Abstract
Context: Hypericum caprifoliatum Cham & Schlecht (Guttiferae) extracts have a potential antidepressant-like effect in rodents. However, the molecular mechanisms by which these extracts exert this effect remain unclear.
Objective: This study evaluated the effect of HC1, a fraction obtained from H. caprifoliatum enriched in phloroglucinol derivatives, on the Na+, K+ ATPase activity in mouse brain and verified the influence of veratrine on the effect of HC1 in the forced swimming test (FST).
Materials and methods: Veratrine (0.06 mg/kg) and HC1 (360 mg/kg) were given alone or combined i.p. 60 and p.o. 30 min, respectively, before FST. The effect of single and repeated administration (once a day for 3 consecutive days) of HC1 (360 mg/kg) on Na+, K+ ATPase activity was evaluated ex vivo in the cerebral cortex and hippocampus of mice subjected or not to FST.
Results: HC1 reduced the immobility time (103.15 ± 18.67 s), when compared to the control group (183.6 ± 9.51 s). This effect was prevented by veratrine (151.75 ± 22.19 s). Mice repeatedly treated with HC1 presented a significant increase in Na+, K+ ATPase activity, both in cerebral cortex (46 ± 2.41 nmol Pi/minċmg protein) and hippocampus (49.83 ± 2.31 nmol Pi/minċmg protein), in relation to the respective controls (30 ± 2.66 and 29.83 ± 2.31 nmol Pi/minċmg protein respectively).
Discussion and conclusion: The HC1 antidepressant-like effect on FST might be related to its capacity to inhibit Na+ influx. HC1 increases hippocampal and cortical Na+, K+ ATPase activities possibly through long-term regulatory mechanisms.
Introduction
Hypericum perforatum (Guttiferae) (St. John's wort) extracts have gained popularity as an alternative to conventional antidepressant drugs for treating mild to moderate forms of depressive disorders (Linde et al., Citation2011). In southern Brazil, 20 Hypericum species have been identified (Dall’Agnol et al., Citation2003); these species are rich in flavonoids and dimeric phloroglucinols conjugated with a filicinic acid moiety (Ferraz et al., Citation2002). These phloroglucinol derivatives were proposed as chemotaxonomic markers for the southern Brazilian species (Nör et al., Citation2004). Among the species studied, Hypericum caprifoliatum Cham & Schlecht showed promising results in the forced swimming test (FST), which predicts antidepressant activity (Daudt et al., Citation2000; Viana et al., Citation2005, Citation2008). However, the molecular mechanisms by which these H. caprifoliatum extracts exert this antidepressant-like effect remains unclear.
Previous work by our group has shown that a cyclohexane extract of H. caprifoliatum and its enriched phloroglucinol fraction (HC1) inhibit the neuronal uptake of dopamine, noradrenaline and serotonin without direct interaction with their respective site on neuronal carriers, suggesting that H. caprifoliatum mechanism of action involves neurotransmitters transport in general (Viana et al., Citation2005). This hypothesis of mode of action has also been considered for hyperforin, a phloroglucinol derivative present in H. perforatum; Wonnemann & coworkers (Citation2000) have proposed that this compound exerts its antidepressant action by mechanisms dependent on Na+ channels.
The Na+-gradient is primarily maintained by Na+, K+ ATPase (Grisar, Citation1984). Both mania and bipolar depression have been associated with increased intracellular Na+ concentrations (Li & El-Mallakh, Citation2004) and a decrease in erythrocyte Na+, K+ ATPase activity (Herman et al., Citation2007; Looney & El-Mallakh, Citation1997). Animal models of despair are associated with decreased Na+, K+ ATPase activity and antidepressants concurrently prevent depressive-like behavior and increase Na+, K+ ATPase activity (de Vasconcellos et al., Citation2005). Rats exposed to chronic variable stress (CVS) developed despair-like endophenotypes and had decreased hippocampal and amygdalar Na+, K+ ATPase activity (Crema et al., Citation2010).
In this paper, we investigated if the Na+ balance is important for H. caprifoliatum antidepressant-like activity by assessing the effects of veratrine (a Na+ channel opener) on HC1 activity in the FST. We also evaluated the effect of acute and repeated administration of HC1 on Na+, K+ ATPase activity in the cerebral cortex and hippocampus of mice submitted or not to a swimming session.
Materials and methods
Plant material
The aerial parts of H. caprifoliatum were collected in the region of Morro Santana, in the state of Rio Grande do Sul, Brazil (October and December/2008). The voucher specimen was deposited at the herbarium of Universidade Federal do Rio Grande do Sul (ICN Bordignon, 3118 Herbário do Departamento de Botânica, Instituto de Biociências, UFRGS). The plant collection was authorized by IBAMA (Instituto Brasileiro do Meio Ambiente e dos Recursos Naturais Renováveis) (Protocol 02000.001717/2008-60).
Preparation of H. caprifoliatum extract
To prepare the lipophilic extract of H. caprifoliatum, dried and powdered plant material (200 g) was extracted with cyclohexane (plant/solvent ratio 1:5 w/v) by static maceration for 10 days, followed by evaporation to dryness under reduced pressure at 45 °C yielding an extract termed ECH. To obtain the purified extract, ECH was treated with acetone, according to Rocha et al. (Citation1995), producing an insoluble fatty residue, which was eliminated through a paper filter, yielding 12 g of a wax-free extract termed HCP.
HC1 purification
HCP was analyzed by preparative thin-layer chromatography (TLC) using silica gel GF254 (Merck, Dr. Whitehouse Station, NJ) as stationary phase and Godin’s reagent as chromogenic agent characteristic for phloroglucinol derivatives (Rocha et al., Citation1995). The extract showed a main spot (Rf 0.10–0.40), reactive to Godin’s reagent, which was removed by preparative TLC and termed HC1 (270 mg). It was characterized by NMR (1H and 13C, 400 MHz), as described by Daudt et al. (Citation2000), and constitutes a fraction enriched in phloroglucionol derivatives.
Animals
Behavioral and biochemical tests were carried-out with male CF1 mice (25–30 g) purchased from Fundação Estadual de Produção e Pesquisa em Saúde – RS (Brazil). The animals were housed in a group of eight in plastic cages (17 × 28 ×13 cm) and all animals were kept under a 12 h light/dark cycle (lights on at 7:00 a.m.) at a constant temperature of 23 ± 1 °C with free access to standard certified rodent diet and tap water. All experimental protocols were approved by The Animal Care Local Ethical Committee (CEUA UFRGS; Protocol 18203), and performed according to the Brazilian law (Brasil, 2008), which are in compliance with the European Communities Council Directive of 24 November 1986 (86/609/EEC) and International Guiding Principles for Biomedical Research Involving Animals (CIOMS, 1985).
Behavioral experiments
Forced swimming test
The FST was carried out according to Porsolt et al. (Citation1978) with minor modifications standardized and validated in our laboratory (Viana et al., Citation2005) as follows: mice were adapted to the laboratory conditions 1 h before being exposed to FST; the animals were individually forced to swim in a cylinder pool (10 cm diameter, 13 cm height, water at 22 ± 1 °C) and the total duration of immobility during a 6 min test was scored (in seconds) by a human observer blinded to the treatments. Each mouse was considered immobile when it remained floating motionless or make only the movements necessary to keep its head above water.
The HC1 fraction was suspended at a concentration of 36 mg/ml in saline with 2% of polysorbate 80. Different groups of animals were treated with HC1 (360 mg/kg, p.o.) or 2% polysorbate 80 solution in saline (NaCl 0.9%) 30 min before the test. This dose was chosen based on previous studies of our group (Viana et al., Citation2005, Citation2008). All treatments were administered at 10 ml/kg body weight.
In order to investigate the potential contribution of Na+ channels on the antidepressant-like effects of HC1, we investigated the influence of a pre-treatment with veratrine on this effect in the FST. Dose–response experiments were performed in the FST and locomotor activity apparatus to determine the appropriate dose of veratrine to be used in the interaction studies. A subactive dose was defined as the one that, by itself, did not reduce mobility in the FST and had no effect on locomotor activity. Veratrine was diluted in saline 0.9% and administered i.p. 60 min before the test at doses of 0.06, 0.125 and 0.5 mg/kg (Prica et al., Citation2008). A vehicle-treated group was introduced as a control. In the interaction studies, veratrine or vehicle were administered 60 min before the test, followed by HC1 that was administered 30 min before testing.
Locomotor activity
The measurement of spontaneous locomotor activity was performed in the open-field and was carried out according to Müller et al. (Citation2012). After the drug administration (pre-treatment/or treatment), mice were replaced in their holding cages for the required administration-test interval and then individually transferred to a transparent acrylic box measuring 45 × 30 × 30 cm with a dark bottom divided into 24 equal quadrants. The animals were observed during 15 min, and the first 5 min were used for the habituation of animals. The number of crossings was recorded by a human observer blinded to treatments. These animals were different from those used in the FST.
Biochemical experiments
Treatments and procedures
Acute treatment: Different groups of mice (n = 6) were treated with a single administration of HC1 (360 mg/kg, p.o.) or vehicle (saline with 2% of polysorbate 80).
Repeated treatment: Different groups of mice (n = 6) were treated once a day with HC1 (360 mg/kg, p.o.) or vehicle (saline with 2% of polysorbate 80), during 3 days.
Two hours after the single or the last repeated treatment, animals were killed by cervical dislocation and the hippocampus and cerebral cortex were removed.
In another set of experiments, different groups of animals were treated as described above and subjected to a single 6 min forced swimming session 1 h after the single or the last of the three treatments. One hour after the swimming session, the animals were killed by cervical dislocation and the hippocampus and the cerebral cortex were removed. All procedures (mice isolation, forced swimming session and decapitation) were performed in separated rooms.
Tissue preparation
The hippocampus or brain cortex were homogenized in 10 volumes (1:10, w/v) of 0.32 mM sucrose solution containing 5.0 mM HEPES and 1.0 mM EDTA, pH 7.5. The homogenates were centrifuged at 1000 × g for 10 min; the supernatants were removed for Na+, K+ ATPase activity determination.
Na+, K+ ATPase activity assay
The reaction mixture for the Na+, K+ ATPase assay contained 5.0 mM MgCl2, 80.0 mM NaCl, 20.0 mM KCl and 40.0 mM Tris-HCl, pH 7.4, in a final volume of 200 μL. After 10 min of pre-incubation at 37 °C, the reaction was initiated by the addition of ATP to a final concentration of 3.0 mM, and was incubated for 20 min. Controls were carried out under the same conditions with the addition of 1.0 mM ouabain. Na+, K+ ATPase activity was calculated by the difference between the two assays according to the method of Wyse et al. (2000). Specific activity of the enzyme was expressed as nmol Pi released per min per mg of protein.
Protein determination
Protein concentration was measured by the method of Bradford (1976), using bovine serum albumin as standard.
Statistical analysis
Data were expressed as mean ± SEM of the mean, and were analyzed by Student’s t-test for biochemical analysis and one-way ANOVA followed by Dunnett’s test for behavioral experiments. The significance level was accepted as different when the p value was equal or less than 0.05.
Results
Behavioral experiments
At doses of 0.125 and 0.5 mg/kg, veratrine reduced the immobility time [One way ANOVA, F(3,28) = 4.890, p < 0.01] and the spontaneous locomotion [One way ANOVA, F(3,28) = 5.255, p < 0.05] when compared to the respective control groups; at 0.06 mg/kg (i.p.) it did not have any effect. Thus, this dose was chosen to be co-administered with an effective dose of HC1 (360 mg/kg, p.o.): veratrine 0.06 mg/kg prevented the HC1 anti-immobility effect [One way ANOVA, F(3,30) = 3.252; p < 0.05] () without affecting the spontaneous locomotion ().
Figure 1. Effects of co-administration of HC1 (360 mg/kg, p.o.) and veratrine (0.06 mg/kg i.p.) on immobility time in the forced swimming test (A) and number of crossings in open-field test (B) in mice. Results expressed as mean ± SEM. *p < 0.05 compared to control groups (one-way ANOVA followed by Dunnett’s test).
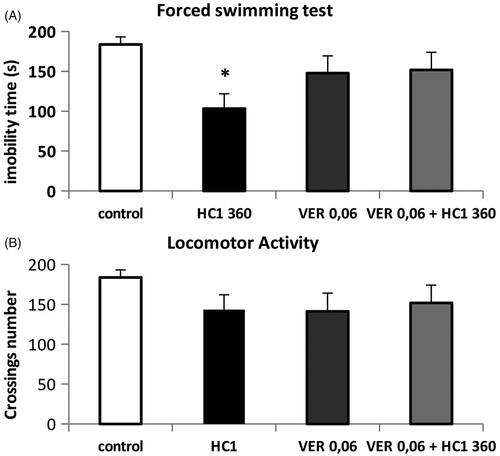
Biochemical experiments
Animals killed 2 h after the single administration of HC1 (360 mg/kg, p.o.) did neither present alterations in Na+, K+ ATPase activity in the cerebral cortex () [t (8) = 0.470, p = 0.651] nor in the hippocampus () [t (8) = 0.361, p = 0.728] when compared to the animals treated with vehicle. When animals were treated for 3 consecutive days with HC1 (360 mg/kg, p.o.) and killed 2 h after the third administration, they presented a significant increase in the enzyme activity, both in the cerebral cortex () [t (8) = 4.455, p < 0.005] and hippocampus () [t (10) = 5.607, p < 0.001].
Figure 2. Effects of HC1 (360 mg/kg, p.o.) acute treatment (a single administration) on Na+, K+ ATPase activity in the cerebral cortex (A) and in the hippocampus (B) of mice. Panels C and D shows the effects of HC1 (360 mg/kg, p.o.) repeated treatment on Na+, K+ ATPase activity in the cerebral cortex and in the hippocampus of mice. Results expressed as mean ± SEM (t-test). **p < 0.01 and ***p < 0.001 compared to control groups.
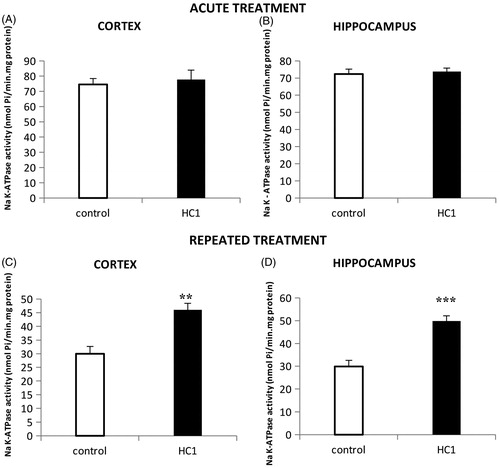
When animals were treated acutely (a single administration of HC1 360 mg/kg, p.o.) and exposed to the forced swimming session, there was no significant alteration in Na+, K+ ATPase activity neither in the cerebral cortex () [t (10) = 0.276, p = 0.611] nor in the hippocampus () [t (10) = 0.479, p = 0.633], when compared to the animals treated with vehicle and also submitted to the forced swimming session. In the same way, animals treated for 3 days with a daily dose of HC1 (360 mg/kg, p.o.), and exposed to the forced swimming session, did not present changes in Na+, K+ ATPase activity neither in the cerebral cortex () [t (10) = 1.160, p = 0.307] nor in the hippocampus () [t (10) = 0,001, p = 0.982], when compared to control animals.
Figure 3. Effects of HC1 (360 mg/kg, p.o.) acute treatment (a single administration) on Na+, K+ ATPase activity in the cerebral cortex (A) and in the hippocampus (B) of mice submitted to swimming session. Panels C and D shows the effects of HC1 (360 mg/kg, p.o.) repeated treatment on Na+, K+ ATPase activity in the cerebral cortex and in the hippocampus of mice submitted to swimming session. Results expressed as mean ± SEM (t-test).
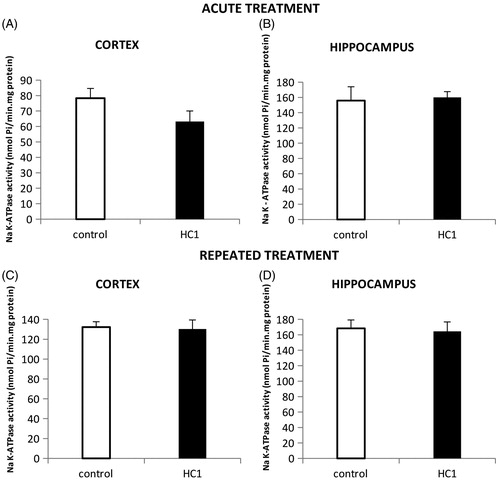
Discussion
The present results replicated previous findings of our laboratory that showed that HC1 reduces immobility behavior in the FST (Viana et al., Citation2005). The effect of the Na+ channel opener veratrine was also in line with earlier results (Prica et al., Citation2008) demonstrating that it is devoid of effect on the FST when administered alone at low doses (0.06 mg/kg, i.p.). At this dose, veratrine prevented the anti-immobility effect of HC1, suggesting that the antidepressant-like effect of HC1 could be related to Na+ influx inhibiting properties.
Pancrazio et al. (Citation1998) demonstrated the ability of tricyclic antidepressants to inhibit the neuronal Na+ channels. Na+ channels are also molecular targets for antiepileptic drugs, such as lamotrigine, topiramate, phenytoin, carbamazepine and valproic acid, which are also mood stabilizers (El-Mallakh et al., Citation2010; Lenkey et al., Citation2010).
The entry of Na+ from the extracellular space into the cell takes place through multiple routes consisting of voltage-sensitive and ligand-gated ion channels as well as various transporters. Central to this Na+-regulating capacity is the Na+, K+ ATPase, which can rapidly activate or deactivate in response to changes in intracellular concentration of Na+ (Inoue & Matsui, Citation1990). Small changes in the cytoplasmic Na+ concentrations secondary to activation of either various Na+-dependent transporters or Na+ channels can have dramatic effects on Na+, K+ ATPase activity.
Since the hippocampus is a region particularly sensitive to stress effects (McEwen, Citation2001) and different morphological and neurochemical effects have been reported in hippocampus after depressive states (Sheline et al., Citation2003), we decided to evaluate the effect of HC1 on the activity of Na+, K+ ATPase on this brain region. We also investigated the effect of HC1 on cerebral cortex Na+, K+ ATPase activity, once our group has already reported that a H. caprifoliatum extract reduced cortical corticosterone levels in stressed mice (Viana et al., Citation2008).
In the present study, repeated HC1 administration (360 mg/kg, p.o. during 3 days) caused a significant increase in Na+, K+ ATPase activity in cerebral cortex and hippocampus of mice when compared to vehicle-treated animals. However, it is worth noting that the reduction in FST immobility time after the acute administration of HC1 appears not to be directly linked to an increase in Na+, K+ATPase activity, since when administered acutely HC1 had no effect in enzyme activity. The regulation of Na+, K+ ATPase activity is a complex matter and is subjected to both short- and long-term regulation by a variety of hormones. The long-term regulatory mechanisms generally affect de novo Na+, K+ ATPase synthesis or degradation (Therien & Blostein, Citation2000). It is conceivable that HC1 affects the long-term regulatory mechanisms since the Na+, K+ ATPase activity was affected by repeated treatment only. Our data so far does not permit us to establish how far the ability of HC1 to inhibit Na+ influx is linked with its capacity for increasing Na+, K+ ATPase long-term activity. But undoubtedly the Na+ gradient plays a role in the antidepressant-like effect of HC1 in the FST.
This finding might contribute for understanding the action of HC1 on monoamine neuronal carriers previously reported by Viana et al. (Citation2005). Na+-gradient is the main driving force of the monoamine transporters (Xhaard et al., Citation2008). As Na+ is transported along the electrochemical gradient, this provides the energy required for the thermodynamically unfavorable transport of substrates (Kristensen et al., Citation2011). The plasma membrane transporters are indirectly driven by the Na+, K+ ATPase which generates gradients of Na+ and K+ and in the process creates a membrane potential (Rudnick, Citation1998).
Interestingly, neither acute nor repeated HC1 treatments were able to alter the Na+, K+ ATPase activity in mice subjected to FST. Animal models of depression have been associated with decreased Na+, K+ ATPase activity (de Vasconcellos et al., Citation2005; Gamaro et al., Citation2003) and it has already been demonstrated possible involvement of endogenous ouabain-like compound in behavioral stress. Weidemann et al. (Citation2004) reported that acute stress can induce the production of digitalis-like compounds in the adrenal gland and the hypothalamus of mice, these being able to inhibit Na+, K+ ATPase. In addition, Goto et al. (Citation1995) demonstrated in rats that acute swimming stress resulted in significant increases of ouabain-like compound levels in plasma and adrenal. Furthermore, steroid hormones, in particular, corticosteroids, have specific long- and short-term regulatory effects on the Na+, K+ ATPase (Therien & Blostein, Citation2000) and it has already been proved that a short forced swimming session increases both plasma and cortical corticosterone levels in mice (Viana et al., Citation2008). The antidepressants imipramine and bupropione as well as a lipophilic extract of H. caprifoliautm were able to reduce the cortical increase of corticosterone induced by forced swimming (Viana et al., Citation2008). Thus, it is conceivable that HC1 acts by counteracting the decrease in Na+, K+ ATPase activity induced by forced swimming stress. This hypothesis deserves further studies.
Conclusions
The antidepressant-like effect of HC1, a fraction enriched in phloroglucinol derivatives obtained from H. caprifoliatum, in the mice FST appears to be dependent of intracellular Na+ concentrations, since it was prevented by veratrine, an opener of voltage sensitive Na+ channels. Besides, HC1 possibly activates Na+, K+ ATPase through long-term regulatory mechanisms. Our results confirmed the potential antidepressant effect of H. caprifoliatum and stressed the notion that phloroglucinol derivatives represent a new molecular pattern with antidepressant properties.
Declaration of interest
The authors report no declarations of interest.
This work was supported by CAPES, CNPq and Programa de Pós-Graduação em Ciências Farmacêuticas (PPGCF-UFRGS).
Acknowledgements
The authors are thankful to A. C. Stein and D. Hasse for technical assistance during the experiments.
References
- Bradford MM. (1976). A rapid and sensitive method for the quantitation of microgram quantities of protein utilizing the principles of protein--dye binding. Anal Biochem 72:248--54
- Brasil. (2008). Ministério Público. Lei no. 11.794, de 8 de outubro de 2008. Published in DOU 9.10.2008
- Council for International Organizations of Medical Sciences. International Guiding Principles for Biomedical Research Involving Animals. Geneva: CIOMS; 1985
- Crema L, Schlabitz M, Tagliari B, et al. (2010). Na+, K+ ATPase activity is reduced in amygdala of rats with chronic stress-induced anxiety-like behavior. Neurochem Res 35:1787–95
- Dall'Agnol R, Ferraz A, Bernardi AP, et al. (2003). Antimicrobial activity of some Hypericum species. Phytomedicine 10:511–16
- Daudt R, von Poser GL, Neves G, Rates SM. (2000). Screening for the antidepressant activity of some species of Hypericum from South Brazil. Phytother Res 14:344–6
- de Vasconcellos AP, Zugno AI, dos Santos AH, et al. (2005). Na+, K+-ATPase activity is reduced in hippocampus of rats submitted to an experimental model of depression: Effect of chronic lithium treatment and possible involvement in learning deficits. Neurobiol Learn Mem 84:102–10
- El-Mallakh RS, Elmaadawi AZ, Loganathan M, et al. (2010). Bipolar disorder: An update. Postgrad Med 122:24–31
- Ferraz A, Bordignon S, Mans D, et al. (2002). Screening for the presence of hypericins in southern Brazilian species of Hypericum (Guttiferae). Pharm Biol 40:294–7
- Gamaro GD, Streck EL, Matté C, et al. (2003). Reduction of hippocampal Na+, K+-ATPase activity in rats subjected to an experimental model of depression. Neurochem Res 28:1339–44
- Goto A, Yamada K, Nagoshi H, et al. (1995). Stress-induced elevation of ouabain-like compound in rat plasma and adrenal. Hypertension 26:1173–6
- Grisar T. (1984). Glial and neuronal Na+–K+ pump in epilepsy. Ann Neurol 16:S128–34
- Herman L, Hougland T, El-Mallakh RS. (2007). Mimicking human bipolar ion dysregulation models mania in rats. Neurosci Biobehav Rev 31:874–81
- Inoue N, Matsui H. (1990). Activation of a brain type Na pump after glutamate excitation of cerebral neurons. Brain Res 534:309–12
- Kristensen AS, Andersen J, Jøgersen TN, et al. (2011). SLC6 neurotransmitter transporters: Structure, function, and regulation. Pharmacol Rev 63:585–640
- Lenkey N, Karoly R, Lukacs P, et al. (2010). Classification of drugs based on properties of sodium channel inhibition: A comparative automated patch-clamp study. PLoS One 5:e15568 . doi: 10.1371/journal.pone.0015568
- Li R, El-Mallakh RS. (2004). Differential response of bipolar and normal control lymphoblastoid cell sodium pump to ethacrynic acid. J Affect Disord 80:11–17
- Linde K, Schumann I, Meissner K, et al. (2011). Treatment of depressive disorders in primary care-protocol of a multiple treatment systematic review of randomized controlled trials. BMC Fam Pract 12:127–33
- Looney SW, El-Mallakh RS. (1997). Meta-analysis of erythrocyte Na, K-ATPase activity in bipolar illness. Depress Anxiety 5:53–65
- McEwen BS. (2001). Plasticity of the hippocampus: Adaptation to chronic stress and allostatic load. Ann N Y Acad Sci 933:265–77
- Müller LG, Salles LA, Stein AC, et al. (2012). Antidepressant-like effect of Valeriana glechomifolia Meyer (Valerianaceae) in mice. Prog Neuropsychopharmacol Biol Psychiatry 36:101–9
- Nör C, Albring D, Ferraz ABF, et al. (2004). Phloroglucinol derivatives from our Hypericum species belonging to the Trigynobrathys section. Biochem Syst Ecol 32:517–19
- Pancrazio JJ, Kamatchi GL, Roscoe AK, Lynch C 3rd. (1998). Inhibition of neuronal Na+ channels by antidepressant drugs. J Pharmacol Exp Ther 284:208–14
- Porsolt RD, Anton G, Blavet N, Jalfre M. (1978). Behavioural despair in rats: A new model sensitive to antidepressant treatments. Eur J Pharmacol 47:379–91
- Prica C, Hascoet M, Bourin M. (2008). Antidepressant-like effect of lamotrigine is reversed by veratrine: A possible role of sodium channels in bipolar depression. Behav Brain Res 191:49–54
- Rocha L, Marston A, Potterat O, et al. (1995). Antibacterial phloroglucinols and flavonoids of Hypericum brasilience. Phytochemistry 40:1447–52
- Rudnick G. (1998). Bioenergetics of neurotransmitter transport. J Bioenerg Biomembr 30:173–85
- Sheline YI, Gado MH, Kraemer HC. (2003). Untreated depression and hippocampal volume loss. Am J Psychiatry 160:1516–18
- Therien AG, Blostein R. (2000). Mechanisms of sodium pump regulation. Am J Physiol Cell Physiol 279:541–66
- Viana A, Rates S, Naudin B, et al. (2008). Effects of acute or 3-day treatments of Hypericum caprifoliatum Cham. & Schltdt. (Guttiferae) extract or of two established antidepressants on basal and stress-induced increase in serum and brain corticosterone levels. J Psychopharmacol 22:681–90
- Viana AF, do Rego JC, von Poser G, et al. (2005). The antidepressant-like effect of Hypericum caprifoliatum Cham & Schlecht (Guttiferae) on forced swimming test results from an inhibition of neuronal monoamine uptake. Neuropharmacology 49:1042–52
- Weidemann H, Salomon N, Avnit-Sagi T, et al. (2004). Diverse effects of stress and additional adrenocorticotropic hormone on digitalis-like compounds in normal and nude mice. J Neuroendocrinol 16:458–63
- Wonnemann M, Singer A, Müller WE. (2000). Inhibition of synaptosomal uptake of 3H-l-glutamate and 3H-GABA by hyperforin, a major constituent of St. John's Wort: The role of amiloride sensitive sodium conductive pathways. Neuropsychopharmacology 23:188–97
- Wyse AT, Streck EL, Barros SV, et al. (2000). Methylmalonate administration decreases Na+, K+-ATPase activity in cerebral cortex of rats. Neuroreport 11:2331--4
- Xhaard H, Backström V, Denessiouk K, Johnson MS. (2008). Coordination of Na(+) by monoamine ligands in dopamine, norepinephrine, and serotonin transporters. J Chem Inf Model 48:1423–37