Abstract
Context: Kombucha (KT) is claimed to have various beneficial effects on human health, but there is very little scientific evidence available in the literature.
Objective: The present study investigates the effects of Camellia sinensis (GT) Linn. (Theaceae) and KT, two natural drinks, on cholesterol and antioxidant status using a hypercholesterolemia rat model.
Materials and methods: The present study compared the free-radical scavenging abilities and polyphenol levels of GT and KT. Wistar rats fed cholesterol-rich diets were given KT or GT (5 mL/kg body weight per day, po) for 16 weeks, then fasted overnight and sacrificed. The plasma lipid levels, thiobarbituric acid reactive substances (TBARS) and aspartate aminotransferase (AST), alanine aminotransferase (ALT), and γ-glutamyl transpeptidase (GGT) serum levels, antioxidant activities of superoxide dismutase (SOD) and catalase (CAT), and creatinine and urea rats were examined.
Results: KT had a phenolic compound of 955 ± 0.75 mg GAE/g) followed, by GT (788.92 ± 0.02 mg GAE/g). The free radical scavenging activity of KT was higher than GT. Compared with GT, KT induced lowered serum levels of TC, TG, VLDL-C, and LDL-C by 26, 27, 28, and 36%, respectively, and increased the serum level of high-density lipoprotein cholesterol (HDL-C). KT induced a 55% decrease of TBARS level in liver and 44% in kidney, compared with those of rats fed a cholesterol-rich diet alone. Moreover, CAT and SOD activities were reduced by 29 and 33%, respectively, in liver and 31 and 35%, respectively, in kidney, after oral administration of KT, compared with those of HCD-fed rats.
Conclusion: The findings revealed that KT administration induced attractive curative effects on hypercholesterolemic, particularly in terms of liver–kidney functions in rats. Its effect on humans needs to be studied further.
Introduction
Obesity is a serious health problem worldwide (De Onis et al., Citation2010; Halfon et al., Citation2013). The prevalence of obesity is rising dramatically among all ages with changes of lifestyles and dietary fat intake (Power & Schulkin, Citation2008). Complementary and alternative medicine applications have attracted special attention in recent research as they offer new promising opportunities for the development of efficient and lower cost alternatives to existing synthetic lipid-lowering agents. In recent years, researchers have paid particular attention to the biologically active ingredients in food and beverages, especially alkaloids and polyphenols due to their positive effects on human health. Tea, a product made up from leaves and buds of Camellia sinensis Linn. (Theaceae), is the second most consumed beverage in the world, well ahead of coffee, beer, wine, and carbonated soft drinks (Costa et al., Citation2002; Rietveld & Wiseman, Citation2003). Originating from India and China, tea is popular all over the world for its taste from the past 2000 years. The economic and social interest in tea is clear and its consumption is part of the daily routine of many, as an everyday drink and as a therapeutic aid in many illnesses. Green tea (GT) is also reported to reduce serum cholesterol levels and inhibit hypertension, mutagenesis, and tumorigenesis in vitro and in vivo (Hodgson et al., Citation1999; Muramatsu et al., Citation1986; Yang & Wang, Citation1995; Yokozawa et al., Citation1998, Citation2002). Several studies have shown that most of these antioxidant properties are correlated with the polyphenolic compounds present rather the ascorbic acid, tocopherol, or β-carotene (Lee et al., Citation2007).
The aging of the population and limitations of modern medicine have led many people to look for new ways to improve their health. When we study the development of civilization and the role of food and folk medicine, we often discover that many foods and beverages were used for their assumed beneficial effects on health. The predominant flavonoids in GT are catechins, namely epicatechin (EC), epicatechin-3-gallate (ECG), epigallocatechin (EGC), and epigallocatechin-3-gallate (EGCG) (Feng, Citation2006). These compounds account for up to 40% of the dry weight of GT (Balentine et al., Citation1997). From a pharmacological perspective, EGCG is currently receiving by far the most attention due to its antioxidant, anti-inflammatory, and anticarcinogenic effects (Shankar et al., Citation2007).
Tea and kombucha tea (KT), a fermented form of green tea, are presented in the literature as two very distinct beverages and no correlation has so far been reported between them (Ernst, Citation2003). Some of the effects reported for KT intakes are, however, very similar to those described for tea (Ernst, Citation2003). Nevertheless, while the composition, properties, and effects of tea on chronic and progressive illnesses, such as obesity and hyperlipidemia, are well-documented in the literature (Rahul et al., 2007), little data are currently available on these issues with regard to KT. In fact, most of the data on KT tea is anecdotal and further studies are needed to elucidate its putative therapeutic potential, particularly against hyperlipidemia.
Although the beneficial and/or adverse effects of KT on human health have not been scientifically determined yet, there are several reasons to believe that KT may have desirable positive effects on human health. In fact, the metabolic and health effects of several probiotic products are gathering increasing momentum in recent years. A number of currently commercialized food products (e.g., yogurt, cheese, fermented vegetables, and kefir) are known to contain live bacteria, or metabolites of bacteria, produced during fermentation processes, and are considered as health-promoting probiotic foodstuffs (Fuller, Citation1989; Perdigon et al., Citation2001). Moreover, several studies have recently demonstrated that KT can reduce cell damage induced by oxidative stress (Gharib, Citation2009; Greenwalt et al., Citation2000). KT has also been reported to constitute a potent therapeutic supplement that improved resistance against cancer, prevented cardiovascular diseases, promoted digestive functions, stimulated the immune system, and reduced inflammatory problems (Greenwalt et al., Citation2000; Murugesan et al., Citation2009).
The present study investigates and compares the effects of GT and KT, two natural drinks commonly consumed around the world, on the cholesterol metabolism and antioxidant status using a hypercholesterolemic rat model. For the sake of pertinence, the biological activities of KT were compared with those reported for GT (Yokozawa et al., Citation2002).
Materials and methods
GT preparation
GT was manufactured from Camellia sinensis by the Tunisian Office Trade. Briefly, 100 g of sugar were added to 1 L of distilled water, and the solution was boiled for 15 min in a sterile conical flask. GT powder was added to the flask at (12 g/L), which was then left to cool down at room temperature for 20 min. Then, the mixture was filtered using a sterile nylon mesh, and the filtrate was used as GT.
KT preparation
KT was prepared as previously reported by Jayabalan et al. (Citation2007). The KT cultures employed in the present work were purchased from a French commercial online outlet (Kombucha-Shop, Kombucha, London, UK). They are known as symbionts of yeasts (e.g. Zygosaccharomyces, Schizosaccharomyces, Torulospora, Rhodotorula, Brettanomyces, Candida, Pichia, and Zygosaccharomyces) and bacteria (e.g., Acetobacter) (Murugesan et al., Citation2009; Teoh et al., Citation2004). The cultures were stored at 4 °C prior to fermentation. GT was poured into 1 L glass jars that had been previously sterilized at 121 °C for 20 min.
The tea broth was inoculated with 2.5% (w/v) of freshly grown tea fungus that had been cultured in the same medium (Hesseltine, Citation1965). The fermentation, kept under aseptic conditions, was carried out by incubating the KT culture at 28 ± 1 °C for 12 d. The flask was covered with clean cheese cloth and fixed with rubber bands. The medium (brew) was then centrifuged aseptically at 15 000 × g for 30 min, the supernatant was filtered by using 0.45 µm millipore membrane filter and stored in polypropylene vials at −20 °C for further use (Kallel et al., Citation2012).
Measurement of free radical-scavenging action
2,2-Diphenyl picrylhydrazyl (DPPH) free radicals scavenging activity was assessed according to Blois (Citation1958), with a slight modification. Different concentrations of the GT and KT (10–50 µg/mL) was mixed with 1 mL of 0.1 mM DPPH in ethanol solution and 450 μL of 50 mM Tris-HCl buffer (pH 7.4) was added. The solution was incubated at 37 °C for 30 min and reduction of DPPH free radicals was measured by reading the absorbance at 517 nm. Control was maintained. This activity is given as % DPPH scavenging and calculated according to the following equation:
The antioxidant activities of GT and KT were expressed as IC50, defined as the concentration of the GT and KT required to cause a 50% decrease in initial DPPH concentration. Each sample was analyzed six times.
Scavenging of superoxide anion
The influence of GT and KT on the generation of superoxide anion was measured according to the method described in previously work (Yen & Chen, Citation1995). Superoxide anion was generated in a non-enzymic system and determined by a spectrophotometric measurement for reduction of nitro blue tetrazolium. The reaction mixture, which contained 1 mL of extract in distilled water, 1 mL of PMS (60 l M) in phosphate buffer (0.1 M, pH 7.4), 1 mL of NADH (468 l M) in phosphate buffer and 1 mL of NBT (150 l M) in phosphate buffer, was incubated at ambient temperature for 5 min, and the color was read at 560 nm against blank samples. All analyses were run in triplicate and mean values were calculated:
where blank OD is the absorbance of the control reaction and sample OD is the absorbance in the presence of GT and KT. The IC50 value was calculated from the plot of the inhibition percentage against the extract concentration. Each sample was analyzed six times.
Determination of total polyphenol content
The total phenolic content in GT and KT was determined with the Folin–Ciocalteau reagent using the method of Chen et al. (Citation2007). A standard curve must be first plotted using gallic acid as a standard. Different concentrations of gallic acid were prepared in methanol, and their absorbances were recorded at 750 nm. Diluted sample (100 µL) was added to 2 mL of 2% Na2CO3 aqueous solution. After 2 min, 100 µL of 50% Folin–Ciocalteau reagent was added. The final mixture was shaken and then incubated for 30 min in the dark at room temperature. The absorbance of all samples was measured at 750 nm, and the results are expressed in mg gallic acid equivalents per L (mg GAE/L). Each sample was analyzed six times.
In vivo studies
Animals and experimental design
Adult male Wistar rats, weighing 170 ± 10 g, were obtained from the Central Pharmacy (SIPHAT, Tunisia). They were housed at 22 ± 3 °C with light/dark periods of 12 h and a minimum relative humidity of 40%. The animals had free access to commercial pellet diet (SICO, Tunisia) and water ad libitum. The general guidelines for the use and care of living animals in scientific investigations were followed (Council of European Communities, Citation2010). The handling of the animals was approved by the Tunisian Ethical Committee for the Care and Use of Laboratory Animals.
The rats were randomly divided into six experimental groups (n = 8).
Group 1 was fed a standard laboratory diet [Cont] ().
Group 2 was fed a standard laboratory diet and GT [Cont + GT] by gastric gavage (5 mL per kg of body weight) every day.
Group 3 was fed a standard laboratory diet and KT [Cont + KT] by gastric gavage (5 mL per kg of body weight) every day.
Group 4 was fed a cholesterol-rich diet (HCD) (normal diet supplemented with 1% cholesterol and 0.25% bile salts) [HCD].
Group 5 received HCD and GT [HCD + GT] by gastric gavage (5 mL per kg of body weight) every day (Hamden et al., Citation2011).
Group 6 received HCD and KT [HCD + KT] by gastric gavage (5 mL per kg of body weight) every day (Hamden et al., Citation2011).
The experiment was conducted over a period of 16 weeks (Jemai et al., Citation2008). The body weight was measured every day. At the end of the experimental period, rats were sacrificed by decapitation in order to minimize the handling stress, and the trunk blood collected. The plasma was prepared by centrifugation (1500 × g, 15 min, 4 °C), frozen, and stored at −20 °C until analysis. The livers and kidneys were removed and rinsed with physiological saline solution. All samples were stored at −80 °C until analyzed.
Table 1. Composition of the control diet (g/kg).
Plasma lipids
Plasma was separated by centrifugation at 3500 rpm for 15 min at 4 °C. Plasma samples collected were used fresh and subjected immediately to sequential ultracentrifugation in a Beckman L7-55 ultracentrifuge (Beckman-Instruments, Fullerton, CA) with a Beckman SW50 rotor. Successive fraction was brought to the appropriate density with solid KBr (d = 1.063 kg/L for LDL) and centrifuged, respectively, at 45 000 rpm for 15 h and at 40 000 rpm for 24 h (Levy et al., 1990). Supernatants were recovered by tube slicing and, after appropriate dilution, triglycerides and cholesterol concentrations were determined by enzymatic methods with commercial kits (ELITECH Diagnostics, Sees, France).
The high-density lipoprotein cholesterol (HDL-C) fraction was determined in the rest of plasma. Plasma concentrations of triglycerides and cholesterol were measured by enzymatic methods with the same commercial kits. Very low-density lipoprotein cholesterol (VLDL-C) was calculated using the following formula: VLDL-C = triglycerides (TG)/5 (Friedwald et al., Citation1972). Atherogenic index was calculated for each animal in various groups using the following formula: low-density lipoprotein cholesterol (LDL-C) + VLDL-C/HDL-C (Dhandapani, Citation2007).
ABTS assay in the serum samples
The Trolox equivalent antioxidant capacity (TEAC) assay, measuring the reduction of the 2,2-azinobis-(3-ethyl benzothiazoline-6 sulfonic acid) (ABTS) radical cation by antioxidants, was derived from the method previously described (Katalinica et al., Citation2005) with minor modifications. Briefly, ABTS radical cation (ABTS•+) was produced by reacting ABTS stock solution with 2.45 mM potassium persulfate and allowing the mixture to stand in the dark at room temperature for 12–16 h before use. For the study, ABTS•+ solution was diluted with phosphate buffer saline pH 7.4 phosphate-buffered saline (PBS) to an absorbance of 0.70 (±0.02) at 734 nm. After addition of 2 mL of diluted ABTS•+ solution to 50 µL of serum, or Trolox standard, the reaction mixture was incubated for 6 min in a glass cuvette at 30 °C. The decrease in absorbance was recorded at 734 nm. All measurements were performed in triplicate. The free radical scavenging capacity of the biological sample, calculated as inhibition percentage of ABTS•+, was equated against a Trolox standard curve prepared with different concentrations (1.5–30 µmol/L). The results are expressed as µM of Trolox equivalents.
Biochemical estimations
Protein quantification
Protein contents in liver and kidney tissues were measured by the method of Lowry et al. (Citation1951) using bovine serum albumin as a standard.
Thiobarbituric acid reactive substances (TBARS) measurement
The liver and kidney tissues malondialdehyde concentrations, index of lipid peroxidation, were determined spectrophotometrically according to Draper and Hadley (Citation1990). Briefly, an aliquot of liver and kidney extracts supernatant was mixed with 1 mL of 5% trichloroacetic acid and centrifuged at 2500 × g for 10 min. An amount of 1 mL of thiobarbituric acid reagent (0.67%) was added to 500 μL of supernatant and heated at 90 °C for 15 min. The mixture was then cooled and measured for an absorbance at 532 nm using a spectrophotometer (Jenway UV-6305, Essex, England). The malondialdehyde values were calculated using 1,1,3,3-tetraethoxypropane as a standard and expressed as nmoles of malondialdehyde/mg of protein.
Antioxidant enzyme activities
Catalase (CAT) activity was measured according to Aebi (Citation1984). A total of 20 µL liver and kidney homogenate (about 1.5 mg proteins) were added to 1 mL phosphate buffer (0.1 M, pH 7) containing 100 mM H2O2. The rate of H2O2 decomposition was followed by measuring the decrease in the absorbance at 240 nm for 1 min. The enzyme activity was calculated using an extinction coefficient of 0.043 mM−1 cm−1 and expressed in international units (IU), i.e., in µmoles H2O2 destroyed/min/mg protein, at 25 °C.
Superoxide dismutase (SOD) activity was assayed by measuring its ability to inhibit the photoreduction of nitroblue terazolium (NBT) (Beyer & Fridovich, Citation1987). In this assay, one unit of SOD is defined as the amount required inhibiting the photo reduction of NBT by 50%. Riboflavin (0.26 mM final concentration) was added to start the reaction and the absorbance was recorded at 560 nm for 20 min. The activity was expressed as units/mg protein, at 25 °C.
Biochemical markers in plasma
Plasma levels of aspartate aminotransferase (AST), alanine aminotransferase (ALT), and γ-glutamyl transpeptidase (GGT) activities and creatinine and urea rates were measured in frozen aliquots of serum by standardized enzymatic procedures using commercial kits from (Biolabo, Maizy, France) on an automatic biochemistry analyzer (Vitalab Flexor E, Diamond Diagnostics, Holliston, MA)
Histopathological analysis
At the time of sacrifice, the liver and kidney tissues were removed and fixed in 10% formaldehyde solution. The washed tissues were dehydrated in increasing gradient of ethanol and finally cleared in toluene. The tissues were then embedded in molten paraffin wax. Sections were cut at 5 µm thickness and stained with hematoxylin and eosin. The slides were photographed with an Olympus UTU1X-2 camera connected to an Olympus CX41 microscope (Tokyo, Japan).
Statistical analysis
Statistical analysis was performed using the Statistical Package for the Social Sciences (SPSS, Version 10.0, SPSS Inc, Chicago, IL). Data are presented as mean ± SD. Determinations were obtained from eight animals per group, and the differences were examined using one-way analysis of variance (ANOVA) followed by the Fisher test (Stat View, SAS Inc, Cary, NC). Statistical significance was accepted at p < 0.05.
Results
Antioxidant capacities of GT and KT
The level of phenolic compounds in GT and KT is shown in . We noticed that KT has the highest amount of phenolic compounds (955 ± 0.75 mg GAE/g) followed by GT (788.92 ± 0.02 mg GAE/g).
Table 2. Amount of total phenolic compounds and free radical scavenging action of GT and KT.
The 50% scavenging concentration on DPPH radical (IC50) values of KT and GT was 38.78 ± 2.58 and 45 ± 1.23 µg/mL, respectively. Superoxide anion was also scavenged (). The 50% scavenging concentration NBT was calculated by linear regression analysis and was found, respectively, at 583 ± 0.52 and 655 ± 1.23 µg/mL for KT and GT.
Protective effects of GT and KT in vivo
Body and organ weights
The body weight increased in all groups throughout the treatment without any significant differences between them (data not shown). There was no difference in the kidney: body weight ratio after 16 weeks (). However, the liver:body weight ratio increased in rats fed a cholesterol-rich diet (HCD) compared with the rats fed a control diet (CD) (). In HCD groups of rats, the liver:body weight ratio reduced significantly after administration of GT and KT compared with those of HCD group (p < 0.05).
Figure 1. Effect of GT and KT on the liver (A) and kidney (B): body weight ratios. Cont, control diet; Cont + GT, control diet + green tea; Cont + KT, control diet + kombucha; HCD, high-cholesterol diet; HCD + GT, HCD + green tea; HCD + KT, HCD + kombucha. Data represent mean ± SD (n = 8 for each group). Values are statistically presented as follows: *p < 0.05 significant differences compared with controls. #p < 0.05 significant differences compared with cholesterol group (HCD) rats.
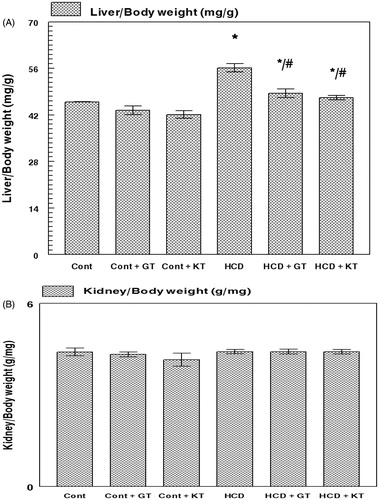
Plasma lipids
shows the plasma lipid levels at the end of the experiment. After 16 weeks of treatment, the total cholesterol TC, TG, LDL-C, and VLDL-C concentrations of rats fed a cholesterol-rich diet (HCD) showed a significant increase compared with the rats fed normal diet (CD) (). However, a decrease of HDL-C concentration of rats in the HCD group was observed (p < 0.05) (). Rats orally administrated with GT and KT had lower concentrations of TC, TG, LDL-C, and VLDL-C than rats receiving an HCD. GT and KT reduced the TC, TG, LDL-C, and VLDL-C levels by 16, 22, 25, and 25% and 26, 27, 36, and 28%, respectively. Moreover, the treatment of HCD fed groups with GT and KT significantly re-established their HDL-C level (p < 0.05). In fact, GT and KT reduced the AI by 42% and 53%, respectively ().
Figure 2. Effects of GT and KT on TC (A), TG (B), LDL-C (C), VLDL-C (D), HDL-C (E), and (AI) (F) levels in hypercholesterolemic rats. Cont, control diet; Cont + GT, control diet + green tea; Cont + KT, control diet + kombucha; HCD, high-cholesterol diet; HCD + GT, HCD + green tea; HCD + KT, HCD + kombucha. Data represent mean ± SD (n = 8 for each group). Values are statistically presented as follows: *p < 0.05 significant differences compared with controls. #p < 0.05 significant differences compared with cholesterol group (HCD) rats.
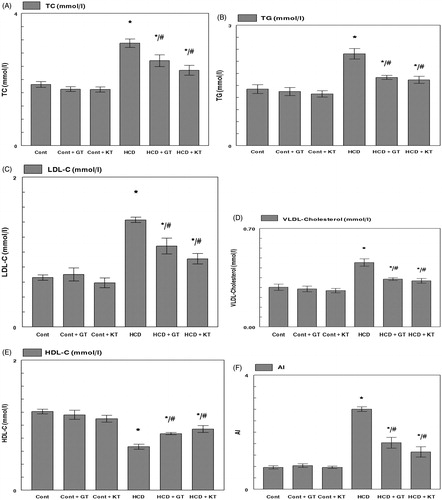
Serum antioxidant potential
ABTS radical cation scavenging ability () in serum of a cholesterol-rich diet fed rats was significantly low in comparison with rats fed normal diet (CD). Oral administration of GT and KT significantly re-established the impairment between both groups.
Figure 3. Effects of GT and KT on antioxidant activity in serum of a cholesterol-rich diet fed rats. Cont, control diet; Cont + GT, control diet + green tea; Cont + KT, control diet + kombucha; HCD, high-cholesterol diet; HCD + GT, HCD + green tea; HCD + KT, HCD + kombucha. Data represent mean ± SD (n = 8 for each group). Values are statistically presented as follows: *p < 0.05 significant differences compared with controls. #p < 0.05 significant differences compared with cholesterol group (HCD) rats.
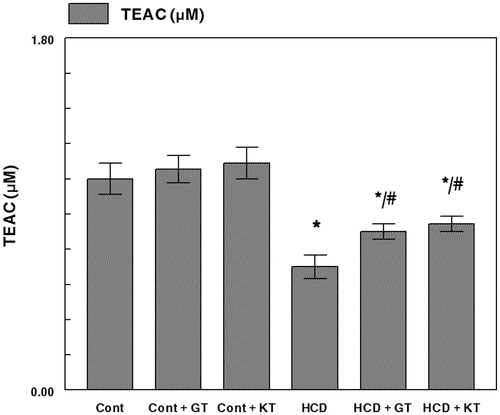
Lipid peroxidation
The TBARS levels were significantly increased (p < 0.05) in livers and kidneys within animals fed high-cholesterol diet compared with the control diet group. This increase was significantly reduced after treatment with GT and KT (). In fact, TBARS concentrations in liver and kidney were reduced by 32 and 55% and 33 and 44%, respectively, in groups 5 and 6.
Table 3. Antioxidant enzyme activity and lipid peroxidation concentration of different experimental groups.
Analysis of SOD and CAT activities in liver and kidney tissues
The activities of CAT and SOD were significantly increased (p < 0.05) in liver and kidney within animals fed high-cholesterol diet compared with the control diet group. This increase was significantly reduced after treatment with GT and KT (). In fact, CAT and SOD activities in liver and kidney were reduced by 34 and 36% and 29 and 36% in group 5 and 29 and 33% and 31 and 35% in group 6.
Liver-kidney dysfunction indices
In , we show that the activities of AST, ALT, and GGT in the plasma of fed high-cholesterol diet rats underwent significant increases of up to 67, 138, and 181%, respectively, when compared with control rats (p < 0.05). The administration of KT and GT was found to bring about marked decreases in terms of the three indices of liver toxicity. Moreover, and when compared with control rats, the hypercholesterolemic rats were noted to undergo significant (p < 0.05) increases of 83% and 56% in terms of the creatinine and urea rates in the plasma, respectively. Interestingly, the administration of KT and GT to hypercholesterolemic rats was observed to have reversed this increase back to normal. The findings from histological analyses further confirmed the positive effect of those two supplements on the liver and kidney.
Table 4. Liver profile indices (AST, ALT, and GGT) and kidney parameters (creatinine and urea) of control and experimental groups of rats.
Histopathological analysis
As shown in , the section of the liver from a control rat showed a normal architecture. However, liver of HCD rat exhibited fatty cysts apparition in liver tissues, whereas, examination of liver tissue of HCD-fed rats treated with GT and KT revealed potential protective action evidenced by reducing the development of fat cells in liver.
Figure 4. Histopathological studies of liver in control (A), high-cholesterol fed (B), green tea-supplemented rats (C), and kombucha-supplemented rats (D) (hematoxylin–eosin, H&E staining, 100×).
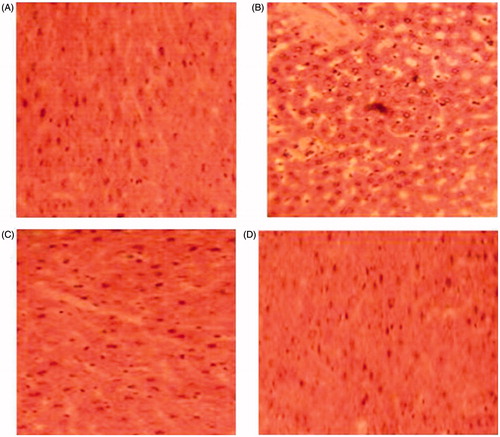
Moreover, as shown in , the section of the kidney from a control rat showed a normal architecture. However, kidney of HCD rat exhibited hypertrophied epithelial cells appeared in the renal tubules, whereas, examination of kidney tissue of HCD-fed rats treated with GT and KT revealed potential protective action.
Discussion
Although KT is popular around the world as a beneficial medicinal health-promoting drink, its beneficial and/or adverse effects on human health have not been scientifically determined yet. No previous study has, for instance, so far reported on the systematic investigation and evaluation of the hypocholesterolemic activity of KT. To the authors’ knowledge, the present work is the first attempt to investigate the protective effects of KT on hypercholesterolemic and the functions of the liver and kidney. The burden of oxidative stress is known to play a central role in the pathogenesis and progression of hypercholesterolemic complications. Hence it is likely that antioxidants might be effective in ameliorating the oxidative stress-mediated complications in hypercholesterolemic pathophysiology.
GT is well known for its antioxidant and free radical scavenging properties. On one hand, recent study has demonstrated that the antioxidant property of GT is mainly attributed to its polyphenolic content (Nooman et al., 2008). On the other hand, the results of the present study suggest that KT contains more polyphenols than GT itself (). The concentration of total phenolic compounds increases progressively up to day 12 probably because the enzymes liberated by the bacteria and yeast during fermentation degrade the complex polyphenols to small molecules and that in turn results in the increase of total phenolic compounds are potent antioxidants (Bhattcharya et al., 2011). Moreover, the free radical scavenging activities of KT are found to be higher than GT, which is in good agreement with other studies (Duenas et al., Citation2007). These results encouraged us to investigate the possible protective effect of KT in comparison with GT against hypercholesterolemic-induced and its complications in different organs including liver and kidney in rat model.
In the present study, rat body weight gains were not significantly different among the groups investigated. This observation is in accord with the study of Ander et al. (Citation2001) who showed that the body weight of hypercholesterolemic rats was not significantly different from that of control animals. Data related with organs weight showed no significant difference among all groups except the liver:body weight ratio which significantly increased in high-cholesterol fed rats. This could be related to an accumulation of lipids such as cholesterol and triglycerides in the liver (Fungwe et al., Citation1994).
The rats in the HCD group, which received a high-cholesterol diet, showed elevated serum total cholesterol, LDL-C than had those of the control group, suggesting that effective induction of hypercholesterolemia by supplementation of cholesterol in the diet was effectively established. The elevations in serum of total TC and TG levels observed on HCD group are in agreement with those reported by Jeon et al. (Citation2007) and Tauseef et al. (Citation2007). The high levels of LDL-C found in HCD rats may be attributed to a down-regulation in LDL receptors by cholesterol included in the diet (Mustad et al., Citation1997). After GT or KT treatment, the levels of TC, TG, and LDL-C were significantly decreased which indicate that both the beverages might be beneficial to hypercholesterolemic rats. The HDL-C concentration was significantly increased only in HCD-fed rats treated with GT or KT. A higher content of HDL-C is very important in humans because it is correlated with a reduced risk of coronary heart disease (Young et al., Citation2004). The increased HDL facilitates the transport of cholesterol from the serum to the liver, where it is catabolized and excreted from the body.
Lowering levels of TC and LDL-C and improving level of HDL-C have been linked to a lower risk of cardiovascular disease (CVD) (Libby et al., 2000). In fact, it was reported that the decrease in LDL-C concentration and the increase of HDL-C level could fasten the removal of cholesterol from peripheral tissues to liver for catabolism and excretion (Young et al., Citation2004). Also, high HDL-C levels may compete with LDL receptor sites on arterial smooth muscle cells and thus inhibit the uptake of LDL (Young et al., Citation2004). In addition, the increase in HDL-C concentration could protect the LDL against oxidation in vivo because lipids in HDL are preferentially oxidized before those in LDL (Young et al., Citation2004). Therefore, the results of the present study indicate that KT may reduce the incidence of CVD. These data are in agreement with the study of Yang and Wang (Citation1995) who reported that polyphenols from KT decrease plasma LDL-C levels and prevent their oxidation in vivo. The mechanism of this hypocholesterolemic action may be due to the inhibition of dietary cholesterol absorption in the intestine or its production by liver (Bursill & Roach, Citation2006) or stimulation of the biliary secretion of cholesterol and cholesterol excretion in the feces (Krzeminski et al., Citation2003). In contrast, the AI, defined as the ratio of LDL-C + VLDL-C and HDL-C, is believed to be an important risk factor of atherosclerosis. Our data clearly demonstrate that GT or KT significantly decreased the ratio. It has been shown that high serum levels of LDL-C and low serum levels of HDL-C are associated with an increased atherosclerosis risk (Korhonen et al., Citation1996). Increasing the HDL-C concentrations and decreasing the LDL-C concentrations in HCD-fed rats indicates the anti-atherogenic property of the KT. Nowadays, it is established that oxidative stress is one of the causative factors that link hypercholesterolemia with atherogenesis (Young & Mc Eneny, Citation2001). Hypercholesterolemia leads to increased cholesterol accumulation in cells thereby activating the production of oxygen-free radicals. Thus, cells are extremely vulnerable to these oxidative challenges and hypercholesterolemia (Kay, Citation1991).
In the present study, a significant decrease in the serum antioxidant potential was observed in rats fed with cholesterol compared with the control group. The increase of the serum antioxidant potential observed in KT-supplemented rats may be due to the removal toxic reactive species resulting from the high-cholesterol feeding. Moreover, we have observed increased activities of the antioxidant enzymes SOD and CAT in liver and kidney of rats fed a high-cholesterol diet as compared with those on a normal diet. Our results are in agreement with reports of other workers (Belguith-Hadriche et al., Citation2010; Jung et al., Citation2003). This increase in SOD and CAT activities is seen as an adaptation process, to face the free radical production. The neutralization of the oxidative burst with GT and KT probably eliminated the need of SOD and CAT participation to scavenge the free radicals (Belguith-Hadriche et al., Citation2010).
Several studies have revealed increased lipid peroxidation in clinical and experimental hypercholesterolemia. It has been established that hypercholesterolemia leads to increased production of oxygen free radicals (Prasad & Kalra, Citation1993), which exert their cytotoxic effect by causing lipid peroxidation, resulting in the formation of TBARS. In our study, hypercholesterolemic rats showed significant rise in liver and kidney TBARS levels. GT or KT treatment, along with a cholesterol diet, showed significant reduction of TBARS in all analyzed tissues. Both KT and GT, due to their excellent radical scavenging capacity, could suppress the oxidative damages and bring these parameters to near normalcy.
These data suggest that rats treated with GT or KT are less susceptible to peroxidative damage under the challenge of an oxidative stress, such as a high-cholesterol diet. These findings are, in fact, in good agreement with the results previously reported by Murugesan et al. (Citation2009) showing that KT has the potential to revert the CCl4-induced hepatotoxicity back through the production of antioxidant molecules during fermentation. Furthermore, Gharib (Citation2009) showed that, owing to its antioxidant potential, KT can ameliorate trichloroethylene-induced kidney damage by preventing lipid peroxidation and ROS species formation (Gharib, Citation2009).
It has been reported that oxidative stress is one of the causative factors that link hypercholesterolemia with the pathogenesis of atherosclerosis (Young & Mc Eneny, Citation2001). This stress results from an unbalance between the production of free radicals and the effectiveness of the antioxidant defense system (Halliwell, Citation1994). Oxidative environments might cause the damage of cells and tissues in the liver and kidney (Takeda et al., Citation2011), which is observed in the increased levels of AST, ALT, and GGT activities (indices of liver dysfunction) and of urea and creatinine (indices of kidney dysfunction). As far as the present study is concerned, the findings showed that GT or KT proved remarkably efficient in the decrease of the liver and kidney dysfunction indices in surviving hypercholesterolemic rats, namely the AST, ALT, and GGT activities and the urea and creatinine levels. The nephroprotective effects KT were also attributed to organic acids (e.g., acetic and glucuronic acids) which are known to facilitate the detoxification process through conjugation with toxins, which they then solubilize and eliminate from the body (Andlauer et al., Citation2000). This supplement could, therefore, be considered as a potential strong candidate for future industrial application as a therapeutic agent against liver and kidney toxicities. It is worth noting that the findings indicated that the curative effects achieved with the administration of KT were more pronounced than those reported for GT, which could presumably be attributed to the large amounts of polyphenols present in KT as compared with GT (Pratt & Hudson, Citation1990). In fact, further studies on the mechanisms and modes of action of KT are needed to fully appreciate its values and limitations.
Conclusion
Overall, we would like to mention that, with the increase in the popularity of tea and fermented tea, the present findings about the beneficial effects of KT and GT provide valuable information in the field of human healthcare research. The present study demonstrated that KT exhibited a pronounced hypocholesterolemic effect, reduced the lipid peroxidation process, and enhanced the antioxidant defense system in the experimental atherogenic model. These effects highlighted KT as a source of antioxidants able to reduce the frequency of cardiovascular diseases. The beneficial effect of dietary KT is presumably attributed to its potent hypocholesterolemic property, as well as antioxidant potential. Further studies are obviously needed to capitalize on the protective effects of KT in humans and to make its use suitable as an effective functional food with therapeutic potential.
Declaration of interest
The authors report that they have no conflict of interests. This research was supported by the Tunisian Ministry of Higher Education and Scientific Research and the Tunisian Ministry of Public Health.
References
- Aebi H. (1984). Catalase in vitro. Meth Enzymol 105:121–6
- Ander BP, Weber AR, Rampersad P, et al. (2001). Prevention of ischaemia-induced ventricular fibrillation in hypercholesterolemic rabbits consuming a flaxseed enriched diet. Br J Nutr 163:425–33
- Andlauer W, Kolb J, Furst P. (2000). A novel efficient method to identify betaglucuronidase activity in rat small intestine. J Parenter Enteral Nutr 24:308–10
- Balentine DA, Wiseman SA, Bouwens LC. (1997). The chemistry of tea flavonoids. Crit Rev Food Sci Nutr 37:693–704
- Belguith-Hadriche O, Bouaziz M, Jamoussi K, et al. (2010). Lipid-lowering and antioxidant effects of ethyl acetate extract of fenugreek seeds in high-cholesterol-fed rats. J Agr Food Chem 58:2116–22
- Beyer Jr WF, Fridovich I. (1987). Assaying for superoxide dismutase activity: Some large consequences of minor changes in conditions. Anal Biochem 161:559–66
- Bhattacharya S, Ahmed KKM, Chakraborty S. (2011). Free radicals cardiovascular diseases: An update. Free Radic Antioxidant 1:7–22
- Blois MS. (1958). Antioxidant determinations by the use of stable free radical. Nature 26:199–200
- Bursill CA, Roach PD. (2006). Modulation of cholesterol metabolism by the green tea polyphenol – epigallocatechin gallate in cultured human liver (HepG2) cells. J Agr Food Chem 54:1621–6
- Chen HY, Lin YC, Hsieh CL. (2007). Evaluation of antioxidant activity of aqueous extract of some selected nutraceutical herbs. Food Chem 104:1418–24
- Costa LM, Gouveia ST, Nobrega JA. (2002). Comparison of heating extraction procedures for Al, Ca, Mg and Mn in tea samples. Ann Sci 18:313–18
- Council of European Communities. (2010). Council instructions about the protection of living animals used in scientific investigations. Off J Euro Commun (JO 10/63/CEE) L276:1–33
- De Onis M, Blossner M, Borghi E. (2010). Global prevalence and trends of overweight and obesity among preschool children. Am J Clin Nutr 92:1257–64
- Dhandapani R. (2007). Hypolipidemic activity of Eclipta prostrata (L) leaf extract atherogenic diet induced hyperlipidemic rats. Indian J Expt Biol 45:617–19
- Draper HH, Hadley M. (1990). Malondialdehyde determination as index of lipid peroxidation. Methods Enzymol 186:421–31
- Duenas M, Hernandez T, Estrella I. (2007). Changes in content of bioactive polyphenolic compounds of lentils by the action of exogenous enzymes. Effect on their antioxidant activity. Food Chem 101:90–7
- Ernst E. (2003). Kombucha: A systematic review of the clinical evidence. Forsch Komplementarmed Klass Naturheilkd 10:85–7
- Feng WY. (2006). Metabolism of green tea catechins: An overview. Curr Drug Metab 7:755–809
- Friedwald WT, Levy RI, Fredickson DS. (1972). Estimation of the concentration of low density lipoprotein cholesterol in plasma, without use of the preparative centrifuge. Clin Chem 18:499–502
- Fuller R. (1989). Probiotics in man and animals. J Appl Bacteriol 66:365–78
- Fungwe TV, Fox JE, Cagen LM, et al. (1994). Stimulation of fatty acid biosynthesis by dietary cholesterol and of cholesterol synthesis by dietary fatty acid. J Lipid Res 35:311–18
- Gharib OA. (2009). Effects of Kombucha on oxidative stress induced nephrotoxicity in rats. Clin Med 4:23 (1–6)
- Greenwalt CJ, Steinkraus KH, Ledford RA. (2000). Kombucha, the fermented tea: Microbiology, composition, and claimed health effects. J Food Prot 63:976–81
- Halfon N, Kandyce L, Slusser W. (2013). Associations between obesity and comorbid mental health, developmental and physical health conditions in a nationally representative sample of US children aged 10 to 17. Acad Pediatr 13:6–13
- Halliwell, B. (1994). Free radicals, antioxidants and human diseases: Curiosity, cause or consequence. Lancet 344:721–24
- Hamden K, Jaouadi B, Carreau S, et al. (2011). Therapeutic effects of soy isoflavones on alpha-amylase activity, insulin deficiency, liver–kidney function and metabolic disorders in diabetic rats. Nat Prod Res 25:244–55
- Hesseltine CW. (1965). A millennium of fungi, food and fermentation. Mycologia 57:149–97
- Hodgson JM, Puddey IB, Burke V, et al. (1999). Effects on blood pressure of drinking green and black tea. J Hypertens 17:457–63
- Jayabalan R, Marimuthu S, Swaminathan K. (2007). Changes in content of organic acids and tea polyphenols during kombucha tea fermentation. Food Chem 102:392–8
- Jemai H, Feki I, Fki I, et al. (2008). Lipid lowering and antioxidant effects of hydroxytyrosol and its triacetylated derivative recovered from olive tree leaves in cholesterol-fed rats. J Agr Food Chem 56:2630–6
- Jeon SM, Kim HK, Kim HJ, et al. (2007). Hypocholesterolemic and antioxidative effects of naringenin and its two metabolites in high-cholesterol fed rats. Transl Res 149:15–21
- Jung UJ, Kim HJ, Lee JS, et al. (2003). Naringin supplementation lowers plasma lipids and enhances erythrocyte antioxidant enzyme activities in hypercholesterolemic subjects. Clin Nutr 22:561–8
- Kallel L, Desseaux V, Hamdi M, et al. (2012). Insights into the fermentation biochemistry of Kombucha teas and potential impacts of Kombucha drinking on starch digestion. Food Res Int 49:226–32
- Katalinica V, Modun D, Music I, Boban M. (2005). Gender differences in antioxidant capacity of rat tissues determined by 2,2′-azinobis (3-ethylbenzothiazoline 6-sulfonate; ABTS) and ferric reducing antioxidant power (FRAP) assays. Comp Biochem Phys C 140:47–52
- Kay NMR. (1991). Drosophila to bacteriophage to erythrocyte; the erythrocyte as a model for molecular and membrane aging of terminally differentiated cells. Gerontology 37:5–32
- Korhonen T, Savolainen MJ, Koistinen MJ, et al. (1996). Association of lipoprotein cholesterol and triglycerides with the severity of coronary artery disease in men and women. Atherosclerosis 127:213–20
- Krzeminski R, Gorinstein S, Leontowicz H, et al. (2003). Effect of different olive oils on bile excretion in rats fed cholesterol-containing and cholesterol-free diets. J Agr Food Chem 51:5774–9
- Lee YL, Yen MT, Mau JL. (2007). Antioxidant properties of various extracts from Hypsizigus marmoreus. Food Chem 104:1–9
- Levy E, Thibault L, Garofalo C, et al. (1990). Combined (n-3 and n-6) essential fatty acid deficiency is a potent modulator of plasma lipids, lipoprotein composition and lipolytic enzymes. J Lipid Res 31:2009–17
- Libby P, Aikawa M, Schonbeck U. (2000). Cholesterol and atherosclerosis. Biochim Biophys Acta 1529:299–309
- Lowry OH, Rosebrough NJ, Farr AL, Randall RJ. (1951). Protein measurement with Folin phenol reagent. J Biol Chem 193:265–75
- Muramatsu K, Fukuyo M, Hara Y. (1986). Effect of green tea catechins on plasma cholesterol level in cholesterol level in cholesterol-fed rats. J Nutr Sci Vitaminol 32:613–22
- Murugesan GS, Sathishkumar M, Jayabalan R, et al. (2009). Hepatoprotective and curative properties of Kombucha tea against carbon tetrachloride-induced toxicity. J Microbiol Biotechnol 19:397–402
- Mustad VA, Etherton TD, Cooper AD, et al. (1997). Reducing saturated fat intake is associated with increased levels of LDL receptors on mononuclear cells in healthy men and women. J Lipid Res 38:459–68
- Nooman AK, Ashok KS, Atif A, et al. (2008). Antioxidant activity of some common plants. Turk J Biol 32:51–5
- Perdigon G, Fuller R, Raya R. (2001). Lactic acid bacteria and their effect on the immune system. Curr Issues Intest Microbiol 2:27–42
- Power ML, Schulkin J. (2008). Sex differences in fat storage, fat metabolism, and the health risks from obesity: Possible evolutionary origins. Br J Nutr 995:931–40
- Prasad K, Kalra J. (1993). Oxygen free radicals and hypercholesterolemic atherosclerosis. Am Heart J 125:958–71
- Pratt DE, Hudson BJF. (1990). Natural antioxidants not explored commercially. In: Hudson BJF, ed. Food Antioxidants. London (UK): Elsevier Applied Science, 171–92
- Rahul B, Birari RB, Bhutani KK. (2007). Pancreatic lipase inhibitors from natural sources unexplored potential. Drug Discov Today 12:879–89
- Rietveld A, Wiseman S. (2003). Antioxidant effects of tea: Evidence from human clinical trials. J Nutr 133:3275–84
- Shankar S, Ganapathy S, Srivastava RK. (2007). Green tea polyphenols: Biology and therapeutic implications in cancer. Front Biosci 12:4881–99
- Takeda S, Sato N, Rakugi H, Morishita R. (2011). Molecular mechanisms linking diabetes mellitus and Alzheimer disease: Beta-amyloid peptide, insulin signaling, and neuronal function. Mol Biosyst 7:1822–27
- Tauseef M, Sharma KK, Fahim M. (2007). Aspirin restores normal baroreflex function in hypercholesterolemic rats by its antioxidative action. Eur J Pharmacol 56:136–43
- Teoh AL, Heard G, Cox J. (2004). Yeast ecology of kombucha fermentation. Int J Food Microbiol 95:119–26
- Yang CS, Wang ZY. (1995). Tea and cancer. J Natl Cancer Inst 85:1038–49
- Yen GC, Chen HY. (1995). Antioxidant activity of various tea extracts in relation to their antimutagenicity. J Agr Food Chem 43:27–32
- Yokozawa T, Dong E, Nakagawa T, et al. (1998). Effects of Japanese black tea on atherosclerotic disorders. Biosci Biotechnol Biochem 62:44–8
- Yokozawa T, Nakagawa T, Kitani K. (2002). Antioxidative activity of green tea polyphenol in cholesterol-fed rats. J Agr Food Chem 50:3549–52
- Young CE, Karas RH, Kuvin JT. (2004). High-density lipoprotein cholesterol and coronary heart disease. Cardiol Rev 12:107–19
- Young IS, Mc Eneny J. (2001). Lipoprotein oxidation and atherosclerosis. Biochem Soc Trans 29:358–62