Abstract
Context: Mogroside V, a compound isolated from Momordica grosvenori Swingle, which belongs to the Cucurbitaceae, is a traditional Chinese medicine reported to have anti-inflammatory potential in murine macrophages and a murine ear edema model.
Objective: To investigate the effects and mechanisms of action of this compound in a model of acute lung injury (ALI) induced by lipopolysaccharides (LPS).
Materials and methods: Female BALB/c mice were treated with commercial mogroside V (2.5, 5 and 10 mg/kg) for 1 h prior to intranasal injection of LPS (10 μg in 50 μl). After 12 h, airway inflammation in the ALI model was determined by the wet/dry weight (W/D) ratio, myeloperoxidase (MPO) activity of lung tissue, leukocyte recruitment and cytokine levels in the bronchoalveolar lavage fluid (BALF). Additionally, lung tissue was examined by histology and western blotting to investigate the changes in pathology and the signalling in the presence and absence of mogroside V.
Results: Mogroside V at 5 and 10 mg/kg inhibited airway inflammation induced by LPS as measured by the decrease in the histological changes (44 and 67.3% reduction in lung injury score, respectively), a 28.9 and 55.3% reduction in lung MPO activity, and inflammatory cell counts, interleukin-1β (IL‐1β, 382 and 280 pg/ml, respectively), IL-6 (378 and 232 pg/ml, respectively) and tumor necrosis factor-α (TNF-α, 12.5 and 7.8 ng/ml, respectively) levels in the BALF. Additionally, mogroside V treatment reduced the activation of cyclooxygenase 2 (COX-2), inducible NO synthase (iNOS), and the nuclear factor (NF)-κB.
Discussions and conclusions: Together, these data suggest that mogroside V has the potential to protect against LPS-induced airway inflammation in a model of ALI.
Introduction
The lung is a very complex immunologic organ, and pulmonary disorders can be classified according to the type of immune response elicited (Greenberger, Citation2008). Innate immunity is characterized by the presence of polymorphonuclear neutrophils (PMNs) and the upregulation of procoagulant activity and TNF-α levels, all of which are important mediators in diseases such as pneumonia and ALI (Opitz et al., Citation2010).
ALI, the basis of acute respiratory distress syndrome (ARDS), is a clinical syndrome characterized by an acute inflammatory process that includes neutrophil accumulation, diffuse lung inflammation (resulting in noncardiogenic pulmonary edema) and impaired gas exchange. ALI has also been thought to contribute to fibrosis (which can arise from a number of insults such as sepsis) and viral infections (Ishii, Citation2011; Johnson & Matthay, Citation2010). ALI/ARDS involves several types of pathogenesis, such as oxidant/antioxidant disorders, dysregulation of inflammatory/anti-inflammatory pathway, upregulation of chemokine production and the increased upregulation of adhesion molecules (Bosma et al., Citation2005). LPS, a main component of the outer membrane of Gram negative bacteria, has been reported to induce the inflammatory response by activating numerous inflammatory cells and resulting in acute lung injury (ALI).
NF-κB, a key transcription factor involved in regulating the expression of proinflammatory mediators, can be activated when pulmonary cells are exposed to LPS. In most cell types, NF-κB complexes are retained in the cytoplasm by the inhibitory protein IκB (Karin, Citation1999; Karin & Ben-Neriah, Citation2000). NF-κB activation typically involves the phosphorylation of IκB by the IκB kinase (IKK) complex, which results in the degradation of IκB, and subsequent release of NF-κB, allowing it to translocate freely to the nucleus. Upon exposure to LPS, activated NF-κB can release numerous cytokines, including TNF-α, IL-6 and IL-1β, which play a critical role in the generation of ALI (Agouridakis et al., Citation2002; Bhatia & Moochhala, Citation2004).
Mogroside V () is a compound extracted from Momordica grosvenori, a fruiting plant that is primarily grown in Guangxi province in southern China. The fruits of Momordica grosvenori have been traditionally used to make hot drinks for the treatment of sore throat and the removal of phlegm. Recent research has shown that mogroside V possesses beneficial anticancer, antidiabetic, antioxidant and anti-inflammatory activities (Qi et al., Citation2008). Inflammation, a multi-step process that is mediated by activated inflammatory and immune cells, is a hallmark of many human diseases, including pulmonary inflammatory diseases (e.g., chronic obstructive pulmonary disease and asthma), infectious diseases and cancer (Ingram, Citation1995). Using murine macrophages and a murine ear edema model, previous studies have suggested that mogroside V exhibits anti-inflammatory potential by downregulating the inflammatory genes iNOS, COX-2 and IL-6 and upregulating some inflammation protective genes such as PARP1, BCL2l1, TRP53 and MAPK9 (Di et al., Citation2011). Although a number of studies have addressed the therapeutic potential of mogroside V, its ability to protect against LPS-induced ALI remains poorly understood. The aim of the present study was to assess the protective effects of mogroside V in a LPS-induced mouse ALIacute lung injury model and elucidate the potential anti-inflammatory mechanisms.
Materials and methods
Reagents
TNF-α, IL-1β and IL-6 ELISA kits were purchased from Biolegend (San Diego, CA). Goat anti-COX-2 and NOS2 rabbit polyclonal antibodies were purchased from Santa Cruz (Santa Cruz, CA). Phospho-NF-κB/p65 rabbit monoclonal antibody (mAb), NF-κB p65 (C22B4) rabbit mAb, phospho-IκBα (Ser32/36) (5A5) mouse mAb, IκBα rabbit mAb and β-actin mouse mAb were purchased from Cell Signaling Technology Inc. (Beverly, MA). Mogroside V (purity ≥98%) was obtained from the National Institute for the Control of Pharmaceutical and Biological Products (Beijing, China). Peroxidase-conjugated Affinipure goat Anti-mouse IgG (H + L) and Peroxidase-conjugated Affinipure goat Anti-rabbit IgG (H + L) were purchased from Protein Tech Group (Chicago, IL).
Animals
Female BALB/c mice, weighing approximately 18–20 g, were purchased from the Center of Experimental Animals at the Baiqiuen Medical College of Jilin University (Jilin, China) and bred under specific pathogen-free conditions. The mice were housed in microisolator cages and received food and water ad libitum. The laboratory temperature was 24 ± 1 °C, and relative humidity was 40–80%. All animals were treated in accordance with the guidelines for the Animal Welfare and Research Ethics Committee at Jilin University.
Murine model of LPS-induced ALI
All mice were randomly divided into the following seven groups (N = 5 per group): control, mogroside V alone, LPS alone, LPS + mogroside V (2.5 mg/kg), LPS + mogroside V (5 mg/kg), LPS + mogroside V (10 mg/kg) and LPS + dexamethasone (DEX). ALI was induced with LPS, as previously described (Zhang et al., Citation2009). Briefly, mice were challenged with 10 μg of LPS in 50 μl PBS intranasally (i.n.) after inhalational anesthesia with diethyl ether. Control mice were given 50 μl PBS i.n. without LPS. Mogroside V at 2.5, 5 and 10 mg/kg were dissolved in PBS and fed by gavage to mice 1 h prior to LPS administration. All the mice survived after 12 h of LPS treatment.
Collection of bronchoalveolar lavage
Mice were anesthetized after 12 h LPS treatment. Bronchoalveolar lavage fluid (BALF) was performed twice by intratracheal instillation of 500 μl of PBS. The lavage fluid was centrifuged and the supernatants were used for cytokines and chemokines measurements. Cell pellets were resuspended in 0.5 ml of PBS and used for total and differential cell counts on a hemocytometer and cytospins by staining with the Wright–Giemsa staining method.
Cytokine assays
TNF-α, IL-1β and IL-6 levels in the supernatant of BALF were measured with ELISA kits according to the instructions recommended by the manufactures (BioLegend, Inc., San Diego, CA). The optical density (OD) of the microplate was read at 450 nm.
Lung wet/dry weight ratio
After euthanizing the mice, the lungs were removed, blotted dry, weighed to obtain the “wet” weight, and then placed in an oven at 80 °C for 48 h to acquire the “dry” weight. The ratio of the wet weight to the dry weight was calculated to assess tissue edema.
Myeloperoxidase activity in lung
Myeloperoxidase (MPO), an indicator of polymorphonuclear leukocyte accumulation, was determined using test kits purchased from Nanjing Jiancheng Bioengineering Institute (Nanjing, China); every procedure was according to the instructions recommended by the manufactures. Twelve hours after LPS treatment, one part of right lungs was excised. Frozen lung tissue (50 mg) was homogenized and suspended in extraction buffer to obtain 5% of homogenate. The sample including 0.45 ml of homogenate and 0.05 ml of reaction buffer was heated to 37 °C in a water bath for 15 min, after which, the enzymatic activity was determined by measuring the changes in absorbance at 460 nm using a 96-well plate reader.
Lung histology
Histopathological evaluations were performed on the mice. Lungs were removed by dissection and fixed in 4% paraformaldehyde. Lung tissue was sectioned, embedded in paraffin, and cut into 3 μm sections. Tissue sections were then stained with hematoxylin and eosin stain (H&E) to observe morphology (McKay et al., Citation2004).
Western blot analysis of iNOS, COX-2 and NF-κB
Lung tissue was added to lysis buffer and homogenized. Nuclear and cytoplasmic fractions of lung were prepared as previously described (Ito et al., Citation2001). Whole cell lysates were prepared in 1% non diet P-40 lysis buffer with freshly added protease and phosphatase inhibitors. Protein was separated by SDS/PAGE and transferred onto PVDF membrane. Membranes were probed with the following antibodies: anti-COX-2, NOS2 rabbit polyclonal antibody, phospho-NF-κB/p65 rabbit mAb, NF-κB p65 (C22B4) rabbit mAb, phospho-IκBα (Ser32/36) (5A5) mouse mAb, IκBα rabbit mAb and β-actin mouse mAb. The membranes were further probed with HRP-conjugated secondary antibodies and detected by ECL western blot substrate.
Statistical analysis
The data were obtained from three independent experiments and expressed as the means ± standard error of the mean (SEM). Differences between the mean values of normally distributed data were assessed with one-way AVOVA (Dunnett’s t test) and two-tailed unpaired Student’s t test. Statistical difference was accepted at p < 0.05.
Results
Effects of mogroside V on the inflammatory cell count in the BALF of LPS-induced ALI mice
The number of inflammatory cell (i.e., total cells, neutrophils and macrophages) in the BALF was significantly elevated in LPS-induced mice versus control mice. Mogroside V (5 and 10 mg/kg) decreased the total cell number, as well as the number of neutrophils and macrophages (), as compared to the LPS alone group.
Figure 2. Effects of mogroside V on the number of total cells, neutrophils and macrophages in the BALF of LPS-induced ALI mice. Inflammatory cell counts in BALF obtained from challenged mice 12 h after LPS administration. Different cell counts were identified macrophage and neutrophil: (a) control (PBS treated mice); (b) mogroside V treated mice; (c) LPS-challenged mice; (d) LPS-challenged mice treated with mogroside V (2.5 mg/kg); (e) LPS-challenged mice treated with mogroside V (5 mg/kg); (f) LPS-challenged mice treated with mogroside V (10 mg/kg); (g) LPS-challenged mice treated with dexamethasone (2 mg/kg). The values presented are the means ± SEM (n = 5 in each group) of three independent in vivo experiments. *p < 0.05, **p < 0.01 versus LPS group.
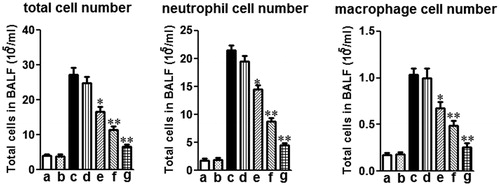
Effects of mogroside V on TNF-α, IL-1β and IL-6 levels in the BALF of LPS-induced ALI mice
TNF-α, IL-1β and IL-6 levels in the BALF were evaluated 12 h after LPS administration. As shown in , LPS markedly increased the levels of TNF-α, IL-1β and IL-6 compared to the control group. Treatment with mogroside V (5 and 10 mg/kg) and dexamethasone significantly decreased TNF-α, IL-1β and IL-6 production compared to those in the LPS alone group ().
Figure 3. Effects of mogroside V on production of inflammatory cytokines TNF-α, IL-1β, IL-6 and IL-8 in the BALF of LPS-induced ALI mice. Mice were given an oral administration of mogroside V 1 h prior to an i.n. administration of LPS. BALF was collected at 12 h following LPS challenge to analyze the inflammatory cytokines TNF-α, IL-1β, IL-6 and IL-8. The values presented are the means ± SEM (n = 5 in each group) of three independent in vivo experiments. *p < 0.05, **p < 0.01 versus LPS group.
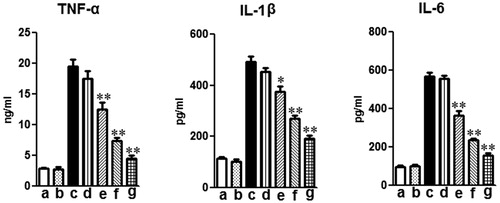
Effects of mogroside V on LPS-induced lung W/D ratio
Twelve hours after LPS administration, the lung W/D ratio was evaluated for edema by determining the lung water content. As shown in , administration of LPS caused a significant increase in the wet-to-dry weight (W/D) ratio compared to the control group (**p < 0.01). However, treatment with either mogroside V (5 and 10 mg/kg) or dexamethasone (2 mg/kg) significantly decreased the lung W/D ratio (*p < 0.05) compared to the LPS alone group.
Figure 4. Effects of mogroside V on the lung W/D ratio of LPS-induced ALI mice. Mice were given an oral administration of mogroside V 1 h prior to an i.n. administration of LPS. The lung W/D ratio was determined at 12 h after LPS challenge. The values presented are the means ± SEM (n = 5 in each group) of three independent in vivo experiments. *p < 0.05 versus LPS group.
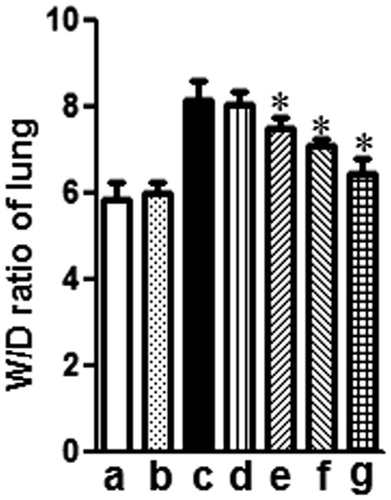
Effects of mogroside V on MPO activity in lung tissue from mice with LPS-induced ALI
PMNs are the major components of inflammatory and immunological reactions in injured lungs. Increases in MPO activity reflect PMN accumulation in the lung and play an important role in the development of ALI. As shown in , 12 h of LPS treatment significantly increased lung MPO activity compared to the control group (*p < 0.01). This increase was apparently reduced by treatment with mogroside V (5 and 10 mg/kg) or dexamethasone (2 mg/kg).
Figure 5. Effects of mogroside V on MPO activity of LPS-induced ALI mice. Mice were given an oral administration of mogroside V 1 h prior to an i.n. administration of LPS. MPO activity was determined at 12 h after LPS challenge. The values presented are the means ± SEM of three independent in vivo experiments. *p < 0.05, **p < 0.01 versus LPS group.
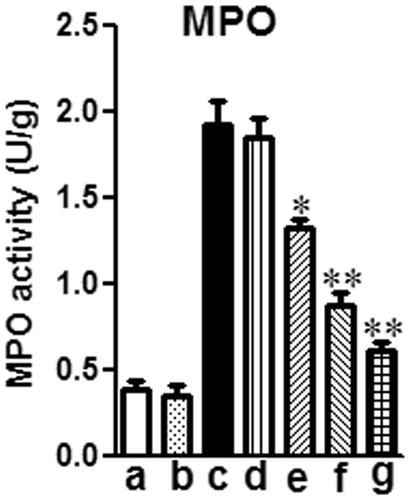
Effects of mogroside V on histological changes in lung tissue
To assess the anti-inflammatory effect of mogroside V on the airway, histopathological studies were performed. Inflammatory cell infiltration in the peribronchial and perivascular areas (H&E staining) was observed in LPS-challenged mice (). However, LPS-induced pathological changes were significantly attenuated by treatment with either mogroside V (5 and 10 mg/kg) or dexamethasone (2 mg/kg).
Figure 6. (A) Effects of mogroside V on histological changes in lung tissues. Mice were given an oral administration of mogroside V 1 h prior to an i.n. administration of LPS. Lungs were processed for histological evaluation of lung tissue using HE staining at 12 h after LPS challenged (magnification 100×). (B) Effects of mogroside V on lung morphology. The slides were histopathologically evaluated using a semi-quantitative scoring method. Lung injury was graded from 0 (normal) to 4 (severe). Mean scores were obtained from five mice. *p < 0.05, **p < 0.01 versus LPS group.
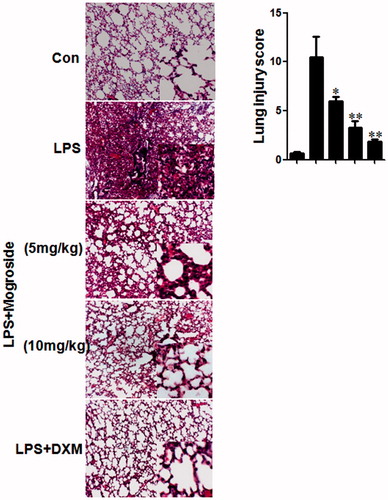
Effects of mogroside V on lung iNOS, COX-2 and NF-κB expression in LPS-induced ALI mice
Western blot analysis showed that the expression of iNOS, COX-2 and NF-κB in lung tissue was increased in the LPS alone group. Pre-treatment with mogroside V (5 and 10 mg/kg) and dexamethasone (2 mg/kg) inhibited the expression of iNOS, COX-2 and NF-κB (). To gain further insight into the mechanism of mogroside V-mediated regulation of NF-κB in vivo, we examined the effect of mogroside V on IκBα phosphorylation and degradation. As shown in , LPS-induced IκBα degradation was significantly blocked by pre-treatment with mogroside V, suggesting that the mechanism of action is related to IκBα phosphorylation.
Figure 7. Effects of mogroside V on lung iNOS, COX-2 and NF-κB expression in LPS-induced ALI mice. Immunoblotting of iNOS, COX-2 and NF-κB in proteins extracts of lung tissues isolated from mice 12 h after the LPS challenge pretreated with mogroside V β-actin was used as an internal control. *p < 0.05, **p < 0.01 versus LPS group.
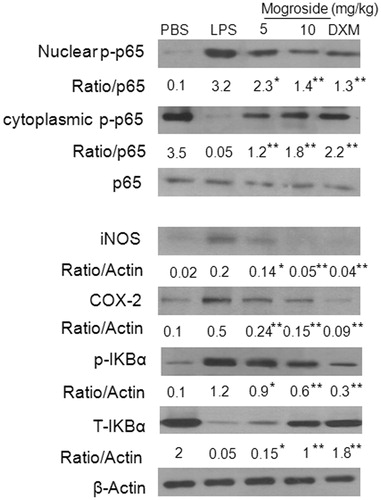
Discussion
ALI and ARDS, both of which are caused by Gram positive and Gram negative bacteria, are common symptoms that can lead to substantial morbidity and mortality. ALI is characterized by a systemic airway inflammatory response including cytokines (e.g., TNF-α, IL-6, IL-8), chemokines, pro-inflammatory mediators and a variety of cells, all of which regulate the migration and pulmonary infiltration of neutrophils into the interstitial tissue (Shields et al., Citation2002). Even in patients who survive ALI, there is evidence that their long-term quality of life is adversely affected (Dowdy et al., Citation2006; Rubenfeld et al., Citation2005). LPS, the main component of the outer membranes of Gram negative bacteria, might be the most important antigen that promotes the development of ALI. In our study, we used a classic mouse model with intra-tracheal administration of lipopolysaccharide (LPS, endotoxin), a component of the Gram-negative bacterial outer cell wall, to induce a pulmonary inflammatory state that recapitulates human ALI (Saluk-Juszczak & Wachowicz, Citation2005). Mogroside V, an extract from Momordica grosvenori, is a traditional Chinese medicine that has been reported to have anti-inflammatory activities in murine macrophages and a murine ear edema model (Cribbs et al., Citation2010). In the current study, we investigated the effect of mogroside V on LPS-induced ALI mice. Our results show that treatment with mogroside V significantly decreased inflammatory cell numbers, pro-inflammatory cytokine production, W/D ratio, MPO activity, lung histological damage and expression of iNOS, COX-2 and NF-κB.
TNF-α, IL-1β and IL-6, characterized cytokines involved in the inflammatory process of ALI (Goodman et al., Citation2003), are released mainly from LPS-stimulated monocytes and macrophages. These cytokines can recruit neutrophils into the pulmonary microvasculature and promote their migration into the lung parenchyma, which is critical for host defense, and contribute to the development of ALI (Szarka et al., Citation1997). In this study, we evaluated the levels of inflammatory cell numbers and pro-inflammatory cytokines 12 h after LPS administration and found that the number of inflammatory cells (total cell number, neutrophils and macrophages) and levels of pro-inflammatory cytokines (TNF-α, IL-1β and IL-6) in the BALF of the LPS alone group were significantly increased; however, treatment with mogroside V resulted in a significant decrease in the levels of inflammatory cell numbers and pro-inflammatory cytokines. iNOS and COX-2 play an important role in ALI (Fukunaga et al., Citation2005). Increased iNOS and COX-2 expression in lung tissue are found in our ALI mice 12 h after LPS administration. Mogroside V can significantly decrease the iNOS and COX-2 expression. Additionally, in lung histological examination, we also found a decrease in the number of inflammatory cells. These results suggest that the protective effects of mogroside on ALI may be attributable to the inhibition of inflammatory factors.
Edema is a representative characteristic of inflammation (Yingkun et al., Citation2013). Mogroside V has been reported to inhibit ear edema in murine model. To investigate the effect of mogroside V on pulmonary edema, we determined the W/D ratio of sampled lung tissue. Our results showed that mogroside V can decrease the W/D ratio compared to the LPS alone group, suggested the inhibitory effect of mogroside V on the lung edema.
The transcription factor NF-κB, regulates the expression of a large number of inflammatory genes, is involved in the pathogenesis of ALI and is known as an important target for anti-inflammatory molecules (Wright & Christman, Citation2003). Under resting conditions, NF-κB is located in the cytoplasm bound to IκB. However, NF-κB can be activated by some stimulation of various receptors including TNF receptor, Toll-like receptors (TLRs) and T-cell receptor (TCR). Once activated, they release inflammatory cytokines and mediators, such as TNF-α, IL-1β and IL-6, NO and PGE2 (Moreno et al., Citation2004). In this study, we observed that mogroside V can inhibit the phosphorylation and degradation of IκBα, and further blocked NF-κB p65 activation. Therefore, the inhibitory effect of mogroside V on ALI could be attributed to its ability to block NF-κB activation induced by LPS.
Conclusions
In summary, the findings of the present study suggest that mogroside V was able to decrease inflammatory cell infiltration into the lung tissue, the production of pro-inflammatory cytokines, the W/D ratio, MPO activity, histological change and expression of iNOS and COX-2. Moreover, the protective effects of mogroside V are associated with the attenuation of inflammatory reactions, and the mechanism may involve blocking the activation of the NF-κB signalling pathway.
Declaration of interest
The authors state no conflicts of interest.
Acknowledgements
This work was supported by a grant from the Natural Science Foundation of Jilin (Nos. 2012. 225 and 2012. 05).
References
- Agouridakis P, Kyriakou D, Alexandrakis MG, et al. (2002). The predictive role of serum and bronchoalveolar lavage cytokines and adhesion molecules for acute respiratory distress syndrome development and outcome. Respir Res 3:25
- Bhatia M, Moochhala S. (2004). Role of inflammatory mediators in the pathophysiology of acute respiratory distress syndrome. J Pathol 202:145–56
- Bosma K, Fanelli V, Ranieri VM. (2005). Acute respiratory distress syndrome: Update on the latest developments in basic and clinical research. Curr Opin Anaesthesiol 18:137–45
- Cribbs SK, Matthay MA, Martin GS. (2010). Stem cells in sepsis and acute lung injury. Crit Care Med 38:2379–85
- Di R, Huang MT, Ho CT. (2011). Anti-inflammatory activities of mogrosides from Momordica grosvenori in murine macrophages and a murine ear edema model. J Agric Food Chem 59:7474–81
- Dowdy DW, Eid MP, Dennison CR, et al. (2006). Quality of life after acute respiratory distress syndrome: A meta-analysis. Intensive Care Med 32:1115–24
- Fukunaga K, Kohli P, Bonnans C, et al. (2005). Cyclooxygenase 2 plays a pivotal role in the resolution of acute lung injury. J Immunol 174:5033–9
- Goodman RB, Pugin J, Lee JS, Matthay MA. (2003). Cytokine-mediated inflammation in acute lung injury. Cytokine Growth Factor Rev 14:523–35
- Greenberger PA. (2008). Immunologic lung disease. J Allergy Clin Immunol 121:S393–7
- Ingram RH Jr. (1995). Relationships among airway-parenchymal interactions, lung responsiveness, and inflammation in asthma. Giles F. Filley Lecture. Chest 107:148S–52S
- Ishii Y. (2011). Acute lung injury/acute respiratory distress syndrome: Progress in diagnosis and treatment topics. I: Pathogenesis and pathophysiology; II: Etiology and epidemiology. Nihon Naika Gakkai Zasshi 100:1522–8
- Ito K, Jazrawi E, Cosio B, et al. (2001). p65-Activated histone acetyltransferase activity is repressed by glucocorticoids: Mifepristone fails to recruit HDAC2 to the p65-HAT complex. J Biol Chem 276:30208–15
- Johnson ER, Matthay MA. (2010). Acute lung injury: Epidemiology, pathogenesis, and treatment. J Aerosol Med Pulm Drug Deliv 23:243–52
- Karin M. (1999). The beginning of the end: IkappaB kinase (IKK) and NF-kappaB activation. J Biol Chem 274:27339–42
- Karin M, Ben-Neriah Y. (2000). Phosphorylation meets ubiquitination: The control of NF-[kappa]B activity. Annu Rev Immunol 18:621–63
- McKay A, Leung BP, McInnes IB, et al. (2004). A novel anti-inflammatory role of simvastatin in a murine model of allergic asthma. J Immunol 172:2903–8
- Moreno C, Merino J, Vazquez B, et al. (2004). Anti-inflammatory cytokines induce lipopolysaccharide tolerance in human monocytes without modifying toll-like receptor 4 membrane expression. Scand J Immunol 59:553–8
- Opitz B, van Laak V, Eitel J, Suttorp N. (2010). Innate immune recognition in infectious and noninfectious diseases of the lung. Am J Respir Crit Care Med 181:1294–309
- Qi XY, Chen WJ, Zhang LQ, Xie BJ. (2008). Mogrosides extract from Siraitia grosvenori scavenges free radicals in vitro and lowers oxidative stress, serum glucose, and lipid levels in alloxan-induced diabetic mice. Nutr Res 28:278–84
- Rubenfeld GD, Caldwell E, Peabody E, et al. (2005). Incidence and outcomes of acute lung injury. N Engl J Med 353:1685–93
- Saluk-Juszczak J, Wachowicz B. (2005). The proinflammatory activity of lipopolysaccharide. Postepy Biochem 51:280–7
- Shields CJ, Winter DC, Redmond HP. (2002). Lung injury in acute pancreatitis: Mechanisms, prevention, and therapy. Curr Opin Crit Care 8:158–63
- Szarka RJ, Wang N, Gordon L, et al. (1997). A murine model of pulmonary damage induced by lipopolysaccharide via intranasal instillation. J Immunol Methods 202:49–57
- Wright JG, Christman JW. (2003). The role of nuclear factor kappa B in the pathogenesis of pulmonary diseases: Implications for therapy. Am J Respir Med: Drugs Devices Other Interventions 2:211–19
- Yingkun N, Zhenyu W, Jing L, et al. (2013). Stevioside protects LPS-induced acute lung injury in mice. Inflammation 36:242–50
- Zhang X, Song K, Xiong H, et al. (2009). Protective effect of florfenicol on acute lung injury induced by lipopolysaccharide in mice. Int Immunopharmacol 9:1525–9