Abstract
Context: Medicinal plants are continuously screened for their pharmacological properties. Despite the diversity and the numerous phytochemicals found in Ardisia (Myrsinaceae) species, its full biological potential has not been fully explored.
Objective: Four naturally occurring alkylbenzoquinone derivatives, namely ardisiaquinone N (1), ardisiaquinone J (2), ardisiaquinone K (3) and a mixture of ardisiaquinone P (4) and K (3) from Ardisia kivuensis Taton (Myrsinaceae) were investigated in vitro for their cytotoxicity and antimicrobial activity.
Materials and methods: Minimal inhibitory concentration (MIC) was determined using the broth micro-dilution assay. Tumor cells growth inhibition was performed by sulphorhodamine B (SRB) assay while sub-diploid DNA fraction was measured by flow cytometry.
Results: Compounds 1, 2 and 3 showed significant antimicrobial activity against two Gram-positive bacteria and one fungus (with MICs varying between 3.12 and 6.25 µg/ml). The four compounds exhibited remarkable antiproliferative activity against the leukemia cell line
TPH-1 with IC50 inhibition values between 2 and 2.1 µg/ml. Cytotoxic activity was found to be related to apoptosis induction.Discussion and conclusion: These findings suggest that natural compounds herein studied are interesting potential candidates for the development of new therapeutic agents, especially against leukemia and Gram-positive bacterial infections.
Introduction
The global emergence of multidrug resistant microorganisms is increasingly limiting the effectiveness of current drugs and significantly causing treatment failure of infections (Bowler, Citation2012). A substantial number of microbial pathogens have been putatively linked to cancer (Helicobacter pylori, Hepatitis C virus), and in turn, up to 30% of all cancers worldwide have been related to infectious diseases (Buonaguro et al., Citation2006). In cancer, besides the impairment of the immunological status associated with the main diseases, several chemotherapeutic agents can often cause additional immunosuppression creating conditions for the emergence of opportunistic infections (Fávaro, Citation2012). This situation emphasizes the urgent need for developing new drugs. In this context, natural compounds that can possess both cytotoxic and antimicrobial properties are considered potential candidates for the treatment of these disease conditions.
Plants have traditionally provided a source of hope for novel drug compounds, as plant herbal mixtures have made large contributions to human health and well-being (Harvey, Citation2008). Medicinal plants are continuously screened for their phytochemical and pharmacological properties. Ardisia is a large genus of the family Myrsinaceae, comprising 500 species distributed throughout the subtropical and tropical regions of the world (Kobayashi & Mejia, Citation2005). Several Ardisia species have been used for medicinal purposes. Due to their medicinal importance, extensive phytochemical studies have been carried out on a large number of Ardisia species and a variety of compounds have been isolated including saponins, coumarins and quinones. Although some of these compounds may also be found in other plant species, the unique combinations of phytochemical constituents in Ardisia species make this plant material a novel source of potential phytotherapeutic agents (Kobayashi & Mejia, Citation2005). Previously, ardisiquinones A–C were firstly isolated in 1968 from the root bark of Ardisia sieboldii Miq., then 27 years after (1995), the isolation of ardisiquinone D–F were reported from the same species (Ignatious et al., Citation2011). In 2001, the series was extended to isolation of ardisiaquinones G–I from the leaves of A. teysmanniana (Myrsinaceae) (Ignatious et al., Citation2011). Recent phytochemical screening of leaves and stem bark of A. kivuensis led to the identification of alkylbenzoquinone derivatives, namely ardisiquinone J–P (Ndontsa et al., Citation2011, Citation2012).
Despite the diversity of the genus and the numerous phytochemicals found in Ardisia species, its full potential has not been fully explored; further investigation on pharmacological screening may open new opportunities to discover chemopreventive agents and to develop pharmaceuticals based on Ardisia constituents. To this end, we undertook to investigate antimicrobial properties of ardisiquinone against a wide range of microbial pathogens as well as their cytotoxic properties against various human cancer cell lines.
Materials and methods
Natural product compounds
The natural compounds used in this study were obtained from the chemical bank of the Laboratory of Organic Chemistry, University of Dschang, Cameroon. They were isolated from the stem bark and leaves of A. kivuensis Taton (syn. Afrardisia cymosa). The isolation procedure and the structure elucidation of compounds (Figure 1) were performed as previously described (Ndontsa et al., Citation2011, Citation2012).
Antimicrobial assay
Bacterial growth conditions
A total of nine microbial strains were tested for their susceptibility to compounds. These strains comprised three Gram-negative Pseudomonas aeruginosa ATCC 27853, Escherichia coli ATCC 25292, vancomycin-resistant Enterococcus faecium (VRE) ATCC 51299 and four Gram-positive bacteria: Methicillin susceptible Staphylococcus aureus (MSSA1), Staphylococcus aureus ATCC 29213, Methicillin-resistant Staphylococcus aureus (MRSA) ATCC 33591 and Staphylococcus epidermidis ATCC 12228 and three fungi Candida albicans (ATCC 10231), Cryptococcus neoformans H99, Cryptococcus neoformans KN99a. They were sub-cultured on fresh appropriate agar plates 24 h prior to any antimicrobial test. The Mueller Hinton Agar (MHA) or Yeast Polypeptone Dextrose Agar (YPDA) was used for the bacteria and fungi activation, respectively, while Mueller Hinton Broth (MHB) or RPMI-1640 was used for the MIC determination.
Minimal inhibitory concentration determination
The minimal inhibitory concentration (MIC) was performed by the broth microdilution method. Stock solutions of compounds were prepared in 100% dimethylsulfoxide (DMSO, Sigma, St. Louis, MO, Cat No. D-2650) and two-fold serial dilutions were prepared in media in amounts of 100 μl per well in 96-well. Microbial suspensions were prepared in sterile normal saline (0.85%) from 24 h (bacteria) or 48 h (yeast) culture. The turbidity of the microbial suspension was adjusted with a densitometer to a McFarland standard of 0.5 for bacteria and 0.9 for yeast, which are equivalent to 1--5 × 108 CFU/ml and 1--5 × 106 CFU/ml, respectively. These suspensions were further diluted to 1:100 in media, and a 100 μl volume of this diluted inoculum was added to each well of the plate, resulting in a final inoculum of approximately 1--5 × 106 CFU/ml or 1--5 × 104 CFU/ml. The final concentration of samples ranged from 0.78 to 100 μg/ml. The final concentration of DMSO was lower than 2.5% and does not affect the microbial growth (Kuete et al., Citation2008). The medium without the agents was used as a growth control and the blank control used contained only the medium. Ciprofloxacin and amphothericin B served as the reference drug for positive controls. The microtiter plates were incubated at 37 °C for 24 h for bacteria and 48 h for yeast. The assay was repeated three-times. The plates were read visually, and the MIC was defined as the lowest concentration of the compound that prevented visible growth with respect to the growth control.
Anticancer activity
Cell lines and treatment
The effect of the extracts and compounds on cell growth was determined in a panel of five human tumor cells including lung A549 adenocarcinoma, breast carcinoma MCF-7, prostate carcinoma PC-3, cervical carcinoma HeLa and acute monocytic leukemia cell line THP-1,
obtained from National Cancer Institute, USA. THP-1, A-549 and PC-3 were maintained in RPMI-1640 medium while MCF-7 and HeLa were cultured in MEM medium. All media used were supplemented with 10% fetal bovine serum (FBS), 100 IU/ml penicillin. The cell lines were maintained under standard cell culture conditions at 37 °C and 5% CO2 in a humidified environment.Cytotoxic activity by SRB assay
In vitro cytotoxicity against the above-mentioned five human cell lines was determined using the sulphorhodamine B assay (SRB) as described previously (Skehan et al., Citation1990). Briefly, cells were harvested in log phase using trypsin (0.05% trypsin, 0.02% EDTA, in PBS). The cell suspensions were diluted with appropriate growth medium to obtain cell densities depending on the cell line: (104 cells/well for HeLa, 104 cells/well for A549, 104 cells/well for THP-1, 1.5 × 104 cells/well for MCF-7 and 104 cells/well for PC-3). An aliquot of 100 µl of each suspension were seeded in 96-wells cell culture plates. The cells were incubated at 37 °C in an atmosphere of 5% CO2 and 95% relative humidity in a CO2 incubator. After 24 h incubation, compounds (100 µl/well) at varying concentrations (1, 10, 30 and 50 µg/ml) were added to the wells containing cells. Paclitaxel 0.1, 1 and 10 µM was used as positive reference. Suitable controls with equivalent concentration of DMSO were also included. The plates were further incubated for 48 h in a CO2 incubator after addition of test material. After incubation cells were fixed by gently layering trichloroacetic acid (50 µl/well, 50% w/v) on top of the medium in all the wells and incubated at 4 °C for 1 h. The plates were washed five times with distilled water and air-dried. Cell growth was measured by staining with sulforhodamine B dye (0.4% w/v in 1% acetic acid, 100 µl/well). The unbound dye was washed 3–5 times with 1% acetic acid and plates were air-dried. The adsorbed dye was dissolved in Tris-Buffer (100 µl/well, 0.01 M, pH 10.4) and plates were gently shaken for 10 min on a mechanical shaker. The optical density (OD) was recorded using a 96-well plate reader. Growth inhibition was calculated by subtracting mean OD values of respective blank from the mean OD value of an experimental set. Percentage growth in presence of compound was calculated considering the growth in the absence of any test material as 100% and in turn percentage growth inhibition in the presence of test material was calculated. The viability and growth in the presence of compound was calculated by the following formula:
The IC50 value is the concentration of sample required to inhibit 50% of the cell proliferation and was calculated by plotting the percentage survival versus the concentrations, using Microsoft office Excel 2010. For all samples, each compound concentration was tested in triplicates in a single experiment.
Sub-G1 DNA content analysis
Sub-G1 analysis was performed as described earlier (Park et al., Citation2007). Briefly, THP-1 cells (106/ml) in exponential phase of growth were seeded in 24-well tissue culture plates and allowed to adhere for 24 h. On day 2, the old medium was changed and the cells were treated with compounds at different concentrations and further incubated for 24 h. Cells were trypsinised, centrifuged and washed with PBS, then fixed gently by adding 70% ethanol. They were fixed in 70% ethanol, washed with PBS and then successively treated with RNase and with propidium iodide (PI) [25 µg/ml] and incubated at 37 °C for 30 min. The percentages of DNA content in sub-G1 cells population were measured using BD-LSR flow cytometer equipped with electronic doublet discrimination capability using blue (488 nm) excitation from argon laser. Data were collected in list mode on 10 000 events for FL2-A versus FL2-W.
Results and discussion
Antimicrobial activity
The minimum inhibitory concentration values of compounds 1, 2, 3 and 4 + 3 against 10 microbial strains are shown in . Each compound was active against at least two bacteria and one fungal strain. All the compounds showed selective antimicrobial activity with MIC values varying between 3.12 and 100 µg/ml. Compounds 1, 2 and 3 were the most active, inhibiting both Gram-negative bacteria and fungi with the least MIC values of 3.12 and 6.25 µg/ml, respectively. All the compounds exhibited weak activity against Gram-negative bacteria that were the most resistant strains with MIC ≥ 25 µg/ml. Natural product compounds are routinely classified as antimicrobials on the basis of the susceptibility test that produce MIC in the range of 10 to 100 µg/ml (Simoes et al., Citation2009). Activity is considered to be significant if MIC values are below 10 µg/ml, moderate when 10 < MIC <100 µg/ml and weak if MIC > 100 µg/ml (Kuete, Citation2010). Therefore, the activity recorded in this work varied selectively from significant to weak for all the four alkylbenzoquinone derivatives compounds studied. However, in this study, MIC > 100 µg/ml was considered as not active, as reported in . Many naturally occurring quinones such as naphtoquinones have been found to exhibit antimicrobial activity. Kim et al. (Citation2010) reported the antimicrobial activity of 1,4-benzoquinones from wheat germ extract. Nevertheless, very little study has been reported on antimicrobial potential of alkylbenzoquinone derivatives. It is important to notice that S. aureus and S. epidermitis were the most susceptible bacteria (inhibited by all the four compounds tested). The activity of compounds 1, 2 and 3, which were the most efficient, was more pronounced on Gram-positive S. aureus and S. epidermitis with MICs values of 3.12 µg/ml on MSSA1 bacteria strains. This observation is consistent with previous published literature according to which, due to their outer membrane that contributes to the intrinsic resistance by acting as an efficient permeability barrier, Gram-negative bacteria are in general more resistant to a large number of antibiotics and chemotherapeutic agent than are Gram-positive (Nikaido, Citation1998). Overall, antimicrobial results showed that alkylbenzoquinone derivatives possess interesting growth inhibition effect against Gram-positive bacteria and C. neoformans H99. Hence, these compounds could be considered as promising antimicrobial agents. Even failing to be used directly as antimicrobial, these compounds might be potential lead compounds that could serve as templates for further medicinal chemistry modifications to develop more efficient antimicrobial therapeutic agents.
Table 1. MIC results for antimicrobial activity of compounds (µg/ml).
Cytotoxic activity
Worldwide, 15 to 20% of cancers are linked to infectious diseases (Liao, Citation2007). In developed western countries, approximately 10% of all cancers are linked to infectious agents, but they account for as much as 20% of all cancers in developing countries (Buonaguro et al., Citation2006). Such evidence has contributed to the major change in cancer drug discovery. Within this new pathogen-related cancers approach, discovery and development of new natural therapeutic agents that can both inhibit human microbial pathogens and tumor cells growth is a new challenge. Therefore, natural alkylbenzoquinone derivative compounds in this study were also tested for their growth inhibiting effect against five human cancer cell lines. The five human cell lines used were representative of tumors from a five variety of human tissue types including blood, lung, breast, prostate and cervix tissues. Primary screening of compounds was performed at a single concentration of 50 μg/ml and results as percentage of growth inhibition () showed that, compounds 2 and 3 had no inhibitory effect on PC-3; a similar observation was found against Hela. However, each of the four compounds had more than 50% inhibition on at least one of the five cell lines. Therefore, their IC50 was determined and results are shown in . It is clear from this table that all the compounds showed strong cytotoxic effect on THP-1 cells (IC50 2–2.1 µg/ml). This activity was two-fold higher compared to paclitaxel used in this study as reference anticancer drug. According to the in vitro cytotoxic activity criteria made by Syarifah et al. (Citation2011), a compound is considered as weakly active if the IC50 ≥ 50 µg/ml, moderately active for 10 µg/ml < IC50 < 50 µg/ml and significantly active if IC50 ≤ 10 µg/ml. Brahemi defined a cutoff point at 4 µg/ml (Brahemi et al., Citation2010). Considering these criteria, the activity obtained with our compounds against THP-1 could be considered as significant. The occurrence of alkylbenzoquinone in Ardisia species as well as their cytotoxic activity against murine breast cancer cell line was previously reported (Sumino et al., Citation2001). Apart THP-1 cell, the antiproliferative activity of compounds against the other cell lines was ranged from moderated to weak. It is noticeable that, regarding the interesting results obtained with THP-1, no structure activity relationship was observed. This was also difficult to establish from the results obtained with other cell lines, although compounds 1 and 2 were not active against PC-3 and compounds 3 + 4 against Hela, their activity towards THP-1 was similar to other compounds.
Figure 2. Antiproliferative activity of compounds 1, 2, 3 and 3 + 4 against HELA, A-549, PC-3, THP-1 and MCF-7 cancer cell lines determined by percentage of growth inhibition.
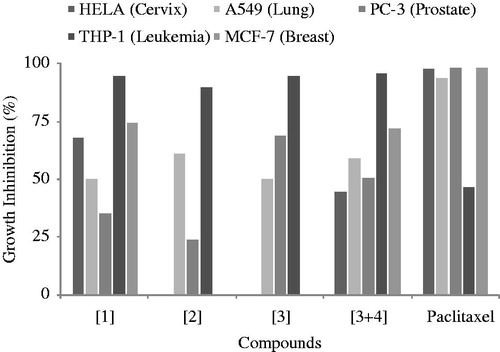
Table 2. IC50 values of compounds on human cancer cell lines (µg/ml).
Because of the remarkable cytotoxic activity of our compounds on THP-1, we investigate whether their inhibitory effect was related to apoptosis induction or not. Thus, sub-diploid DNA content of THP-1 cells was measured by flow cytometry 24 h after treatment with compounds, results are shown in DNA fluorescence histogram (). Treatment of THP-1 with compounds induced the appearance of sub-G1 peak in DNA in the high range of 94.8–98%. This is the percentage of the cell population in which compounds have induced death by apoptosis. Because of this high percentage, DNA content of cells in G1, S and G2/M phases could not appear in the histogram. Paclitaxel showed only 30% of sub-diploid DNA peak. In fact, it has been recognized that apoptotic cells have a reduced DNA stainability following the staining with fluorochromes such as PI, therefore the appearance of cells with low DNA stainability, lower than that of G1 cells (sub-G1) in cultures treated with drugs has been considered to be the hallmark of cell death by apoptosis (Darzynkiewicz et al., Citation1992; Nicoletti et al., Citation1991). Our findings were similar to the recent observation made from ardisianone, a natural benzoquinone derivative, which was shown to display anti-proliferative and apoptotic activities against human hormone-refractory prostate cancer cells (HRPC), PC-3 and DU-145 (Yu et al., 2013). However, hypodiploid DNA peak sometimes may consist of apoptotic cells, necrotic cells, debris blebs of cytotoxic drugs and other debris, resulting either in a strong overestimation of apoptotic cell percentage because isolated apoptotic bodies are counted as apoptotic cells or in an underestimation of the phenomenon if apoptotic cells are gated out as debris (Darzynkiewicz et al., Citation1992). Although necrosis induced by metabolic poisons or lysis produced by complement did not induce any sub-G1 peak in the DNA fluorescence histogram, further investigations such as DNA fragmentation and cell distribution in cell cycle analysis are needed to confirm the apoptotic effect of our compounds. It comes out from this study that alkylbenzone derivatives isolated from A. kivuensis exhibited remarkable antiproliferative activity against the leukemia cell line
TPH-1 and their cytotoxic effect found to be likely related to apoptosis induction.Conclusion
These findings suggest that natural alkylbenzone derivatives from plants are interesting potential candidates for the development of new therapeutic agents especially against leukemia and infectious diseases cause by Gram-positive bacteria.
Declaration of interest
The authors report no conflicts of interest.
Acknowledgements
JPD is thankful to Jawaharlal Nehru Centre for Advanced Scientific Research (JNCASR), the Centre for International Co-operation in Science (CICS).
References
- Bowler PG, Welsby S, Towers V, et al. (2012). Multidrug-resistant organisms, wounds and topical antimicrobial protection. Int Wound J 9:387–96
- Brahemi G, Kona FR, Fiasella A, et al. (2010). Exploring the structural requirements for inhibition of the ubiquitin E3 ligase breast cancer associated protein 2 (BCA2) as a treatment for breast cancer. J Med Chem 53:2757–65
- Buonaguro FM, George KL, PierGiuseppe P. (2006). Introducing infectious agents and cancer. Infect Agent Cancer 1:1
- Darzynkiewicz Z, Bruno S, Del Bino G, et al. (1992). Features of apoptotic cells measured by flow cytometry. Cytometry 13:795–808
- Fávaro WJ, Nunes OS, Seiva FR, et al. (2012). Effects of P-MAPA immunomodulator on toll-like receptors and p53: Potential therapeutic strategies for infectious diseases and cancer. Infect Agent Cancer 7:14
- Harvey AL. (2008). Natural products in drug discovery. Drug Discov Today 13:894–901
- Ignatious A, Rahul J, Pushpa P, Pardasani RT. (2011). Recent advances in 1,4-benzoquinone chemistry. J Braz Chem Soc 22:385–421
- Kim MH, Jo SH, Ha KS, et al. (2010). Antimicrobial activities of 1,4-benzoquinones and wheat germ extract. J Microbiol Biotechnol 20:1204–9
- Kobayashi H, Mejia E. (2005). The genus Ardisia: A novel source of health-promoting compounds and phytopharmaceuticals. J Ethnopharmacol 96:347–54
- Kuete V. (2010). Potential of Cameroonian plants and derived-products against microbial infections: A review. Planta Med 76:1479–91
- Kuete V, Ngameni B, Fotso Simo CC, et al. (2008). Antimicrobial activity of the crude extracts and compounds from Ficus chlamydocarpa and Ficus cordata. (Moraceae). J Ethnopharmacol 120:17–24
- Liao BJ. (2007). Viruses and human cancer. Yale J Biol Med 79:115–22
- Ndontsa BL, Tala MF, Talontsi FM, et al. (2012). New cytotoxic alkylbenzoquinone derivatives from leaves and stem of Ardisia kivuensis (Myrsinaceae). Phytochem Lett 5:463–6
- Ndontsa BL, Tatsimo JSN, Csupor D, et al. (2011). Alkylbenzoquinones with antiproliferative effect against human cancer cell lines from stem of Ardisia kivuensis. Phytochem Lett 4:227–30
- Nicoletti I, Migliorati G, Pagliacci MC, et al. (1991). A rapid and simple method for measuring thymocyte apoptosis by propidium iodide staining and flow cytometry. J Immunol Meth 139:271–9
- Nikaido H. (1998). Antibiotic resistance caused by Gram-negative multidrug efflux pumps. Clin Infect Dis 27:S32–41
- Park WH, Han YW, Kim SW, et al. (2007). Antimycin A induces apoptosis in As4.1 juxtaglomerular cells. Cancer Lett 251:68–77
- Simoes M, Bennett RN, Rosa EA. (2009). Understanding antimicrobial activities of phytochemicals against multidrug resistant bacteria and biofilms. Nat Prod Rep 26:746–57
- Skehan P, Storeng R, Scudiero D. (1990). New colorimetric cytotoxicity assay for anticancer-drug screening. J Natl Cancer Inst 82:1107–12
- Sumino M, Sekine T, Ruangrungsi N, Ikegami F. (2001). Ardisiphenols A-C, novel antioxidants from the fruits of Ardisia colorata. Chem Pharm Bull 49:1644–6
- Syarifah SMM, Nurhanan MY, Haffiz JM, et al. (2011). Potential anticancer compound from Cerbera odollam. J Trop Forest Sci 23:89–96
- Yu CC, Wu PJ, Hsu JL, et al. (2013). Ardisianone, a natural benzoquinone, efficiently induces apoptosis in human hormone-refractory prostate cancers through mitochondrial damage stress and surviving downregulation. Prostate 73:133–45