aucheriana (Decne.) Baum. for the identification of bioactive lead(s). The possible mechanism of action of the identified phytochemicals and their potential to sensitize human colorectal cancer cells to standard chemotherapeutic drugs were also investigated.
Materials and methods
Human colorectal cancer cell lines (SW1116 and SW837) and normal human fibroblast (CRL1554) were obtained from the American Type Culture Collection, ATCC (VA, USA). Trypsin, Leibovitz's L-15 and EMEM (Eagle Minimum Essential Medium) media, fetal bovine serum (FBS) and penicillin/streptomycin solution (200×) were obtained from Mediatech, Inc. (Herndon, VA). Annexin V-FITC apoptosis detection kit was obtained from BD Hoffmann-La Roche Inc. (Nutley, NJ). DNA-prep kit was obtained from Beckman & Coulter (Kendall, FL). Primers, Taqman probes and all the reagents for RT-PCR and Real-Time qPCR were obtained from Applied Biosystem (Carlsbad, CA). Chemicon’s cell invasion assay kit (Cat. No. ECM550) and Millipore in vitro angiogenesis assay kit (Cat. No. ECM625) were obtained from CHEMICON® International, Inc. (Temecula, CA) and MILLIPORE® International, Inc. (Billerica, MA), respectively. Cayman’s NFκB (p65) transcription factor assay kit was obtained from Cayman Chemical (Ann Arbor, MI); Nuclear/cytosol fractionation kit was obtained from Biovision Inc. (Milipitas, CA). Standard syringic acid (SA) and other chemicals were purchased from Sigma Aldrich Chemicals (St Louis, MO). Organic solvents of high-performance liquid chromatography (HPLC) grade were obtained from Fisher Scientific (Atlanta, GA).
Plant material
Tamarix aucheriana, a flowering shrub that grows up to 3 m tall, is woody with scattered branches, carrying densely arranged pink flowers. The fruit is cone-shaped, 3-valved capsule with many seeds; each seed has a terminal tuft of long hairs. It is a halophytic species, and in Kuwait, it is found in coastal areas and inland salt marshes (Daoud & Al-Rawi, Citation1985).
The aerial plant material was collected from the coastal areas near Sulaibikhat district during spring 2007. It was identified by the Herbarium Curator at Kuwait University, and a voucher specimen KTM 5461 was deposited in the University Herbarium (KTUH). The collected plant material was dried under shade (18–40 °C) and ground using a blender. The powdered plant material was packed in airtight plastic zipper bags for storage at room temperature.
Isolation and identification of SA from T. aucheriana
Tamarix aucheriana (256 g) was Soxhlet extracted with methanol to give 11.26 g of the crude material. This crude material (4 g) was fractionated on 200–300 mesh silica gel (BDH, UK) column (150 × 3 cm) packed in toluene. The column was eluted with an increasing percentage of methanol in chloroform 30:70 v/v (CH3OH:CHCl3). Fractions (20 mL) were collected, and analyzed on aluminum-coated silica gel thin-layer chromatography (TLC) plates using ethyl acetate:toluene:methanol (4:4:2; v/v) as a development solvent system. Fractions of the same composition were pooled and concentrated on a rotavapor (Buchi RE111 Rotavapor connected with Buchi 461 water bath and chiller HAAKE D8, Fisons, New Castle, DE) under reduced pressure (0–200 mbar). White crystalline flakes were recovered from the fractions eluted with CH3OH:CHCl3 (20:80; v/v). The product was recrystallized (1% yield of the crude extract) from ethyl acetate and identified as 4-hydroxy-3,5-dimethoxybenzoic acid (SA) using UV, IR, MS, 1H-NMR and 13C-NMR spectral data. The purified SA was chromatographically compared with pure commercially available SA (Sigma-Aldrich, S6881, Batch # 047K1477) (St. Louis, MO). The spectral data of the natural product and the authentic commercial product were essentially identical, confirming the identity of the natural SA.
Cell culture
Human colorectal cancer cell lines (SW1116 and SW837) were cultivated in Leibovitz’s L15 medium, 90% and fetal bovine serum, 10%. L15-medium formulation was devised for use in a free gas exchange with atmospheric air. Normal human fibroblasts (CRL1554) were cultivated in Dulbecco's modified Eagle medium (90%) and fetal bovine serum (10%).
Cell proliferation studies
The effect of SA on cell proliferation was studied by the MTT [3-(4,5-dimethylthiazol-2-yl)-2,5-diphenyltetrazolium bromide] assay and the color intensity was spectrophotometrically quantified (Abaza et al., Citation2008a). Colorectal cancer cell lines (SW1116 and SW837) and normal human fibroblasts (CRL1554) were plated in 96-well microtiter plates at a cell density of 27 × 103 cells/well. Cells were incubated for 24–72 h in a culture medium containing increasing concentration of SA (0–2 mg mL−1) at 37 °C in a non-CO2 incubator. After completion of the treatment period, the media were discarded and 100 µL/well of MTT (5 mg mL−1 membrane filter sterilized culture medium) was added and the plate was incubated for 4 h at 37 °C. MTT solution was aspirated and the purple-colored formazan crystals, thus formed, were dissolved in 200 µL/well of DMSO:ethanol (1:1; v/v) for 20 min at ambient temperature to lyse the cells and dissolve the colored crystals formed in viable cells. Change in absorbance was read at λ = 490 and 650 nm in an ELISA reader (Labsystems, Finland). For observing morphological changes, cancer cells SW1116 and SW837 were plated (2.5 × 105 cells mL−1) into a 24-well plate in a non-CO2 incubator for 18 h. Cells were treated with SA (7.5 mg mL−1) for 24 h and observed under an inverted microscope (Carl Zeiss MicroImaging GmbH, Gottingen, Germany).
Colony formation assay
The effect of SA, on the colony formation of human colorectal cancer cells, was determined as described previously (Abaza et al., Citation2008a). SW1116 and SW837 cells were plated (2.5 × 105 cells mL−1) for 18 h in a non-CO2 incubator. Cells were left untreated or treated with SA (7.5 mg mL−1) and incubated for 24 h. Cells were trypsinized, counted and plated at 500 cells mL−1 into a 6-well plate and incubated in a non-CO2 incubator for 10–14 d. Cells were fixed in 100% methanol for 30 min at room temperature and stained with 0.1% crystal violet, for 1 h. The stained colonies were counted and compared with control.
Flow cytometric analysis of the cell cycle
Distribution of cells, in cell cycle (Go/G1, S and G2/M) phases, was determined using flow cytometry by measuring DNA content of the nuclei labeled with propidium iodide (PI) as described previously (Abaza et al., Citation2008a). Human colorectal cancer cells SW1116 and SW837 were plated (1.0 × 105 cells mL−1) into 24-well plates and incubated at 37 °C in a non-CO2 incubator for 18 h. Cells were treated with SA (4.07 mg mL−1) for 24, 48 and 72 h, starting 18 h after seeding the cells in culture. Untreated and SA-treated human cancer cells were collected by trypsinization, washed with cold PBS (phosphate buffered saline) and counted. Cells were processed using a DNA-prep kit (Beckman & Coulter) and a DNA-Prep EPICS workstation (Beckman & Coulter). During this process, cells were treated with a cell-membrane permeabilizing agent (nonionic detergents) followed by PI and RNAase. The samples were incubated at room temperature for 15 min before analysis by flow cytometry (FC500, Beckman & Coulter). The percentage of cells in different cell-cycle phases were calculated using the Phoenix statistical software package, advanced DNA cell-cycle software (Phoenix Flow System, San Diego, CA).
Assessment of apoptosis by annexin V-FITC and PI staining
Cancer cell lines SW1116 and SW837 were plated (1.0 × 105 cells mL−1) into a 24-well plate and incubated at 37 °C in a non-CO2 incubator. Cells were treated with SA (4.07 mg mL−1) for 24 h. The assay was carried out according to the manufacturer's instructions. Cells from the control and treatment groups were resuspended in a 100 µL staining solution containing annexin-V fluorescein and PI in HEPES [4-(2-hydroxyethyl)piperazine-1-ethanesulfonic acid] buffer (annex V-FITC, BD Pharmingen, San-Diego, CA). The cells were analyzed by flow cytometry after incubation at room temperature for 15 min.
In vitro cell invasion assay
The in vitro inhibition of cancer cells invasion was tested using Chemicon’s cell invasion assay kit (ECM550). The dried ECM was re-hydrated by adding 300 µL of serum-free medium (warmed at 37 °C) to the interior of the inserts at room temperature for 1–2 h. The medium was removed from the inserts and 500 µL of medium containing 10% FBS was added to the lower chamber. Cancer cells SW1116 and SW837 were plated (2.5 × 105 cells/well) into 24-well plates and incubated at 37 °C in a non-CO2 incubator. Starting 18 h after seeding the cells in culture, they were treated with SA (7.5 mg mL−1) for 24 h and counted after harvesting. Untreated and SA-treated cells were added to each insert (300 µL containing 0.5–1 × 106 cells mL−1 in a serum free medium) and incubated for 24–72 h in a non-CO2 incubator at 37 °C. The non-invading cells, as well as the ECM (Extra cellular matrix) gel layer, were removed and the invasive cells on the lower surface of the membrane were stained by dipping the insert in staining solution for 20 min. The inserts were rinsed with water, air-dried and cells were counted by photographing the membrane through an inverted light microscope.
In vitro angiogenesis assay
The anti-angiogenic effect of SA was tested using a Millipore in vitro angiogenesis assay kit (ECM625). ECMatrix was thawed on ice and 100 µL of 10× ECMatrix diluent was added to 900 µL of ECMatrix gel solution and mixed. ECMatrix solution (50–100 µL) was transferred to each well of a 96-well tissue culture plate. The plate was incubated at 37 °C for 1 h. Cancer cells SW1116 and SW837 were plated (2.5 × 105 cells/well) into 24-well plates and incubated at 37 °C in a non-CO2 incubator. Starting 18 h after seeding the cells in culture, cells were treated with SA (7.5 mg mL−1) for 24 h and counted after harvesting. Untreated or SA-treated cancer cell lines SW1116 and SW837 were added (5000–10 000 cells/well) on top of the solidified matrix solution. The plate was incubated at 37 °C and tube formation was inspected under an inverted light microscope at 200× magnification.
Measurement of NFκB activity
Cancer cells SW1116 and SW837 were plated (2.5 ×105 cells mL−1) into a 24-well plate in a non-CO2 incubator for 18 h. The cells were treated with SA (7.5 mg mL−1) for 24 h. Cellular nuclear extracts were purified using a nuclear/cytosol fractionation kit (K266-25, Biovision, Inc.). NFκB (p65) DNA binding activity was determined by Cayman’s NFκB (p65) transcription factor assay (Cayman Chemical Company, Ann Arbor, MI). In this assay, a specific double-stranded DNA sequence containing the NFκB response element was immobilized onto the bottom of wells of a 96-well plate. NFκB (p65) of the nuclear extracts or positive control was detected by the addition of specific primary antibody directed against NFκB (p65). A second antibody conjugated to HRP (Horseradish peroxidase) was added to provide a sensitive colorimetric readout at λ = 450 nm.
Monitoring proteolytic activities of proteasome
Cancer cells SW1116 were plated (2.5 × 105 cells mL−1) into 24-well plates in a non-CO2 incubator for 18 h. Cells were treated with SA (7.5 mg mL−1) for 24 h. Cytosolic fractions were prepared using a nuclear/cytosolic fractionation kit (Biovision, Inc.). The cytosolic extracts (5 µg) of untreated and SA-treated cancer cells were incubated with 20 µM fluorogenic substrates for various proteolytic activities of the proteasome, Suc-Leu-Leu-Val-Tyr-AMC (for proteasomal chymotrypsin-like activity), benzyloxycarbonyl(Z)-Leu-Leu-Glu-AMC (for proteasomal PGPH activity) and Z-Gly-Gly-Arg-AMC (for proteasomal trypsin-like activity) for 90 min at 37 °C in 100 µL of assay buffer (20 mM Tris-HCl, pH 8.0). The reaction mixture was diluted to 200 µL with the assay buffer followed by the measurement of hydrolyzed 7-amido-4-methyl-coumarin (AMC) using a Varian CARY Eclipse Fluorescence spectrophotometer (Varian Inc, Palo Alto, CA), with excitation at λ = 360 nm and emission λ = 460 nm.
Analysis of mRNA level of apoptosis- and cell-cycle-regulatory genes
Analysis of the expression of cell cycle and apoptosis controlling genes was performed on cells from control and SA-treated groups with real-time polymerase chain reaction (RT-PCR) using an ABI 7000 SDS system (Applied Biosystems) and the comparative ΔΔCt method (Abaza et al., Citation2008a). Ready-made assays-on-demand target gene expression probes and primers were obtained from Applied Biosystems. The targets and their assay numbers for cell-cycle regulatory genes were as follows: cdk1 (Hs00364293_m1), cdk2 (Hs00608082_m1), cdk4 (Hs00364847_m1), cdk6 (Hs00608037_m1.) and cdk7 (Hs00361484_m1); cyclin A1 (Hs00171105_m1), cyclin B1 (Hs00259126_m1), cyclin C (Hs00193177_m1), cyclin E1 (Hs01026542_m1), and cyclin H (Hs00236923_m1); p15 (Hs00394703_m1), p16 (Hs00233365_m1), p18 (Hs00176227_m1), p19 (Hs00176481_m1), p21 (Hs00355782_m1), and p27 (Hs00197366_m1); and p53 (Hs00153340_m1).
The targets and their assay numbers for pro-apoptotic, anti-apoptotic and caspase genes were as follows: Bad (Hs188930_m1), Bak (Hs00832876_m1), Bax (Hs00180269_m1), Bid (Hs00609632_m1), Bim (Hs00375807_m1), Apaf1 (Hs00559441_m1), and Smac (Hs00219876_m1); AIF (Hs00269879_m1), cIAP-1 (Hs0023691_m1), c-IAP-2 (Hs00985029_m1), Bcl2 (Hs00608023_m1), and FLIP (Hs00354474_m1); casp2 (Hs00154242_m1), casp3 (Hs00234387_m1), casp6 (Hs00154250_m1), casp7 (Hs00169152_m1), casp8 (Hs01018151_m1), and casp9 (Hs00154260_m1); and GAPDA. The later was used as an endogenous control to normalize the expression values for each sample.
For the comparative Ct method, we performed a two-step RT-PCR using cDNA and carried out real-time quantitation using the target gene expression assays and Taqman universal master mix (Applied Biosystems). Cancer cells SW1116 were plated (1 × 105 cells mL−1) into 24-well plates in a non-CO2 incubator for 18 h. Cells were treated with SA (4.07 mg mL−1) for 24 h; mRNA was extracted using nucleospin RNAII ready to use system (Macherey-Nagel). For the RT reaction, 200 ng µL−1 of mRNA was used. First, DNA was eliminated with DNase-I treatment for 20 min at 25 °C, followed by heat inactivation for 10 min at 65 °C. The cDNA synthesis was performed using a high-capacity cDNA reverse transcription kit (Applied Biosystem) according to manufacturer's instructions. For each sample, 2.5 μL of cDNA and 12.5 μL of Taqman universal master mix (2×) were used, and the volume was made to 25 μL with nuclease-free water on an optical 96-well reaction plate (Applied Biosystems). Real-time RT-PCR was performed on an ABI 7000 SDS system, using ABI Prism's SDS collection software version 1.1 (Applied Biosystems), and as per Taqman universal master mix manufacturer’s protocol: step 1 at 95 °C for 10 min; step 2 at 94 °C for 15 s and step 3 at 60 °C for 1 min. The amount of target, normalized to an endogenous reference and relative to a calibrator (untreated), was given by 2−ΔΔCt and the log comparative Ct was presented graphically.
Sensitivity of colorectal cancer cells to the combined treatment with SA and standard anticancer drugs
The potential of SA to sensitize human colorectal cancer cells to standard chemotherapeutic drugs was investigated with some modifications as described previously (Abaza et al., Citation2008b). Chemotherapeutic drugs, namely camptothecin (∼95%), 5FU (≥99%), oxaliplatin (≥98%), taxol (≥95%), vincristine (≥95%), etoposide (≥98%), ellipticine (97%), amsacrine (≥98%), aphidicolin (≥98%), doxorubicin (≥98.0%), vinblastine (≥96%) were procured from Sigma Aldrich, and homoharringtonine (≥96%) was from ENZO Lifesciences (Ann Arbor, MI). Cancer cells SW1116 and SW837 were plated (27 × 103 cells/well) into a 96-well plate at 37 °C in a non-CO2 incubator. At 18 h after starting the culture, the cells were treated for 24 h with various concentrations of camptothecin (115 × 10−11 to 0.5 × 10−3 M), 5FU (84 × 10−10 to 3.5 × 10−3 M), doxorubicin (110 × 10−12 to 4.3 × 10−5 M), oxaloplatin (80 × 10−10 to 3 × 10−3 M), taxol (32 × 10−10 to 1.3 × 10−2 M), vinblastine (54 × 10−9 to 2 × 10−2 M), vincristine (32 × 10−10 to 1.3 × 10−3 M), etoposide (54 × 10−9 to 2 × 10−2 M), ellipticine (26 × 10−9 to 1.1 × 10−2 M), amsacrine (16 × 10−9 to 6 × 10−3 M), homoharrigtonine (24 × 10−12 to 1 × 10−5 M) and aphidicolin (35 × 10−12 to 1.5 × 10−5 M). The drug was removed, the cells were washed with HBSS (Hanks balanced salt solution), treated with SA (1.1 or 1.6 mg mL−1) for 48 h and cell growth was monitored by the MTT assay.
Interaction of SA with standard chemotherapeutic drugs
In order to evaluate the type of interaction between SA and standard chemotherapeutic drugs in human colorectal cancer, the cells were treated with SA (1.1 or 1.6 mg mL−1) and standard chemotherapeutic drugs individually and in combination. The type of drug interaction was determined using the following relationships: SFA + B > (SFA) × (SFB) =antagonistic, SFA + B = (SFA) × (SFB) = additive and SFA + B <(SFA) × (SFB) = synergistic, where SF is the surviving fraction, and A and B indicate the agent used alone, while (A + B) refers to the agents used in combination (Abaza et al., Citation2008b; Lam et al., Citation2001).
Statistical analyses
Results were expressed as the mean ± SEM. Statistical analyses were performed with SPSS version 18 (SPSS, Chicago, IL). Statistical significance of differences between the control and treated groups were determined by one-way ANOVA using LSD. p Values < 0.05 were considered statistically significant.
Results
Isolation and identification of SA from T. aucheriana
The methanol crude extract of T. aucheriana was fractionated using a glass column packed with 200–300 mesh silica gel in toluene. Fractionation was carried out with chloroform–methanol mixtures and the crude product was crystallized from ethyl acetate yielding 36.9 mg SA from 4 g of the methanol crude extract of T. aucheriana (1% yield). Spectral data including UV, IR, MS, 1H-NMR and 13C-NMR confirmed that the purified product was 4-hydroxy-3,5-dimethoxy-benzoic acid (SA). SA identity was further confirmed by comparison with a commercially available sample purchased from Sigma-Aldrich.
Antimitogenic effect of SA
SA significantly inhibited (p ≤ 0.0001) the growth of human colorectal cancer cells compared to normal human fibroblast that showed a maximum growth inhibition of ≤20% after 24–72 h of SA treatment (). SA inhibited colorectal cancer cells in a time- and dose-dependent manner with IC50 values of 0.95–1.15 mg mL−1 and 1.13–1.2 mg mL−1 for SW1116 and SW837, respectively, after 24–72 h of treatment. Treatment of colorectal cancer cells with SA (7.5 mg mL−1) for 24 h induced cellular morphological changes (). The results of growth inhibition were confirmed by the inhibition of colony formation (). SA (7.5 mg mL−1) significantly inhibited the colony formation by SW1116 (p ≤ 0.02) and SW837 (p ≤ 0.0001) compared to both untreated cell lines. The antimitogenic effect of SA on cancer cells was comparable with those produced by the chemotherapeutic drugs used in this study (). The concentrations used in the subsequent studies were within the IC50 values and the other concentration was higher than the IC50 values (1.6 mg mL−1). Although this concentration of SA was slightly higher than that found in human blood, its toxicity toward normal human fibroblast cells was found to be very low ().
Figure 1. Antimitogenic activity of SA on human colorectal cancer and normal human fibroblast cells. (a) Time- and dose-dependent antimitogenic effect of SA on cancer cell lines SW1116 and SW837 and normal human fibroblast CRL1554, (b) Morphological changes of cancer cells treated with SA, (c) Colony formation by untreated and SA-treated cancer cell lines.
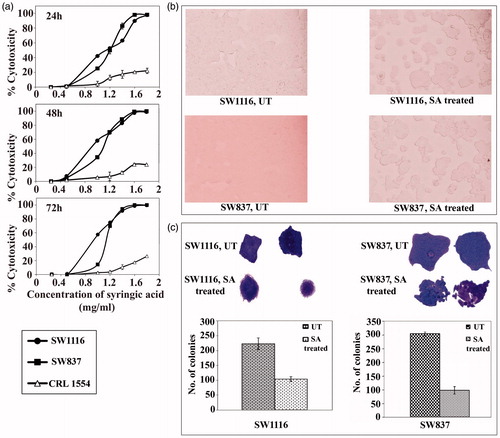
Cell-cycle phase distribution after treatment with SA
A 24 h treatment of cancer cells with SA (4.07 mg mL−1) resulted in an accumulation of SW1116 cells in S-phase (52.9% versus 20.5% for untreated, UT) and G2/M phase (15.1% versus 13.2% for UT) (). Similar results were obtained with SW837 cells, which showed a marked increase in SW837 cells in S-phase (56% versus 31.5% for UT) and G2/M-phase (11.6% versus 6.7% with UT). A 48 h treatment of SW1116 with SA at the same concentration showed growth-arrested cells in the G1/Go phase (54.5% versus 48.6% for UT) and G2/M phase (14.4% versus 9.2% for UT). On the other hand, SW837 cells were growth-arrested in the G2/M phase (12.8% versus 2.4% for UT). An exposure to SA at the same concentration, for 72 h showed growth-arrested SW1116 in S-phase (42.4% versus 31.1% for UT) and G2/M phase (13.8% versus 9.0% for UT). Similar results were obtained with SW837 cells, which was growth arrested in S-phase (28% versus 26.3% for UT) and G2/M (12.9% versus 4% for UT) (). Treatment of cancer cells with SA increased the percentage of apoptotic cells in a time-dependent manner ().
Figure 2. Analysis of cell cycle of cancer cells treated with SA. Cancer cell lines SW1116 and SW837 were processed as previously described (Abaza et al., Citation2008a). At least three samples were analyzed and 20 000 events were scored for each sample. The vertical axis represents the relative number of events and the horizontal axis represents the fluorescence intensity.
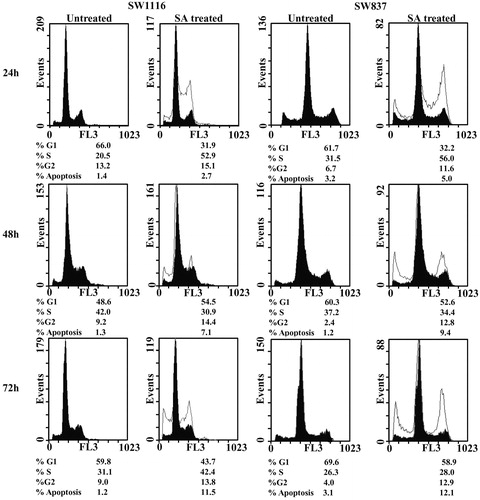
Induction of apoptosis and inhibition of NFκB DNA-binding and proteasome activities
An increase in the percentage of sub-G1 phase implied an increase in the percentage of apoptotic cells (). Apoptotic cell death is accompanied with modifications in plasma membrane structure by surface exposure of phosphatidylserine (PS), while the membrane integrity remains unaffected. Surface exposed PS can be detected by its affinity for annexin V, a phospholipid-binding protein in the presence of calcium. Treatment of SW1116 cells with SA (4.07 mg mL−1) for 24 h induced 3% early apoptosis, 10.9% late apoptosis and 4.5% necrosis. Similar results were obtained with SW837 cells treated with SA, which induced 3% early apoptosis, 6.1% late apoptosis and 4.8% necrosis ().
Figure 3. Assessment of apoptosis, NFκB activity and proteasome activities in cancer cells treated with SA. (a) Assessment of apoptosis. B1: percentage of necrotic cells, B2: percentage of late apoptotic cells, B3: percentage of living cells and B4: percentage of early apoptotic cells. (b) Monitoring the NFκ-B activity in nuclear extracts of cancer cells, (c) monitoring the 26S proteasomal activities in cancer cell extracts.
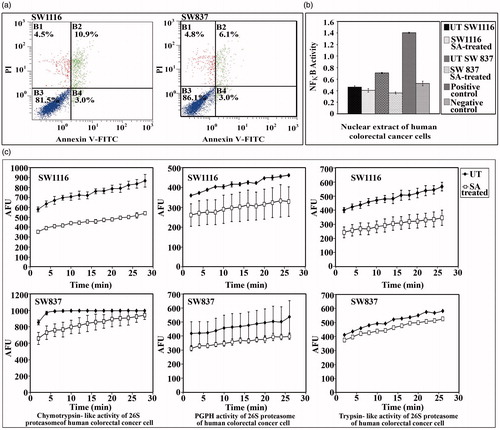
DAN-binding activity of NFκB showed a non-significant decrease (p > 0.129) in the DNA-binding activity of NFκB in SW1116 cells. Meanwhile, a marked significant decrease (p ≤ 0.0001) in the DNA-binding activity of NFκB was observed when SW837 cells were treated with SA (). The effect of SA on the various proteolytic activities of ubiquitin–proteasome system (UPS) was monitored. SA significantly inhibited the chymotrypsin-like activity (p ≤ 0.0001), the PGPH activity (p ≤ 0.0001) and the trypsin-like activity (p ≤ 0.002) of the 26S proteasome in the cytosolic extract of SW1116 cells (). A significant inhibition of the PGPH and chymotrypsin-like activities (p ≤ 0.0001) was observed with SA, which slightly affected the trypsin-like activity of the 26S proteasome (p ≤ 0.4) in the cytosolic extract of SW837 cells ().
Inhibition of the invasion and angiogenesis of colorectal cancer cells by SA
The results summarized in indicate the ability of SA to inhibit the invasion of colorectal cancer cells through a thin layer of ECMatrixEM that occludes the membrane pores and blocks non-invasion cells from migrating through ECM cells. On the other hand, the invasive layer migrated through the ECM layer and clung to the bottom of the polycarbonate membrane. The number of cells at the polycarbonate membrane bottom markedly decreased after treatment with SA () compared with the control (). In the anti-angiogenic assay, untreated colorectal cancer cells exhibited their ability to grow and tended to produce tubule formation. Meanwhile, colorectal cancer cells treated with SA were mostly deceased (data not shown).
Genes controlling cell cycle and apoptosis in colorectal cancer cells-treated with SA
Our data show that SA differentially down-regulated the expression of Cdk4, Cdk6 and cyclins B, C, E1, H and up-regulated the expression of p19, p21 and p27 (). SA differentially up-regulated the expression of the pro-apoptotic genes, including Bax, Bak, Bad, Bid, Bim, Apaf1, AIF Smac, caspase-2, 3, 6, 7, 8 and 9. Contrarily, SA down-regulated the expression of anti-apoptotic genes, such as cIAP-1, c-IAP-2, Bcl2 and FLIP ().
Figure 5. Assessment of the mRNA expression of the genes controlling cell cycle and apoptosis in cancer cells-treated with SA. (a) Assessment of the mRNA expression of cyclin-dependent kinase, cyclin and cyclin-dependent kinase inhibitors genes in cancer cell line SW1116 treated with SA. (b) Assessment of the mRNA expression of pro-apoptotic, anti-apoptotic and caspase genes in cancer cell line SW1116-treated with SA.
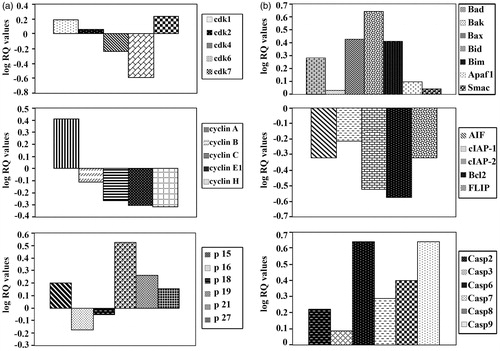
Cytotoxicity potentiation of standard chemotherapeutic drugs by SA
SA catalyzed sensitization of human colorectal cancer cells to standard chemotherapeutic drugs was evaluated. The IC50 and the sensitization ratios were used as a measure of the ability of SA to enhance the sensitivity of human colorectal cancer cell lines SW1116 () and SW837 () toward standard chemotherapeutic drugs acting through different mechanisms of action. The significance of potentiation induced by SA was evaluated from the statistical analyses of results summarized in and . These results demonstrate differential sensitization of colorectal cancer cells to standard chemotherapeutic drugs with a marked increase in their sensitivity to camptothecin (sensitization ratio, SR = 500, 1.1 mg/mL), 5FU (SR = 20 000, 1.6 mg mL−1), doxorubicin (SR = 210, 1.6 mg mL−1), taxol (SR = 3134, 1.6 mg mL−1), vinblastine (SR = 1000, 1.1 mg mL−1), vincristine (SR = 130, 1.6 mg mL−1) and amsacrine (SR = 107.3, 1.6 mg mL−1).
Table 1. IC50, IC70, IC80 values, sensitization ratios and P-values of the combined treatment with SA and standard chemotherapeutic drugs in human colorectal cancer cell line SW1116.
Table 2. IC50–IC80, sensitization ratios and p-values of the combined treatment with SA and standard chemotherapeutic drugs in human colorectal cancer cell line SW837.
SA and chemotherapeutic drug interaction
The results summarized in and imply differences in cancer cell lines in response to combination of standard chemotherapeutic drugs and SA. The combination drugs displayed additive, synergistic or antagonistic antimitogenic effects on colorectal cancer cells.
Table 3. Analysis of the combined effects of SA and standard chemotherapeutical drugs on human colorectal cell lines.
Table 4. Analysis of the combined effects of SA and conventional chemotherapeutical drugs on human colorectal cell lines.
Discussion
Phenolic compounds have attracted considerable attention during the past few years due to their many potential health benefits (Duthie & Crozier, Citation2000). However, few studies have reported the possible role of phenolic acids in cancer prevention and antigenotoxicity (Clifford, Citation2000). The diverse biological activities and the protection against hepatic carcinoma by Tamarix gallica L. (Tamaricaceae) methanol extracts prompted us to test the antimitogenic activity of T. aucheriana. The present study was focused on anticancer activity, and the mechanism of action of SA, a metabolite of Tamarix aucheriana. So far, no study has been reported about the anticancer potential of SA on human colorectal cancer cells.
SA showed a time- and dose-dependent antimitogenic effect toward human colorectal cancer cells (). Compared with untreated colorectal cancer cells, a significant reduction in colony formation in SW1116 and SW837 cells treated with SA () reflected the potential antimitogenic activity of the phenolic acid. One of the important criteria for anticancer drugs is the ability to selectively kill cancer cells with minimal damage to the normal cells. Our data show an insignificant effect of SA on normal human fibroblasts (), and this prompted us to explore the activity of SA in detail. Our results supplement previous studies on the growth inhibitory effect of natural phenolic acids against different types of cancer cells (Ho et al., Citation2010; Yin et al., Citation2009).
Disruption of the cell-cycle process causes an imbalance between cell proliferation and cell death, leading to cancer development. Thus, the cell cycle could serve as a target for anticancer agents to inhibit the uncontrolled proliferation of cancer cells and initiate their apoptosis. In the current study, SA treatment of human colorectal cancer cell lines showed a time-dependent alteration in the colorectal cancer cell distribution in different phases of the cell cycle. SA markedly arrested the growth of both SW1116 and SW837 in S-phase/G2-M phase, G1-phase/G2-M phase and S-phase/G2-M phase after 24, 48, and 72 h of treatment, respectively. SA also exhibited a marked proapoptotic effect in both SW1116 and SW837 cells as determined by annexin V-FITC and PI staining (). Our results are supported by numerous reports indicating that phenolic acids have a great potential in cell-cycle arrest in different phases and apoptosis (Chen et al., Citation2009; Hsu et al., Citation2011; Lo et al., Citation2010; Madlener et al., Citation2007; Ou et al., Citation2010; Reddivari et al., Citation2010; Tseng et al., Citation2000; You & Park, Citation2010, Citation2011).
Many different cancer types, including human colorectal cancer, possess high NFκB activity. In the present study, cancer cell line SW1116 treated with SA showed a non-significant decrease (p ≥ 0.13) in the DNA-binding activity of NFκB. Meanwhile, SW837 cells treated with SA exhibited a significant decrease (p ≤ 0.0001) in the DNA-binding activity of NFκB (). SA methylester has been reported to significantly reduce the transcriptional activity of NFκB, the DNA-binding activity of NFκB and levels of p65 in human and mouse prostate cancer cells. Gallic acid (GA), another phytophenolic acid, has been reported to inhibit the protein level of NFκB-p65 in PC-3 human prostate cancer cells (Liu et al., Citation2011). DNA-binding and transcriptional activities of NFκB are also inhibited in caffeic acid phenethylester (CAPE)-treated jurkat cells, demonstrating an excellent potential of phenolic acids for inhibiting or lowering NFκB activity (Lo et al., Citation2010).
Having established the activity of SA as an inhibitor for NFκB, attention was focused on the effect of SA on various proteolytic activities of ubiquitin proteasome system (UPS). SA significantly (p ≤ 0.0001) inhibited the chymotrypsin-like activity, the PGPH activity (p ≤ 0.0001) and trypsin-like activity (p ≤ 0.002) of the 26S proteasome in the cytosolic extract of SW1116 (). At the same time SA significantly (p ≤ 0.0001) inhibited the PGPH and chymotrypsin-like activities of 26S proteasome of human colorectal cancer cells SW837. However, SA non-significantly (p ≤ 0.4) affected the trypsin-like activity of SW837 cells (). A virtual binding affinity study on SA for 20S yeast proteasome using SYBL 8.0 Surflex-Dock was conducted. The results showed that SA had a binding score toward 20S yeast proteasome comparable with polyphenols like chrysin, hesperetin and genistein, and higher than apigenin, kaempferol and sinapinic acid. All these except sinapinic acid have a common 5,7-dihydroxy function on a flavonoid or isoflavonoid molecular structure and have a close similarity with SA in inhibiting chymotrypsin-like activity of proteasome in different types of cancer cells (Wu & Fang, Citation2010). We have studied the expression of two known substrates of proteasome, Bax and p27kip, and as expected, their expression dramatically increased upon treatment with SA, putting this molecule under the spotlight for its proteolytic activities of UPS.
The ability of SA to inhibit colorectal cancer cells through a thin layer of ECMatrixEM (Billerica, MA) was also examined. The number of cells at the bottom of the polycarbonate membrane markedly decreased compared with the control after treatment with SA (). Many phenolic compounds are known to act comparably highlighting the potential of phenolic acid as worthy inhibitors of cancer cells (Ho et al., Citation2010; Lin et al., Citation2011; Lirdprapamongkol et al., Citation2005, Citation2009; Liu et al., Citation2011; Lu et al., Citation2010). The results supplemented the anti-angiogenic potential of SA towards human colorectal cancer cells. The untreated colorectal cancer cells grew on matrigel and tended to produce a tubule formation. Meanwhile, most of the colorectal cancer cells treated with SA did not survive strengthening the idea that phenolic acid was a good angio-preventive agent (Lirdprapamongkol et al., Citation2009; Lu et al., Citation2010).
Inhibition of cell cycle and an induction of apoptosis in cancer cells are two major goals in cancer treatment. Therefore, we examined the expression of genes controlling both cell cycle and apoptosis in human colorectal cancer cells treated with SA that down-regulated the expression of Cdk4, Cdk6 and cyclins B, C, E1 and H. On the other hand, SA up-regulated the expression of p19, p21Cip1/Waf1 and p27kip1 (). Numerous similar reports about other phenolic acids have appeared in the literature (Hsu et al., Citation2011; Ou et al., Citation2010; Raina et al., Citation2008; Won-Kyoung et al., Citation2008).
The differential up-regulation of the expression of the pro-apoptotic genes, including Bad, Bak, Bax, Bid, Bim, Apaf1 and Smac () with SA and a differential down-regulation of the anti-apoptotic genes such as cIAP-1, c-IAP-2, Bcl2 and FLIP, as well as the proapoptotic gene AIF () by SA encouraged additional investigations of this simple but important phenolic acid. Thus, SA differentially up-regulated the expression of caspase genes, including caspase-2, 3, 6, 7, 8 and 9 (). The regulatory mechanism of SA-induced apoptosis is thought to involve a cascade of caspases, including initiator caspase-8 of the extrinsic pathway and caspase-9 of the intrinsic pathway of apoptosis. These initiator caspases could activate effector caspase-3, -6 and -7. SA induced mitochondrial membrane depolarization and release of SMAC/DIABLO from mitochondria into the cytosol. This type of regulatory mechanism in the control of cancer has been reported for other phenolic acids reflecting on their potential as anticarcinogenic compounds (Chia et al., Citation2010; Lo et al., Citation2010; Tseng et al., Citation2000; Won-Kyoung et al., Citation2008; Yin et al., Citation2009).
Over-expression of BcL2, BcL-xL or other anti-apoptotic homologues or loss of pro-apoptotic Bax, Bak is a common feature of human tumors (Mro'zek et al., Citation2003). SA elevated pro-apoptotic and depletes anti-apoptotic genes. BcL2 is known to inhibit the release of cyto-c and SMAC/DIABLO in human tumor cell lines (Adrain et al., Citation2001). SA-induced BcL2 depletion may be necessary to facilitate the release of cyto-c and SMAC/DIABLO from mitochondria that adheres to IAPs and inhibits their caspase-binding activity. From the data accumulated, we predict that the antimitogenic activity of the multifaceted molecule, SA, is associated with (1) induction of apoptosis, (2) induction of cell-cycle arrest, (3) inhibition of migration and angiogenesis, (4) suppression of chymotrypsin-like, caspase-like and trypsin-like proteolytic activities of proteasome, and (5) blockage of NFκB signaling.
There is a need to develop new therapeutics to overcome current problems of drug resistance, high toxicity and lack of target specificity for anticancer agents. Use of modifiers such as liposomes, nanoparticles, is receiving attention by many researchers (Duthie & Crozier, Citation2000). We studied the potential of SA to sensitize human colorectal cancer cells to conventional chemotherapeutic drugs. It was found that SA (1.1 and 1.6 mg mL−1) differentially increased the sensitivity of SW1116 and SW837 cells to standard chemotherapeutic drugs with diverse mechanisms of action. Thus, SA showed a marked increase in the sensitivity of colorectal cancer cells to camptothecin (SR = 500), 5FU (SR = 20 000), doxorubicin (SR = 210), taxol (SR = 3134), vinblastine (SR = 1000), vincristine (SR = 130) and amsacrine (SR = 107). The combined treatment with SA and tested chemotherapies showed synergistic and/or additive cytotoxicities on human colorectal cancer cells ( and ). Combined therapy promotes synergism among the different drugs against cancer cells and suppresses drug resistance through distinct mechanisms of action.
Figure 6. SA potentiates the cytotoxicity of standard chemotherapeutic drugs on cancer cell line SW1116. Cancer cells SW1116 were plated into a 96-well plate at 37 °C in a non-CO2 incubator. 18 h after starting the culture, the cells were treated for 24 h with various concentrations of chemotherapies. Then the drug was removed and the cells were washed, treated with SA for 48 h and cell proliferation was monitored by the MTT assay.
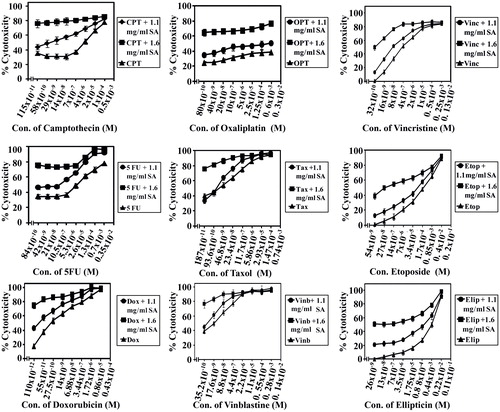
Figure 7. SA potentiates the cytotoxicity of conventional chemotherapeutic drugs on cancer cell line SW837. Cancer cells SW837 were plated into a 96-well plate at 37 °C in a non-CO2 incubator. 18 h after starting the culture, the cells were treated for 24 h with various concentrations of chemotherapies. Then the drug was removed and the cells were washed, treated with SA for 48 h and cell proliferation was monitored by MTT assay.
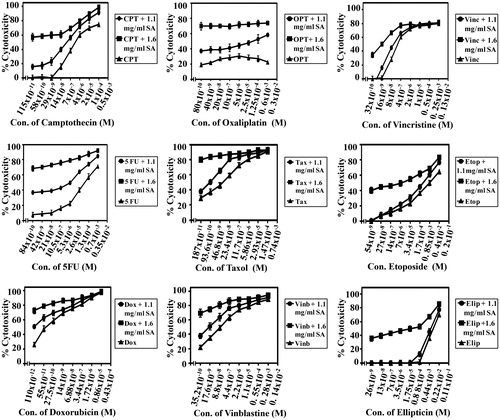
Figure 8. SA potentiates the cytotoxicity of amsacrine, homohorrigtonine and aphidicolin on cancer cell lines SW1116 and SW837. Cancer cell lines SW1116 (a) and SW837 (b) were plated into 96-well plates. 18 h after starting the culture, the cells were treated for 24 h with various concentrations of amsacrine, homoharrigtonine and aphidicolin. Then the drug was removed and the cells were washed, treated with SA for 48 h and cell proliferation was monitored by the MTT assay. The effect of SA alone on the growth of SW1116 and SW837 was also evaluated (c).
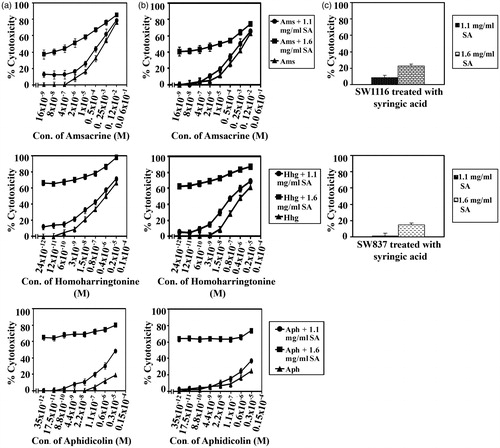
In conclusion, SA exerted multifaceted chemotherapeutic effect by inducing cell-cycle arrest, apoptosis, decreasing cell proliferation, cell migration, angiogenesis, inhibiting the DNA-binding activity of NFκB and proteasome activity. The ability of SA to sensitize colorectal cancer cells to conventional chemotherapeutic drugs may correlate well with its ability to inhibit commonly reported molecular targets.
Declaration of interest
There is no conflict of interest.
Acknowledgements
This study was supported by Kuwait University research grant # SL05-04. The investigators thankfully acknowledge the assistance of Science Analytical Facilities (SAF) (General Facility Project # GS01/01, GS03/01, and GS01/03) for spectral data.
References
- Abaza MS, Al-Saffar A, Al-Sawan S, Al-Attiyah R. (2008a). c-myc Antisense oligonucleotides sensitize human colorectal cancer cells to chemotherapeutic drugs. Tumor Biol 29:287–303
- Abaza MS, Bahman AM, Al-Attiyah RJ. (2008b). Roscovitine synergizes with conventional chemo-therapeutic drugs to induce efficient apoptosis of human colorectal cancer cells. World J Gasteroenterol 14:5162–75
- Adrain C, Creagh EM, Martin SJ. (2001). Apoptosis-associated release of Smac/DIABLO from mitochondria requires active caspases and is blocked by Bcl-2. EMBO J 20:6627–36
- Boulos L. (1983). Medicinal Plant of North Africa. Algonac, Michigan: Publications Inc
- Cappell MS. (2007). From colonic polyps to colon cancer: Pathophysiology, clinical presentation, screening and colonoscopic therapy. Minerva Gastroenterol Dietol 53:351–73
- Chen HM, Wu YC, Chia YC, et al. (2009). Gallic acid, a major component of Toona sinensis leaf extracts, contains a ROS-mediated anti-cancer activity in human prostate cancer cells. Cancer Lett 286:161–71
- Chia YC, Rajbanshi R, Calhoun C, Chiu RH. (2010). Anti-neoplastic effects of gallic acid, a major component of Toona sinensis leaf extract, on oral squamous carcinoma cells. Molecules 5:8377–89
- Clifford MN. (2000). Chlorogenic acids and other cinnametes-nature occurrence, dietary burden, absorption and metabolism. J Sci Food Agric 80:1033–43
- Daoud HS, Al-Rawi A. (1985). Flora of Kuwait, Dicotyledoneae. Vol. 1. London: KPI Publishers
- Duthie G, Crozier A. (2000). Plant-derived phenolic antioxidants. Curr Opin Clin Nutr Metab Care 3:447–51
- Espin C, Garcia-Conesa MT, Tomas-Barberan FA. (2007). Nutraceuticals: Facts and fiction. Phytochemistry 68:2986–3008
- Gaston B. (1998). La Grande Flore en Couleurs, 1st ed. Suisse, France: Belgique et pays voisins
- Ghazanfar SA. (1994). Handbook of Arabian Medicinal Plants. Boca Roton: CRC Press
- Heim KE, Tagliaferro AR, Bobilya DJ. (2002). Flavonoid antioxidants: Chemistry, metabolism and structure–activity relationships. J Nutr Biochem 13:572–84
- Herrmann K. (1989). Occurrence and content of hydroxycinnamic and hydroxybenzoic acid compounds in foods. Crit Rev Food Sci Nutr 28:315–47
- Hertog MG, Feskens EJ, Hollman PC, et al. (1993). Dietary antioxidant flavonoids and risk of coronary heart disease: The Zutphen Elderly Study. Lancet 342:1007–11
- Ho HH, Chang CS, Ho WC, et al. (2010). Anti-metastasis effects of gallic acid on gastric cancer cells involve inhibition of NF-kappaB activity and down-regulation of PI3K/AKT/small GTPase signals. Food Chem Taxicol 48:2508–16
- Hsu JD, Kao SH, Ou TT, et al. (2011). Gallic acid induces G2/M phase arrest of breast cancer cell MCF-7 through stabilization of p27(Kip1) attributed to disruption of p27(Kip1)/Skp2 complex. J Agric Food Chem 59:1996–2003
- Lam PK, To EW, Chan ES, et al. (2001). In vitro modulation of head and neck cell growth by human recombinant interferon α and 13-cis-retinoic acid. Br J Biomed Sci 58:226–9
- Lila MA. (2007). From beans to berries and beyond: Teamwork between plant chemicals for protection of optimal human health. Ann NY Acad Sci 114:372–80
- Lin HH, Chen JH, Chou FP, Wang CJ. (2011). Protocatechuic acid inhibits cancer cell metastasis involving the down-regulation of Ras/Akt/NF-κB pathway and MMP-2 production by targeting RhoB activation. Br J Pharmacol 162:237–54
- Lirdprapamongkol K, Kramb JP, Suthiphongchai T, et al. (2009). Vanillin suppresses metastatic potential of human cancer cells through PI3K inhibition and decreases angiogenesis in vivo. J Agric Food Chem 57:3055–63
- Lirdprapamongkol K, Sakurai H, Kawasaki N, et al. (2005). Vanillin suppresses in vitro invasion and in vivo metastasis of mouse breast cancer cells. Eur J Pharm Sci 25:57–65
- Liu KC, Huang AC, Wu PP, et al. (2011). Gallic acid suppresses the migration and invasion of PC-3 human prostate cancer cells via inhibition of matrix metalloproteinase-2 and -9 signaling pathways. Oncol Rep 26:177–84
- Lo C, Lai TY, Yang JH, et al. (2010). Gallic acid induces apoptosis in A375.S2 human melanoma cells through caspase-dependent and -independent pathways. Int J Oncol 37:377–85
- Lu Y, Jiang F, Jiang H, et al. (2010). Gallic acid suppresses cell viability, proliferation, invasion and angiogenesis in human glioma cells. Eur J Pharmacol 641:102–7
- Madlener S, Horvath Z, Saiko P, et al. (2007). Gallic acid inhibits ribonucleotide reductase and cyclooxygenases in human HL-60 promyelocytic leukemia cells. Cancer Lett 245:156–62
- Miyata T. (2007). Pharmacological basis of traditional medicines and health supplements as curatives. J Pharmacol Sci 103:127–31
- Mro'zek A, Petrowsky H, Sturm I, et al. (2003). Combined p53/Bax mutation results in extremely poor prognosis in gastric carcinoma with low microsatellite instability. Cell Death Diff 10:461–7
- Nishino H, Satomi Y, Tokuda H, Masuda M. (2007). Cancer control by phytochemicals. Curr Pharm Des 13:3394–9
- Ou TT, Wang CJ, Lee YS, et al. (2010). Gallic acid induces G2/M phase cell-cycle arrest via regulating 14-3-3β release from Cdc25C and Chk2 activation in human bladder transitional carcinoma cells. Mol Nutr Food Res 54:1781–90
- Raina K, Rajamanickam S, Deep G, et al. (2008). Chemopreventive effects of oral gallic acid feeding on tumor growth and progression in TRAMP mice. Mol Cancer Ther 7:1258–67
- Randhir R, Lin YT, Shetty K. (2004). Phenolics, their antioxidant and antimicrobial activity in dark germinated fenugreek sprouts in response to peptide and phytochemical elicitors. Asia Pac J Clin Nutr 3:295–307
- Reddivari L, Vanamala J, Safe SH, Miler JC Jr. (2010). The bioactive compounds alpha-chaconine and gallic acid in potato extracts decrease survival and induce apoptosis in LNCaP and PC3 prostate cancer cells. Nutr Cancer 62:601–10
- Rowley PT. (2005). Inherited susceptibility to colorectal cancer. Annu Rev Med 56:539–54
- Sehrawat A, Sultana S. (2006a). Tamarix gallica L. [Tamaricaceae] ameliorates thioacetamide-induced hepatic oxidative stress and hyperproliferative response in Wister rates. J Enzyme Med Chem 21:215--23
- Sehrawat A, Sultana S. (2006b). Evaluation of possible mechanisms of protective role of Tamarix gallica against DEN initiated and 2-AAF promoted hepatocarcinogenesis in male Wistar rats. Life Sci 79:1456--65
- Sharma SK, Parmar VS. (1998). Novel constitutes of Tamarix species. J. Sci Ind Res 57:873–90
- Smith RA, Cokkinides V, Brawley OW. (2008). Cancer screening in the United States, a review of current American Cancer Society guidelines and cancer screening issues. Cancer J Clin 8:161–79
- Tseng TH, Kao TW, Chu CY, et al. (2000). Induction of apoptosis by hibiscus protocatechuic acid in human leukemia cells via reduction of retinoblastoma (RB) phosphorylation and Bcl-2 expression. Biochem Pharmacol 60:307–15
- Won-Kyoung S, Chi-Heum C, Sabarish R, et al. (2008). Induction of apoptosis by Hibiscus protocatechuic acid in human uterine leiomyoma cells. Korean J Gynecol Oncol 19:48–55
- Wu YX, Fang X. (2010). Apigenin, chrysin, and luteolin selectively inhibit chymotrypsin-like and trypsin-like proteasome catalytic activities in tumor cells. Planta Med 76:128–32
- Yin MC, Lin CC, Wu HC, et al. (2009). Apoptotic effects of protocatechuic acid in human breast, lung, liver, cervix, and prostate cancer cells: Potential mechanisms of action. J Agric Food Chem 57:6468–73
- You BR, Park WH. (2010). Gallic acid-induced lung cancer cell death is related to glutathione depletion as well as reactive oxygen species increase. Toxicol In Vitro 24:1356–62
- You BR, Park WH. (2011). The effects of mitogen-activated protein kinase inhibitors or small interfering RNAs on gallic acid-induced HeLa cell death in relation to reactive oxygen species and glutathione. J Agric Food Chem 59:763–71