Abstract
Context: Excess use of alcohol is known to be associated with liver diseases such as fatty liver, alcoholic hepatitis, and cirrhosis. Various practices may be applied to prevent or treat the damage caused by chronic alcoholism. Coprinus comatus (O.F. Müll.) Pers. (Agaricaceae) is a macrofungus that has been reported to aid the recovery of murine livers damaged by benzopyrene.
Objective: In this study, the possible therapeutic effects of three different doses (50, 100, and 150 mg/kg) of C. comatus polysaccharide (PS) extract were studied in rats subjected to an alcoholic diet. The histological and biochemical results were compared between the control and experimental groups.
Materials and methods: Modified Lieber–Decarli’s calorie-adjusted liquid alcohol diet was given orally for 60 d. In addition to histopathology, alanine transaminase (ALT), aspartate transaminase (AST), mitochondrial membrane integrity, total cytochrome-c oxidase activity (TotalStCox), total mitochondrial cytochrome-c oxidase activity (TotalMtStCox), and caspase-3 values were used as liver parameters, and liver sections from all experimental groups were examined by electron microscopy.
Results: Using histopathological assessment, it was observed that there was a decline in liver hepatocyte vacuolization in the treatment group fed 50 mg PS/kg. The TotalStCox and TotalMtStCox values of this group differed from the EtOH control group (p < 0.05).
Discussion and conclusion: Daily administration of 50 mg/kg of C. comatus PS extract considerably reduced the negative effects of alcohol on liver structure and function.
Introduction
In recent years, alcohol consumption has increased rapidly all over the world, and alcoholic liver disease has become a major social and health problem (SriRajaskanthan & Preedy, Citation2006; Yuan et al., Citation2007). The metabolic activity of the liver is primarily affected by alcohol consumption. Setting aside the contribution of the stomach, the main organ responsible for alcohol catabolism is liver.
Various synthetic drugs have been used to prevent or treat alcoholic liver damage. However, they all have various adverse effects if used continuously. It is, therefore, necessary to apply an alternative medical approach for the treatment. Natural compounds are found to be attractive alternatives because of their effectiveness, limited side effects, and low cost (Hwang et al., Citation2005). Because of their various biological activities, mushrooms have been used as natural drugs in ancient Oriental countries and in Eastern and Mesoamerican cultures (Lindequist et al., Citation2005; Wasser, Citation2002). Polysaccharides (PSs) are considered to be important components of mushrooms because they have antitumor (Daba & Ezereonye, Citation2003; Wasser, Citation2002), antibacterial, hypolipidemic (Yang et al., Citation2000), hypoglycemic (Wayhs et al., Citation2013; Yamac et al., Citation2008, Citation2009), and immunomodulatory (Lindequist et al., Citation2005; Wasser, Citation2002) properties. Some mushroom PSs are commercially available, including Lentinan (Chihara et al., Citation1970), Schizophyllan (Tabata et al., Citation1981), and Krestin (Tsukagoshi, Citation1974).
Several species of mushrooms, such as Agaricus brasiliensis Fr. (Agaricaceae), Grifola frondosa (Dicks.) Gray (Meripilaceae), Lentinula edodes (Berk.), Pegler (Marasmiaceae), Phellinus linteus (Berk. & M.A. Curtis) Teng (Hymenochaetaceae), Macrocybe lobayensis (R. Heim) Pegler & Lodge (Tricholomataceae) and Trametes versicolor (L.) Lloyd (Polyporaceae), have been investigated for their hepatoprotective and healing activities in liver disorders induced by different agents (Eidi et al., Citation2013; Wang et al., Citation2012). However, the species of mushrooms that have been studied for that purpose are very limited. In this study, the PS extract of the edible mushroom Coprinus comatus (O.F. Müll.) Pers. (Agaricaceae) was investigated for possible beneficial effects on liver disease induced by alcohol.
Materials and methods
Preparation of the PS extract
Coprinus comatus (O.F. Müll.) Pers. (Agaricaceae) strain HORST 4030 carpophores was harvested and dried at 50 °C. Then, the dried carpophores were ground into fine powder; 50 g of powdered carpophore was resuspended in 1 L of water and autoclaved at 120 °C. The resulting suspension was cooled and centrifuged at 7000 rpm for 15 min to obtain a clear extract, which was then concentrated to 50 mL by boiling. Two volumes of 96% ethanol were added to the solution, and the mixture was kept at 4 °C overnight to precipitate the PSs. The resulting precipitate was centrifuged, collected, and solubilized in a low volume of water. The residual solid particles were removed through centrifugation, and the PSs were reprecipitated in two volumes of 96% ethanol. The precipitate was collected through centrifugation and vacuum-dried for further use in this study.
Experimental design
In this study, all the animals were treated according to the National Institutes of Health guidelines, using the protocols approved by the Eskisehir Osmangazi University Institutional Animal Care and Use Committee. This study was conducted upon receipt of the approval, numbered 179/2010, from the Eskisehir Osmangazi University, Medicine Faculty. Healthy, 3-month-old male Sprague–Dawley rats weighing 220 ± 20 g were used. The animals were randomly divided into five groups, with eight rats in each group. All the test animals were supplied by the Laboratory on Production of Test Animals, Refik Saydam Hygiene Institute, Turkish Republic Ministry of Health. During the experiment, these animals were kept in transparent polycarbonate cages in rooms with 12:12 illuminated/dark light settings, and the heat (22 ± 2 °C) and atmospheric humidity (45–50%) were automatically adjusted.
Throughout the 60-day course of the experiment, our modified, calorie-adjusted liquid ethyl alcohol (EtOH) diet, as described by Lieber et al. (Citation1989), was given orally. The total calorie content (based on approximately 1000 kcal) of the applied liquid diet consisted of 40% fat, 36% ethanol, 20% protein, and 4% carbohydrates. Liquid diet was prepared fresh and daily adjustment of the calories was conducted with dextrin. Group I animals were given an alcohol-free diet for 60 days, and the rest of the groups were given the same diet for the first week only. The prepared diet was put in the water bowls of the cages once a day and the rats were fed ad libitum. Except for Group I, 96.5% ethyl alcohol (EtOH) (density (d) = 0.81 g/cm3) was added to the bowl of all the other groups. The alcohol was gradually increased each week, from an initial percentage of 2.4 to −4.8, and finally 7.2%. In the remainder of the experimental period, the diet was continued with 7.2% alcohol (Uzbay et al., Citation2006). The experimental animals from Group II were administered 1 mL of 0.9% NaCl each day between days 54 and 60. Three doses of PS (50, 100, or 150 mg/kg/day) from Coprinus comatus were given orally through gavage to the test animals in Groups III, IV, and V, respectively, by dissolving it in 1 mL of 0.9% NaCl.
The EtOH diet was given between 9:00 am and 10:00 am each day, and the amount consumed was recorded for all the experimental groups. The animals’ daily EtOH consumption was calculated according to the following formula: A = dx[(V × 75)/W] (Uzbay et al., Citation2006) where A is the g/kg/day EtOH consumption, d is the specific weight (density) of 96.5% EtOH (0.81 g/cm3 at 25 °C), V is the quantity of liquid diet consumed on a daily basis (mL), W is the weight of test animal (g).
At the end of the experimental process, all the experimental animals were dissected under ether anesthesia and were then sacrificed by drawing all the blood from the heart. Serum samples were obtained from the blood for biochemical analyses.
Following the sacrifice of each experimental animal, the entire liver was taken out and a piece was placed in the freezer in polyethylene tubes at −80 °C for biochemical analyses. Liver tissues of standard weight were taken for fresh mitochondrial analyses. The rest of the liver tissue samples were fixed for histological and TEM studies.
Biochemical analysis
To identify the dysfunctions in the liver cells, alanine transaminase (ALT) and aspartate transaminase (AST) were measured in the serum samples using a Roche/Hitachi MODULAR P autoanalyzer and Roche commercial kits (Roche Molecular Systems, Branchburg, NJ).
Caspase-3 activities in liver tissues were determined spectrophotometrically by measuring the absorbance of pNA at 405 nm using a colorimetric caspase-3 measurement kit (Sigma, St. Louis, MO). Hydrolysis of pNA by caspase-3 generates acetyl–Asp–Glu–Val–Asp–p-nitroanilide (AC-DEVD-pNA), according to Zovein et al. (Citation2004). The results were expressed by normalizing to the amount of tissue protein.
Isolation of mitochondria from liver tissue and the determination of mitochondria inner and outer membrane integrities were conducted with a mitochondria isolation kit (Sigma, St. Louis, MO) and a cytochrome oxidase kit (Sigma, St. Louis, MO). The mitochondrial membrane potential (ΔΨ) was determined by measuring the inner mitochondrial membrane integrity (JC-1 absorbance in mitochondria) and expressed in mitochondrial protein fluorescence units (FLU/mgP) produced in the mitochondrial suspension.
To determine the mitochondrial outer membrane integrity and the amount of mitochondria in subcellular fractions, the StCox analysis was conducted using a commercial kit (Sigma, St. Louis, MO). The StCox kit uses an optimized colorimetric assay based on the observation of a decrease in the absorbance of ferrocytochrome-c, measured at 550 nm, which is caused by the oxidation of ferrocytochrome-c to ferricytochrome-c. The integrity of the mitochondrial outer membrane was estimated as a percentage of the activity difference occurring in the presence of n-dodecyl-β-d-maltoside (TotalStCox activity), which is a detergent, or in its absence (StCox activity in intact mitochondria). Tissue protein measurements were conducted by the biuret method (Layne, Citation1957).
Histological and TEM studies
After 60 d, the animals were sacrificed, and the liver tissue from each animal was collected and fixed in 10% neutral formaldehyde for histological analyses. After fixation, the tissues were mounted in paraffin blocks through a routine procedure. Four to six micrometer sections of tissue were cut from these blocks for standard hematoxylin & eosin (H&E) staining. Histopathological examination was performed for all these preparations.
Liver sections (1 mm3) from each animal were taken for ultrastructural examination, and TEM tissue follow-up was performed. The first and second fixations were performed with 4% glutaraldehyde and 2% osmium tetroxide, respectively. Following the alcohol series and propylene oxide treatments, Epon 512 was used to embed the sections. The ultrasections were stained with uranyl acetate and lead citrate, and the sections were examined with a JEOL-JEM-1220 Transmission Electron Microscope (JEOL Ltd, Tokyo, Japan). Pictures were taken with an Olympus Megaview G2 model camera (Olympus FV10i, Tokyo, Japan).
Statistical analysis
SPSS 12.0 (SPSS Inc., Chicago, IL) was used to assess the data from our studies. For inter-group comparisons, the LSD method was used. The Mann–Whitney U test was used to compare the scores from the inter-group histopathological assessments. The statistical data from each experimental group were expressed as the mean ± standard error (SE). Differences between the experimental groups were deemed significant if p < 0.05.
Results
The daily EtOH amounts received by each animal are shown in , and they were found to be statistically similar.
Figure 1. EtOH consumption levels for animals in all groups. The data are shown as the mean ± SE values (n = 8).
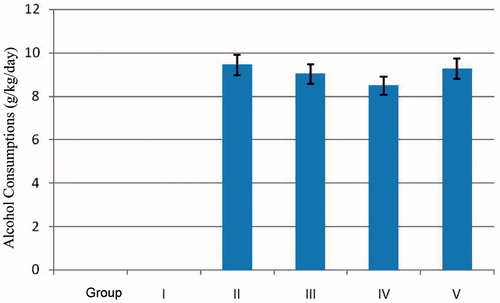
The serum ALT and AST levels of animals in Groups I–V were subjected to a comparative assessment among the groups (). It was found that the serum ALT activities of Group I (control group), Group II (EtOH control group), and Group III (EtOH + 50 mg/kg PS) were similar, while there were statistically significant differences between Group IV (EtOH + 100 mg/kg PS), Group V (EtOH + 150 mg/kg PS), and Group I (p < 0.05). Similarly, it was observed that Groups IV and V were different from Group II (p < 0.05). There was a statistically significant difference between Groups I and IV (p < 0.001). For the serum AST activities, Group IV was significantly different from control Groups I and II (p < 0.05).
Table 1. ALT and AST levels in serum.
For caspase-3 values, there were no significant differences found between any of the groups (). The values of inner and outer mitochondrial membrane integrities that were isolated from the liver tissues of the animals in the experimental groups are given in . The inter-group comparisons showed that Groups III, IV, and V were different from the non-treated control Group I (p < 0.05). Group I was significantly different from the alcoholic control Group II (p < 0.001) (). The TotalStCox activity of Groups I and II was significantly different from that of Group III (50 mg/kg PS), Group IV (100 mg/kg PS), and Group V (150 mg/kg PS) (p < 0.001) (). The TotalMtStCox activity of Group I was different from Groups IV and V (p < 0.05). Group III was found to be significantly different from Groups I and II (p < 0.001) ().
Table 2. The activity levels of caspase-3, mitochondrial inner membrane load, mitochondrial outer membrane integrity, TotalStCox activity, and TotalMtStCox.
Histopathological analyses
The histological changes of the liver are shown in . Livers of the animals in Group I had a normal lobular structure (). However, in the Group II liver sections, expansions occurred in the sinusoids (). Advanced degeneration and polymorphonuclear leukocyte (PMNL) infiltration () were observed in the Group III sections. Expansive and large vacuoles were also observed in the liver parenchyma of Group II rats ().
Figure 2. Histopathological changes: (A) control rat liver. (B, C, and D) Alcohol-treated rat liver.
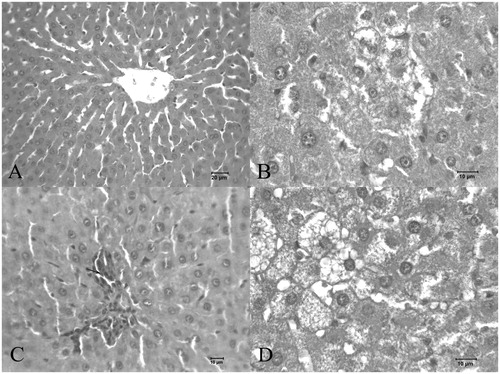
Table 3. Histopathologic evaluations for experimental groups (n = 8).
In the liver sections from Group III animals, the histological structure was very similar to that of Group I, and the vacuolization decreased in the hepatocyte cytoplasm and parenchyma compared to Group II. No PMNL infiltration or expanded sinusoids were observed in the liver sections from Group III ().
Figure 3. Histopathological changes in (A) alcohol + 50 mg/kg PS-treated rat liver; (B) alcohol + 100 mg/kg PS-treated rat liver; (C and D) alcohol +150 mg/kg PS-treated rat liver.
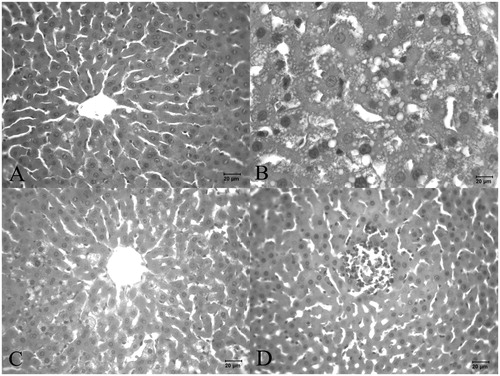
In the liver sections from Group IV animals, vacuolization in the liver parenchyma of the hepatocyte cytoplasm was significantly increased, and hepatocyte degeneration and sinusoidal expansion were common ().
In the histological analyses of the Group V liver sections, it was observed that hemorrhage and PMNL infiltration were increased in the sinusoidal area. The sinusoidal expansions occurred in the vena centralis area of the liver, and necrotic areas were common in the tissue. Additionally, vacuolation occurred in the hepatocytes ().
TEM analysis
In thin sections of the liver tissues from Group I, it was observed that organelle structures such as the mitochondria were normal ().
Figure 4. Transmission electron micrograph of hepatocytes obtained from the liver of control rats given water and food (N, nucleus; M, mitochondria).
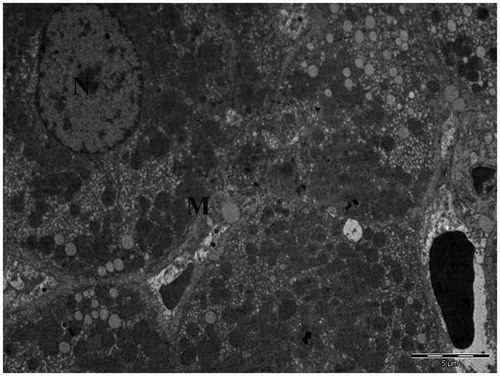
Thin liver sections from the animals in Group II showed an increase in lipid droplets that resulted in clusters, and the ultrastructural integrity of the cells was lost (). Abnormal mitochondria changes and the expansion and melting of cristae were also observed. In addition, swelling and fractures were present in the smooth endoplasmic reticulum ().
Figure 5. (A) Lipid droplets, (B) melting of the structure of the mitochondrial cristae in the liver sections from Group II, (C and D) normal mitochondria and small lipid droplets in Group III, (E) lipid droplets in Group IV, (F) lipid droplets and swollen mitochondria in hepatocytes from Group V.
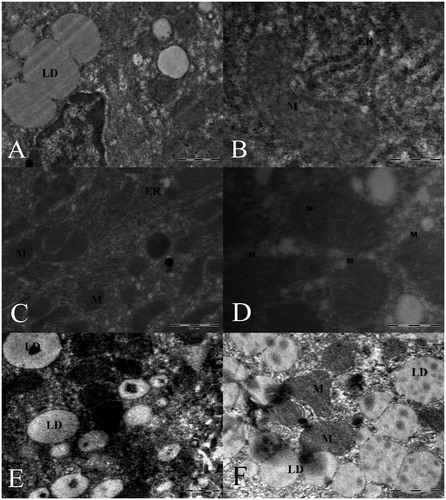
In Group III, therapeutic effects were observed in the thin liver structure. The structural morphology was similar to that of the control group (Group I). Large lipid droplets were not observed in the hepatocytes (). The endoplasmic reticulum of Group IV was expanded and the structures of the mitochondria and mitochondrial cristae were disrupted. There was also an increase in lipid vacuoles (). In Group V, there was an increase in the number of lipid droplets; additionally, the mitochondria were swollen, and the structure of the mitochondrial cristae was disrupted. There were fractures in the smooth endoplasmic reticulum ().
Discussion
Disruption of liver cell integrity and degeneration of cells affects liver enzyme levels (Castilla et al., Citation2004; Kanbak et al., Citation2001). It was found that the serum ALT levels in Group II (EtOH control group) were higher than those in Group I (control group). However, this difference was not considered to be significant. In the PS groups, the Group IV (100 mg PS/kg) and Group V (150 mg PS/kg) ALT levels were elevated when compared to those of the control group (p < 0.05). This increase was not significant. In various clinical and experimental studies, it was reported that alcohol-induced serum ALT levels were elevated during alcoholic liver disease (Kim et al., Citation2009; Pari & Suresh, Citation2008; Zhou et al., Citation2001). For the AST levels, there was a decline in Group II (EtOH control group), while there was an increase in the PS groups.
EtOH-induced liver injury is characterized by increased formation of ROS and inflammatory cytokines. The result of this development is hepatic steatosis, injury, and necrotic or apoptotic cell death (Cohen et al., Citation2009). In the alcohol-induced liver damage established experimentally, we showed that caspase-3 activation was one of the indicators of apoptosis. There are numerous studies demonstrating that EtOH causes cell death by inducing caspase-3 activity (Olney et al., Citation2002; Young et al., Citation2005). Das et al. (Citation2011) have shown that long-term EtOH consumption increases caspase-3 activity in rat liver tissue. Zhou et al. (Citation2001) have also found that exposure to ethanol caused an increase in caspase-3 activation in rat liver tissue. Although not statistically significant, the caspase-3 activity in Group II, to which alcohol was administered, was higher than that in the other groups. In addition, the caspase-3 values in control Groups I and V were very similar. Therefore, a treatment effect was observable.
Ethanol has the ability of increasing the effectiveness of caspase-3. However, caspase-3 activation was sometimes affected by factors, such as ethanol and lipopolysaccharide (LPS). Thus, there cannot be a significant increase in caspase-3 activity or even it might decrease. In a study conducted on tolerance mice induced with LPS, hepatic apoptosis is supprased compared to the control group rats and the activity and expression of caspase-3 are down-regulated (Luan et al., 2007).
An in vivo study investigating the effects of the use of alcohol on the pancreas death signals reported that caspase-8 gene expression decreased, and depending on this, caspase-3 activity decreased (Wang et al., 2006). Koteish et al. (Citation2002) report that the administration of LPS to rats fed with normal diet show histological evidence of increased apoptosis with an increase in caspase-3 activity in the short term; however, similar situation was observed in rats exposed to alcohol. However, after the administration of LPS to rats exposed to alcohol, histological liver damage has been observed much more than the control group fed with normal diet and administered with LPS. In that case, ethanol might inhibit the activity of hepatic caspase-3.
It has been demonstrated that chronic EtOH consumption can cause changes in the mitochondrial structure and function of experimental animals on a subcellular level (Venkatraman et al., Citation2003). Mitochondrial factors such as membrane potential and membrane permeability are believed to be regulatory mechanisms of cellular mitochondrial activity. Deterioration in the mitochondrial membrane potential was also observed in the hepatocytes of rats exposed to alcohol. Additionally, an increase in mitochondrial membrane permeability has previously been reported (Gear, Citation1970).
Oxidative stress occurring as a result of alcohol consumption induces mitochondrial permeability and can also cause caspase activation and initiation of apoptosis mechanism due to cytochrome-c release (Ishii et al., Citation2003).
Adachi and Ishii (Citation2002) demonstrated that acute alcohol consumption caused mitochondria-mediated apoptosis. In our study, the integrity of the outer membrane was assessed by measuring StCox activity in the mitochondrial membranes. The Group II StCox activity was significantly lower than the control group. However, no significant differences were observed in the mitochondrial outer membrane integrity between the groups. Kim et al. (Citation2009) noted that alcohol decreased the StCox mRNA release.
In this study, the integrity of the inner mitochondrial membrane was examined by measuring the electrochemical proton gradient (ΔΨ). ΔΨ was found to be lower in Group II, where we conducted the alcohol application (p < 0.05). The decrease of ΔΨ in the ethanol group demonstrated a deterioration of the mitochondrial permeability transition (MPT) and the release of cytochrome-c, which usually precedes apoptosis. Guan et al. (Citation2004), who studied the effect of high-dose ethanol, noted that intracellular types of ROS formed as a result of EtOH decreased the mitochondrial membrane potential. They determined that higher cytochrome-c content was present in the cytosol of groups that were given ethanol. Yan et al. (Citation2007) also showed that the long-term consumption of EtOH decreased the transmembrane potential. We believe that EtOH affects MPT through oxidative stress because one of the major targets of free oxygen radicals in hepatocytes exposed to ethanol is the mitochondrial respiratory chain (Kaplowitz & Tsukamoto, Citation1996). EtOH intoxication causes mitochondrial depolarization. EtOH-induced apoptosis in hepatocytes is mediated by changes in MPT and by the decrease in mitochondrial membrane potential. Cytochrome-c release, which may occur as a result of mitochondrial damage, leads to caspase activation. This effect was observed in a study on hepatocytes by Higuchi et al. (Citation2001). Caspase activation and apoptosis initiation could be prevented by the interception of cytochrome-c release of MPT inhibitors like Cys-A (cyclosporin A). Cytochrome-c release may occur as a result of mitochondrial damage, and it leads to caspase activation, as observed by Higuchi et al. (Citation2001). In the groups that received PS, there was a significant increase in ΔΨ compared to Group II due to the improvement in mitochondrial transmembrane potential (p < 0.05). In light of this finding, PSs obtained from C. comatus applied for 100 mg/kg/day appear to contribute to decrease of experimental alcoholic liver damage.
There are many scientific studies on alcoholic liver disease. Most of these studies examined the liver function through biochemical tests on liver tissues, histopathological analyses, and thin structure assessments. Depending on the use, amount, and frequency of alcohol consumption, significant structural and functional changes can occur in the liver (Jaeschke et al., Citation2002; Lieber, Citation2004).
The histopathological assessment in our study demonstrated that the initial symptoms of an alcoholic liver were present in Group II (EtOH control group), including the formation of fat vacuoles and necrotic areas and the degeneration of hepatocytes. Our results are in agreement with those from analogous studies. These data indicate that our alcohol model is relevant (Das & Vasudevan, Citation2007). It was observed that there was a decline in the vacuolization of the liver hepatocytes in Group III. In this group, there was an increase in devacuolization at higher doses. Coprinus comatus PS used was a crude preparation that contained some protein and some polyphenols. The polyphenols, or PS and polyphenol complexes, can induce intracellular ROS. Therefore, we suggest that a higher dosage of crude PS could be toxic in our alcoholic liver model. Additionally, a 50 mg PS/kg dose may result in PMNL infiltration, an increase in the inflammatory response and the formation of free oxygen radicals. In the study performed by Das and Vasudevan (Citation2007), it was found that long-term alcohol exposure resulted in an increase in PMNL infiltration. In similar studies, adiposity and PMNL infiltration were considered to be histopathological findings (Hall et al., Citation2001; Ronis et al., Citation2004). Grissa et al. (Citation2007) reported that adiposity, inflammation, fibrosis, and necrosis occurred more often in an alcoholic group than a control group. Pari and Suresh (Citation2008) found degeneration, adiposity, and inflammatory cells. These symptoms are related to portal pathway stenosis and atherosclerosis in the alcoholic group. Additionally, several groups have observed intense adiposity in the livers of alcoholic rats (Pari & Suresh, Citation2008; Zhou et al., Citation2001).
The initial damage occurs because of changes in the mitochondrial morphology and functions, and this damage is dependent on alcohol consumption. The first stage of alcoholic liver pathogenesis is based on oxidative stress and is manifested by the loss of mitochondrial function (Fukumura et al., Citation2007).
In our study, it was observed that the mitochondria became swollen. Additionally, the cristae melted and were depleted in the liver parenchymal cells of animals that were fed an alcoholic diet. It was observed that smooth endoplasmic reticulum hypertrophy and fractures occurred in the alcohol group. We attributed this finding to an excessive generation of cytochrome P450 enzymes in the smooth endoplasmic reticulum of the alcoholic livers. Of the doses of PS given, it was observed that 50 mg/kg preserved the integrity of the mitochondrial external membrane and resulted in the vacuoles being present at a lower level. Any structural changes that were observed in the mitochondria may result in the oxidation of fatty acids and the accumulation of fat in cells.
In the study performed by Cameron and Neuman (Citation1999), an increase in the lipid-storage capacity of perisinusoidal stellate cells (lto) was observed. The number of lipid vesicles stored in lto cells increased by a 100-fold when compared to the control group (Cameron & Neuman, Citation1999). Fatty change indicates that abnormal fat accumulates in parenchymal cells. Small fat vacuoles in the cells indicate an absolute increase in intra-cellular lipids. This finding is indicative of damage, but it does not result in cell death. However, fatty liver change sometimes results in necrosis. In many cases, this effect may manifest itself around dead cells. In a similar study, it was found by EM that there were morphological changes, indicating disorders in the membranes of the liver parenchymal cells of rats exposed to alcohol (Ostrowskaa et al., Citation2004).
It is known that alcohol is a hepatotoxin that changes mitochondrial and microsomal functions. The manifestation of alcohol-dependent fatty liver depends on the following mechanisms: generation of free fatty acids at an elevated level, a decline in the use of triglycerides, a decline in the oxidation of fatty acids, a blockage of lipoprotein excretion, and an increase in lipolysis.
Based on our microscopy results, we suggest that a 50 mg/kg dose of C. comatus PS may provide therapeutic effects to livers damaged by alcohol. However, based on our biochemical results, it will be necessary to keep the PS dose under 50 mg/kg in subsequent studies because it is necessary to determine the proper range for the doses.
Declaration of interest
The authors report no conflicts of interest. The authors alone are responsible for the content and writing of this article.
Notes
This study was derived from the Ph.D. thesis of the first author.
References
- Adachi A, Ishii H. (2002). Role of mitochondria in alcoholic liver injury. Free Radic Biol Med 32:487–91
- Cameron RG, Neuman MG. (1999). Novel morphologic findings in alcoholic liver disease. Clin Biochem 32:579–84
- Castilla R, Gonzalez R, Fouad D, et al. (2004). Dual effect of ethanol on cell death in primary culture of human and rat hepatocytes. Alcohol Alcohol 39:290–6
- Chihara G, Himuri J, Maeda YY, et al. (1970). Fractionation and purification of the polysaccharides with marked antitumor activity. Cancer Res 30:2776–81
- Cohen JI, Roychowdhury S, Dibello PM, et al. (2009). Exogenous thioredoxin prevents ethanol-induced oxidative damage and apoptosis in mouse liver. Hepatology 49:1709–17
- Daba AS, Ezereonye OU. (2003). Anti-cancer effect of polysaccharides isolated from higher basidiomycetes mushrooms. African J Biotechnol 2:672–8
- Das SK, Vasudevan DM. (2007). Alcohol-induced oxidative stress. Life Sci 81:177–87
- Das SK, Mukherjee S, Vasudevan DM. (2011). Effects of long term ethanol consumption on cell death in liver. Indian J Clin Biochem 26:84–7
- Eidi A, Moghadam JZ, Mortazavi P, Olamafar S. (2013). Hepatoprotective effects of Juglans regia extract against CCl4-induced oxidative damage in rats. Pharm Biol 51:558–65
- Fukumura A, Tsutsumi M, Tsuchishima M, et al. (2007). Effect of the inducer of interleukin-6 (ME3738) on rat liver treated with ethanol. Alcohol Clin Exp Res 31:S49–53
- Gear AR. (1970). Inner- and outer-membrane enzymes of mitochondria during liver regeneration. Biochem J 120:577–87
- Grissa AK, Mornagui B, Aouani E, et al. (2007). Resveratrol, a red wine polyphenol, attenuates ethanol-induced oxidative stress in rat liver. Life Sci 80:1033–9
- Guan ZP, Lui CY, Morkin E, Bahl JJ. (2004). Oxidative stress and apoptosis in cardiomyocyte induced by high-dose alcohol. J Cardiovasc Pharmacol 44:696–702
- Hall PM, Lieber CS, De Carli LM, et al. (2001). Models of alcoholic liver disease in rodents: A critical evaluation. Alcohol Clin Exp Res 25:254–61
- Higuchi H, Adachi M, Miura S, et al. (2001). The mitochondrial permeability transition contributes to acute ethanol-induced apoptosis in rat hepatocytes. Hepatology 34:320–8
- Hwang HJ, Kim SW, Lim JM, et al. (2005). Hypoglycemic effect of crude exopolysaccharides produced by a medicinal mushroom Phellinus baumii in streptozotocin-induced diabetic rats. Life Sci 76:3069–80
- Ishii H, Adachi M, Fernández-Checa JC, et al. (2003). Role of apoptosis in alcoholic liver injury. Alcohol Clin Exp Res 27:1207–12
- Jaeschke H, Gores GJ, Cederbaum AI, et al. (2002). Mechanisms of hepatotoxicity. Toxicol Sci 65:166–76
- Kanbak G, Inal M, Baycu C. (2001). Ethanol-induced hepatotoxicity and protective effect of betaine. Cell Biochem Funct 19:281–5
- Kaplowitz N, Tsukamoto H. (1996). Oxidative stress and liver disease. Prog Liver Dis 14:131–59
- Kim SJ, Lee JW, Jung YS, et al. (2009). Ethanol-induced liver injury and changes in sulfur amino acid metabolomics in glutathione peroxidise and catalase double knockout mice. J Hepatol 50:1184–91
- Koteish A, Yang S, Lin H, et al. (2002). Chronic ethanol exposure potentiates lipopolysaccharide liver injury despite inhibiting Jun N-terminal kinase and caspase 3 activation. J Biol Chem 12:13037–44
- Layne E. (1957). Spectrophotometric and turbidimetric methods for measuring proteins. Meth Enzymol 10:447–55
- Lieber CS, De Carli LM, Sorrell MF. (1989). Experimental methods of ethanol administration. Hepatology 10:501–10
- Lieber CS. (2004). Alcoholic fatty liver. Alcohol 34:9–19
- Lindequist U, Niedermeyer TH, Julich WD. (2005). The pharmacological potential of mushrooms. Evid Based Complement Alternat Med 2:285–99
- Luan J, Zhou B, Ding H, Qi Z. (2007). Tolerance of mice to lipopolysaccharide is correlated with inhibition of caspase-3-mediated apoptosis in mouse liver cells. Acta Biochim Biophys Sin 39:96–100
- Olney JW, Tenkova T, Dikranian K, et al. (2002). Ethanol-induced apoptotic neurodegeneration in the developing mouse brain. Brain Res Dev Brain Res 133:115–26
- Ostrowskaa J, £uczaja W, Kasackab I, et al. (2004). Green tea protects against ethanol-induced lipid peroxidation in rat organs. Alcohol 32:25–32
- Pari L, Suresh A. (2008). Effect of grape (Vitis vinifera L.) leaf extract on alcohol induced oxidative stress in rats. Food Chem Toxicol 46:1627–34
- Ronis MJ, Hakkak R, Korourian S, et al. (2004). Alcoholic liver disease in rats fed ethanol as part of oral or intragastric low-carbohydrate liquid diets. Exp Biol Med 229:351–60
- Srirajaskanthan R, Preedy V. (2006). Diagnosis and management of alcoholic liver disease. Clin Effect Nurs 9S3:286–94
- Tabata, K, Ito W, Kojima T, et al. (1981). Ultrasonic degradation of Schizophyllan, an antitumor polysaccharide produced by Schizophyllum commune fries. Carbohydr Res 89:121–35
- Tsukagoshi SOF. (1974). Protein-bound polysaccharide preparation, PS-K, effective against mouse sarcoma1 80 and rat ascites hepatoma AH-13 by oral use. Gann 65:557–8
- Uzbay T, Kayir H, Celik T, Yuksel N. (2006). Acute and chronic tianeptine treatments attenuate ethanol withdrawal syndrome in rats. Prog Neuropsychopharmacol Biol Psychiatry 30:478–85
- Venkatraman A, Shiva S, Davis AJ, et al. (2003). Chronic alcohol consumption increases the sensitivity of rat liver mitochondrial respiration to inhibition by nitric oxide. Hepatology 38:141–7
- Wang YL, Hu R, Lugea A, et al. (2006). Ethanol feeding alters death signaling in the pancreas. Pancreas 32:351–9
- Wang H, Wu G, Park HJ, et al. (2012). Protective effect of Phellinus linteus polysaccharide extracts against thioacetamide-induced liver fibrosis in rats: A proteomics analysis. Chin Med 7:23
- Wasser SP. (2002). Medicinal mushrooms as a source of antitumor and immunomodulating polysaccharides. Appl Microbiol Biotechnol 60:258–74
- Wayhs CAY, Tortato C, Mescka CP, et al. (2013). The association effect of insulin and clonazepam on oxidative stress in liver of an experimental animal model of diabetes and depression. Pharm Biol 51:533–8
- Yamac M, Kanbak G, Zeytinoglu M, et al. (2008). Hypoglycemic effect of Lentinus strigosus (Schwein.) Fr. crude exopolysaccharide in streptozotocin-induced diabetic rats. J Med Food 11:513–17
- Yamac M, Zeytinoglu M, Kanbak G, et al. (2009). Hypoglycemic effect of crude exopolysaccharides produced by Cerrena unicolor, Coprinus comatus and Lenzites betulina isolates in streptozotocin-induced diabetic rats. Pharm Biol 47:168–74
- Yan M, Zhu P, Liu HM, et al. (2007). Ethanol induced mitochondria injury and permeability transition pore opening: Role of mitochondria in alcoholic liver disease. World J Gastroenterol 13:2352–6
- Yang BK, Ha JY, Jeong SC, et al. (2000). Production of exo-polymers by submerged mycelial culture of Cordyceps militaris and its hypolipidemic effect. J Microbiol Biotechnol 10:784–88
- Young C, Roth KA, Klocke BJ, et al. (2005). Role of caspase-3 in ethanol-induced developmental neurodegeneration. Neurobiol Dis 20:608–14
- Yuan G, Gong Z, Li J, Li X. (2007). Ginkgo biloba extract protects against alcohol-induced liver injury in rats. Phytother Res 21:234–8
- Zhou Z, Sun X, Kang YJ. (2001). Ethanol-induced apoptosis in mouse liver: Fas- and cytochrome c-mediated caspase-3 activation pathway. Am J Pathol 159:329–38
- Zovein A, Flowers-Ziegler J, Thamotharan S, et al. (2004). Postnatal hypoxic-ischemic brain injury alters mechanisms mediating neuronal glucose transport. Am J Physiol Regul Integr Comp Physiol 286:273–82