Abstract
Context: Cisplatin is a highly effective chemotherapeutic agent against many tumors; however, it has potent adverse effects. Zengmian Yiliu granule (ZMYL), a traditional Chinese medicine (TCM) compound, has been clinically used against platinum (Pt)-induced toxicity and to enhance the efficacy of cisplatin.
Objective: The study was conducted to investigate the likelihood of potential pharmacokinetics drug–herbs interaction (DHI) between cisplatin and ZMYL.
Materials and methods: An improved ICP-MS method combined with ultrafiltration and microwave-assisted digestion was performed to determine the total and free Pt concentrations in rat plasma after intraperitoneal administration of cisplatin (9 mg/kg) or a combined administration with ZMYL (1 g/kg) by gavage.
Results: ZMYL produced a potential DHI on the pharmacokinetic parameters of cisplatin, calculated from the total Pt concentration. The clearance rate decreased from 110.52 to 66.12 mLh−1 kg−1, the mean residence time extended from 63.1 to 164.54 h, the area under the plasma concentration–time curve increased from 86.58 to 152.93 µg h mL−1, the elimination half-life extended from 48.38 to 126.4 h, and the elimination rate constant decreased from 0.017 to 0.006 h, in the ZMYL combination group (p < 0.05). In terms of free Pt concentration, the apparent volume of distribution and clearance rate was statistically different (p < 0.05). The Pt plasma protein binding ratios in the early dose stages were significantly boosted by the co-administration of ZMYL (p < 0.01).
Discussion and conclusion: ZMYL is a potential complementary and alternative medicine for cisplatin chemotherapy. The therapeutic benefits of ZMYL–cisplatin chemotherapy derived from pharmacokinetic interaction needs further investigation.
Introduction
Cisplatin (cis-diaminedichloroplatinum, CDDP) is a classical inorganic coordination compound, which was first discovered for its anticancer effect in 1965 (Rosenberg, Citation1985). As a first-generation platinum (Pt) drug, CDDP is one of the most effective chemotherapeutics and is widely used in chemotherapy for the treatment of a variety of different malignancies (Park et al., Citation2009). CDDP DNA-binding is known as the basic molecular mechanism inhibiting DNA synthesis through the formation of DNA adducts and cross-links. CDDP is normally highly bound (>80%) to plasma protein and more readily reacts with DNA in its hydrolyzed form when a water molecule displaces a chloride ion (Alderden et al., Citation2006). The unbound fraction of a drug is responsible for its pharmacological activity and overall disposition. Small changes in the unbound fraction of a highly bound drug could result in a disproportionately large increase in drug action. Ignorance of the pharmacological implications of the free drug fraction may contribute to an incidence of unexpected adverse reactions (Mills et al., Citation1997); hence, the quantitative investigation of drug-binding is essential in pharmacokinetic study (Wang et al., Citation2003).
CDDP belongs to the group of cell-cycle nonspecific drugs, which can cause different side effects, such as nephrotoxicity, neurotoxicity, ototoxicity, gastrointestinal tract toxic reaction, and others (Minami, Citation1995). These toxic reactions have severely restrained the use of CDDP and have influenced the life quality of cancer patients. Nowadays, an increasing amount of CDDP is being used in combination with other drugs; consequently, complementary and alternative medicine, which could relieve the toxic and side-effect of chemotherapy, improve patient ability to endure chemotherapy. Several attempts have therefore been made to further develop complementary and alternative medicine with the aim of preserving the high therapeutic activity of CDDP by reducing unwanted side-effects. Sunitinib has been reported to potentially improve the chemotherapeutic efficacy and ameliorate CDDP-induced nephrotoxicity in experimental animals (Suddek, Citation2010), whereas intratympanic dexamethasone and vitamin E have protective effects on CDDP-induced ototoxicity (Paksoy et al., 2011). The combination of CDDP and gemcitabine might be a promising new strategy for the treatment of neuroblastoma (Besançon et al., Citation2012). Moreover, the triple combination of paclitaxel, CDDP, and 5-FU is effective in metastatic and/or recurrent nasopharyngeal carcinoma. CDDP, in combination with other agents, including docetaxel or capecitabine, had also been reported (Chen et al., Citation2012).
The synergistic effect of traditional Chinese medicine (TCM) in chemotherapy is the hotspot for combined cancer therapy. In clinical practice, TCM adjuvant chemotherapy has been proven to reduce the incidence rate of toxicity during chemotherapy. Resveratrol, a polyphenol found in numerous plant species, works against CDDP-induced genotoxicity and apoptosis (Attia, Citation2011). The protective effect of ellagic acid ameliorating CDDP-induced testicular toxicity has been reported (Türk et al., Citation2008). Polyphenol in an appropriate dose of grape-seed (Vitis spp.) extract has a therapeutic value in treating CDDP-induced emesis (Wang et al., Citation2005). CDDP combined with compound recipes of TCM also had satisfactory action in synergy and attenuation. Dietary Amrit Nectar tablets (MA-7, containing 38 herbs) may protect against CDDP-induced changes in glutathione (GSH) and glutathione-S-transferase (GST) activity in rat liver and kidney (Dwivedi et al., Citation2005). A compound recipe made of Cinnamomum cassia Presl (Lauraceae), Curcuma phaeocaulis Val. (Zingiberaceae), Psoralea corylifolia L. (Fabaceae), and Rheum officinale Baill (Polygonaceae) could strengthen the anticancer activity of CDDP by preventing calcium influx and restraining the drug resistance gene expression of lung cancer (Cao et al., Citation2008). The Shengjiang Xiexin Decoction, composed of Zingiber officinale Rosc. (Zingiberaceae), Glycyrrhiza uralensis Fisch. (Fabaceae), Panax ginseng C. A. Mey. (Araliaceae), and so on, could confer protection on the brain tissue and gastrointestinal epithelium of mice, as influenced by CDDP (Xian & Wang, Citation2010).
Zengmian Yiliu granules (ZMYL), a compound composed of Astragalus membranaceus (Fisch.) Bge. var. mongholicus (Bge.) Hsiao (Fabaceae), Codonopsis pilosula (Franch.) Nannf (Campanulaceae), Rehmannia glutinosa Libosch. (Scrophulariaceae), Scutellaria barbata D. Don (Labiatae), Atractylodes macrocephala Koidz (Compositae), and others, has been proven by long-term clinical application and more than 20 years of verification for its curative effect in ovarian cancer (Huang et al., Citation2004; Zhang et al., Citation2012). Previous reports have revealed that ZMYL could increase white blood cells in peripheral blood, increase DNA content of bone marrow and spleen index destroyed by CDDP, regulate immune function, and improve bone marrow suppression in tumor-bearing mice (Zhang et al., Citation2011). Evidence has shown that ZMYL could inhibit neogenesis of tumor vessels (HU et al., Citation2012). Furthermore, ZMYL could reverse CDDP-resistance of resistant ovarian cancer in nude mice and enhance the tumor inhibitory effect (Li et al., Citation2012; Qi et al., Citation2012). In addition, several components in ZMYL, such as paeoniflorin, albiflorin, and wogonin, have been reported to have antitumor activity or to enhance the efficacy of CDDP radiation and chemotherapy as well as oppose the toxicity of chemotherapy-induced myelosuppression (Chen et al., Citation2008; Xu et al., Citation2011; Yu et al., Citation2007). These results indicate that ZMYL is a potential complementary and alternative medicine during CDDP chemotherapy. The efficacy of ZMYL has already been documented; nevertheless, concern regarding its perceived mechanism, particularly with respect to knowledge on drug–herbs interaction (DHI) potential and its clinical significance, persists. Hence, the likelihood of potential pharmacokinetic DHI between CDDP and ZMYL is suspected and was investigated in this paper.
Reported analytical methods for the determination of CDDP in biological specimens include atomic absorption spectrometry (AAS) (Nygren & Lundgren, Citation1997), electrospray ionization mass spectrometry (ESI-MS) (Minakata et al., Citation2006), inductively coupled plasma-atomic emission spectroscopy (ICP-AES), capillary gas chromatography (GC) (Laghari et al., Citation2008), and inductively coupled plasma-mass spectrometry (ICP-MS) (Yamada et al., Citation2005). Among these methods, ICP-MS is effective in detecting trace elements in biologic materials, multi-elements analysis of human tissue, and metabolic studies of minerals in humans (Hanada et al., Citation1998). For the pharmacokinetic study, the total and free CDDP concentration in plasma was determined via an improved ICP-MS method combined with ultrafiltration and microwave-assisted digestion.
Materials and methods
Drugs and reagents
CDDP was purchased as a powder injection (QiLu Company, ShanDong, China). ZMYL was provided by the Shanghai Shuguang Hospital affiliated with the Shanghai University of Traditional Chinese Medicine. The platinum standard was purchased from NSI Solutions Incorporated (1000 ppm, NSI, Raleigh, NC). The internal standard solution (100 ppm, Li, Sc, Ge, Y, In, Tb, Lu, Ir, Bi, Agilent, Tokyo, Japan) and tuning solution (1 ppb, Ce, Co, Li, Mg, Tl, Y. Agilent, Tokyo, Japan) for ICP-MS were purchased from Agilent Technologies (Santa Clara, CA). Nitric acid (HNO3) was obtained from Merck (65%, Suprapur® grade, Merck, Darmstadt, Germany). The other chemicals used were of HPLC grade. All dilutions were made using high purity deionized water (18.2 MΩ) obtained from a Milli-Q water purification system (Millipore, Billerica, MA).
Animals
Male and female Sprague–Dawley rats weighing 240–300 g were provided by the Experimental Animal Centre of Shanghai University of Traditional Chinese Medicine, China, with certificate SYXK (Shanghai) 2009-0069. All animal-use procedures were in accordance with the regulations of the experimental animal administration issued by the State Committee of Science and Technology of the People’s Republic of China on 14 November 1988 and approved by the Ethical Committee of Shanghai University of Traditional Chinese Medicine (approval No: SUTCM-2011-1010, approved on 10 October 2011). The animals were housed in different cages (five animals per cage) and acclimated in the laboratory for at least 1 week prior to testing. Animals were housed in a well-lit air-conditioned room at 21 °C with 60% relative humidity at standard environmental conditions (12 h light and 12 h dark cycle) and had free access to rodent chow and tap water prior to the study. Before the experiments, the animals were fasted for 12 h with free access to water prior to administration.
Instrumentation
The ICP-quadrupole-MS of Agilent 7500CX (Agilent Technologies, Santa Clara, CA) equipped with a peristaltic pump, a nebulizer, a spray chamber, and a quartz torch was used in Pt analyses. Instrumentation of MARS X for microwave digestion (CEM, New York, NY) with a digestion jar of XPRESS TFM (10 mL) was used in the pretreatment of the plasma sample. Ultrafiltration tube of Amicon® Ultra (10 KD) (Millipore, Billerica, MA) was applied to acquire free Pt plasma.
Preparation of ZMYL
ZMYL was prepared using A. membranaceus (18 g), which was extracted via circumfluence extraction with 60% ethanol for 1.5 h, and was then filtered. This procedure was repeated three-times, and the filtrates were ultimately combined. The residue, which was left after ethanol was evaporated, was incorporated with 10 other Chinese crude drugs, namely, C. pilosula (15 g), Paeonia lactiflora Pall. (Paeoniaceae) (15 g), R. glutinosa (12 g), S. barbata (12 g), Akebia quinata (Thunb.) Decne (Lardizabalaceae) (12 g), A. macrocephala (9 g), Lycium barbarum L. (Solanaceae) (9 g), Asparagus cochinchinensis (Lour.) Merr (Liliaceae) (9 g), Cornu cervi degelatinatum (Cervidae) (9 g), and Aucklandia lappa Decne (Compositae) (6 g), and was extracted with eight volumes of water for 1.5 h and then filtered. This extraction was repeated thrice, and the filtrates were combined and decompressed to a density of 1.1–1.2. These filtrates were precipitated using 70% ethanol, left to stand, and filtered. Afterwards, the filtrates were merged with the ethanol extract from A. membranaceus, decompressed to a density of 1.1–1.2, mixed with 4.1 kg of dextrin, and spray-dried. The process was finished in the Shanghai Shuguang Hospital, which is affiliated with the Shanghai University of Traditional Chinese Medicine. A finished product of ZMYL with batch number 20110526 was obtained and used for the experiment.
The quality of ZMYL was controlled by determining the main active ingredients via a validated UPLC/MS method (Gong et al., 2014). The paeoniflorin, albiflorin, scutellarin, astragaloside IV, and wogonin contents in ZMYL batch number of 20110526 were 1.73, 1.38, 0.10, 0.02, and 0.002 mg/g, respectively (Gong et al., Citation2014).
Pretreatment of plasma sample
Preparation of plasma-containing free CDDP: Plasma was handled using an ultra-filtration tube (10 KD), as previously described (Johnsson et al., Citation1997). About 100 µL of the plasma sample was pipetted into an ultra-filtration tube and was centrifuged at 4000 × g for 40 min at 4 °C. The underlayer ultra-filtrate was obtained as the plasma-containing free CDDP.
Microwave-assisted digestion: About 20 µL of the plasma sample/ultra-filtrate was pre-digested overnight with 750 µL of 65% (v/v) HNO3 in a screw-capped digestion jar (cleaned using 35% (v/v) HNO3) and was then digested by applying microwaves. Digestion was conducted at 800 W at 110 °C for 15 min. After deflation, digestion was continued at 800 W at 110 °C for 5 min and then at 1600 W at 160 °C for 15 min. After completing digestion, the fluid was transferred from the digestion jar, and 5% nitric acid was added until a 10 mL volume was reached.
Preparation of internal standard working solution
Simultaneous with the preparation of standard curve, internal standard working solutions of 1 ppm concentration were prepared by precisely adding 5% nitric acid to 100 μL of the internal standard solutions (100 ppm) until a volume of 10 mL was reached.
Mass spectrum condition
Pt analyses were performed using an ICP-quadrupole-MS (Agilent 7500CX, Tokyo, Japan). The sample introduction system consisted of a micromist glass concentric nebulizer, a Peltier-cooled (4 °C) double-pass glass spray chamber, and a quartz torch. Sample transport from the autosampler to the nebulizer was performed using a peristaltic pump. The instrument was cooled by using argon gas of 99.999% purity. Data were acquired and processed using the ICP-MS Expert Software (Agilent Technologies, Santa Clara, CA). The instrument settings of the ICP-MS had the following operating parameters: ICP radio frequency (RF) power, 1500 W; RF matching, 1.80 V; sampling depth, 8.0 mm; carrier gas, 0.90 L/min; makeup gas, 0.21 L/min; nebulizer pump, 0.10 rps; He gas, 4.5 mL/min; extract 1, 0.0 V; extract 2, −120.0 V; cell entrance, −30 V; cell exit, −30 V; QP bias, −16.0 V; Octp bias, −20.0 V.
Method validation
The method validation followed the Guidance for Industry-Bioanalytical Method Validation of the Food and Drug Administration (USA).
Calibration curve, lowest limit of detection (LLOD), and lower limit of quantification (LLOQ): A standard solution of Pt (1000 ppm) was further diluted daily by using 5% nitric acid to obtain the working solutions. A calibration curve was constructed with various Pt concentrations of 0.001, 0.01, 0.1, 1, 10, 100, and 1000 ppb, and the blank solution was 5% nitric acid. The various plasma Pt concentrations were calculated using the linear regression equation obtained from the calibration curve. Quality control (QC) samples were independently prepared in the same manner at low (0.1 ppm), medium (1.0 ppm), and high (100 ppm) levels by using different stock solutions. The acceptance criterion for a calibration curve was a correlation coefficient (r) of 0.99 or better and that each back-calculated standard concentration must be within ±15% deviation from the spiked values (±20% at LLOQ). The LLOQ is defined as the lowest concentration of the calibration curve. The LLOD was determined as the concentration resulting in a peak height greater than three times the baseline noise level (S/N = 3).
Recovery of microwave-induced digestion: 20 µL of blank rat plasma was spiked with 100 µL of three concentration levels of 10000, 100, and 10 ppb of Pt standard solution prior to digestion. After digestion using the above-mentioned protocol, the plasma samples were determined via ICP-MS. The resulting concentrations were compared with those of the standard concentrations (100, 1, and 0.1 ppb) to calculate the recoveries of microwave-induced digestion.
Recovery of ultrafiltration: The Pt standard solutions (100 µL) with concentrations of 10 000, 100, and 10 ppb were pipetted into ultrafiltration tubes (10 KD) and centrifuged at 4000 × g for 40 min at 4 °C. The ultrafiltrate was transferred, and 5% nitric acid was added up to a final volume of 10 mL to determine and calculate the ultrafiltration recoveries.
Precision assay: Precision was determined via quintuple analyses of plasma samples with three different Pt concentrations (0.1, 1.0, and 100.0 ppb). After pretreatment, to determine the intra-day variances, the assays were performed using the same samples at five time intervals within 1 d. Inter-day variances were also determined by assaying the spiked samples over three consecutive days. Relative standard deviations (RSD) were calculated from these sampled values of Pt.
Stability: The stability validation of the analyses was investigated at different conditions. The freeze–thaw stability was determined after three freeze–thaw cycles. The QC samples were thawed at room temperature, re-frozen at −20 °C over three cycles, and assayed. Three aliquots of the QC samples were stored at room temperature for 24 h to cover the sample preparation to determine the short-term stability. The calibration curves of freshly prepared standards were used for all stability tests of the QC samples. The mean values and standard deviations of the ratios between the mean measured concentrations and the spiked concentrations were used for stability evaluation. For the acceptance criterion of stability, the deviation compared with the spiked concentration corresponding to each level should be within ±15% of the nominal concentration.
Pharmacokinetic study
Experiments were performed on 14 rats (200–250 g) that were randomly divided into two groups of 7, the CDDP group and the CDDP combined with the ZMYL group. In the CDDP group, CDDP was freshly dissolved in a saline solution and was intraperitoneally administered to the rats at a dose of 9 mg/kg, which was the clinical equivalent dose of CDDP (1.54 mg/kg). In the CDDP combined with the ZMYL group, ZMYL was dispersed as a suspension in a saline solution and successively administered to the rats by gavage at a dose of 1 g/kg for 4 d. One hour after oral administration of ZMYL at the 4th day, CDDP was administered as in the CDDP group. Blood (of approximately 0.3 mL) was sampled via the postorbital venous plexus veins at 0.17, 0.33, 0.5, 1, 2, 4, 12, 24, 48, and 72 h after CDDP administration while the animals were unrestrained and conscious. The blood samples were immediately centrifuged at 15 700 × g for 10 min to obtain plasma, which was then stored at −80 °C until analysis.
Data analysis
All data were processed using the pharmacokinetic data analysis software program PK solutions 2™ (Summit Research Services, Seattle, WA). The plasma concentration–time curves were plotted. The elimination rate constant (Ke), elimination half-life (T1/2), apparent volume of distribution (Vd), clearance rate (CL), mean residence time (MRT), and other pharmacokinetic parameters were calculated. The area under the plasma concentration–time curve from zero to time t (AUC0–t) was directly obtained from the observed concentration–time data. The area under the plasma concentration–time curve from zero to infinity (AUC0−∞) was calculated using the trapezoidal rule with extrapolation to infinity with the terminal elimination rate constant (Ke). SPSS version 15.0 software (SPSS Inc., Chicago, IL) was used for statistical analyses. The experimental data and the pharmacokinetic parameters were expressed as mean values ± the standard deviation (SD). Significant differences were assessed using Student’s t-test with α = 0.05 as the minimum level of significance.
Results and discussion
Calibration curve, LLOD, and LLOQ: The calibration curve was obtained using seven standard Pt solutions in 5% (v/v) HNO3 from serial dilutions of a Pt standard solution (1000, 100, 10, 1, 0.1, 0.01, and 0.001 ppb) prepared in aqueous and plasma matrices. The instrument responses for Pt in the two matrices differed by less than ± 15%. Therefore, the aqueous calibration was used for all subsequent analyses. When the least-squares method was used, a typical equation for the calibration curve was Y = 3.533 × 10−3X + 1.813 × 10−5 with a correlation coefficient of 1.000, where Y is the Pt response and X is the plasma Pt concentration (in ppb). The LLOD offered by the instrument software was 6.596 × 10−4 ppb, and the present method offered an LLOQ of 0.001 ppb.
Recoveries: The recoveries of microwave-assisted Pt digestion from QC samples at 0.1, 1.0, and 100 ppb were 117.13%, 105.60%, and 101.27%, and the recoveries of ultrafiltration were 102.47, 99.27, and 104.26%, respectively. The recoveries are listed in .
Table 1. Pt recoveries from microwave-assisted digestion and ultrafiltration.
Precision and accuracy: The precision data for the intra- and inter-day analyses and the accuracy of the method are shown in . The intra-day and inter-day precisions of Pt were within 1.86–10.17% for each of the Pt QC levels, which conformed to the criteria for the analysis of biological samples according to FDA guidelines. The accuracy was required to be within ± 15% (20% for LLOQ), and the intra- and inter-day precisions were not to exceed ± 15% (20% for LLOQ). The results demonstrated that the precision and accuracy of the present method were acceptable.
Table 2. The accuracy and precision for the analysis of Pt in rat plasma (intra-day, n = 5; inter-day, n = 3).
Stability: The results from all stability tests are presented in , which indicate that the analyte was stable at routine laboratory conditions. The deviation of the mean test responses was within 1.73–9.86% in all stability tests of QC samples. The short-term room temperature stability and freeze–thaw stability of the simulation sample for 24 h were acceptable. The method is therefore proven to be applicable for routine analysis.
Table 3. Stability of Pt in rat plasma under different storage conditions (means ± SD).
The total Pt plasma concentration–time curves after CDDP administration alone and after CDDP co-administration with ZMYL are shown in . The figure shows that CDDP was immediately absorbed into the blood circulation in the two groups. The peak total Pt plasma concentrations was reached within 10 min after intraperitoneal administration into the rats at a dose of 9 mg/kg for both groups, which was in agreement with previous reports (Johnsson et al., Citation1995). The total Pt plasma concentrations sharply declined followed by a slower phase of decrease. Considering the absence and presence of ZMYL, the compound does not appear to produce any significant influence on the total Pt plasma concentration–time curves.
Figure 1. Mean plasma concentration–time profiles of total Pt (A) and free Pt (B) in rats after intraperitoneal administration of CDDP at a dose of 9 mg/kg and co-administration with ZMYL at a dose of 1 g/kg.
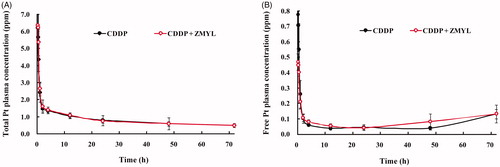
The free Pt plasma concentration–time curves after CDDP administration alone and CDDP co-administration with ZMYL are shown in . In the co-administration with the ZMYL group, the initial free Pt concentrations at 0.17, 0.33, 0.5, and 1 h after intraperitoneal administration of CDDP were notably lower than those in the CDDP group. The free Pt concentrations determined in the CDDP group were 1.65-, 1.58-, 1.37-, and 1.23-times greater than those in co-administration with the ZMYL group. shows that free Pt blood concentrations picked up 72 h after CDDP administration, which was especially apparent in the ZMYL combination group and is consistent with the observation from another study (Vermorken et al., Citation1984). This increase may be due to Pt re-absorption and distribution between tissues and blood.
Generally speaking, the high plasma Pt levels are thought to increase the incidence of CDDP-related toxicity (Erdlenbruch et al., Citation2001). The unbound fraction of a drug is responsible for its pharmacological activity and overall disposition; hence, small changes in the unbound fraction of a highly bound drug could result in a large increase in drug action or side effect (Mills et al., Citation1997). By contrast, a decrease in the free drug fraction in a co-administration group may contribute to a decrease in the incidence of unexpected adverse reactions.
The pharmacokinetic parameters of total and free Pt were calculated in rat plasma of CDDP in the absence or presence of ZMYL (). After administration of clinically tolerable doses of CDDP and ZMYL granules, significant differences were observed between the pharmacokinetic parameters in the two groups, mainly including CL, Ke, MRT, T1/2, and AUC0–∞. Compared with the pharmacokinetic parameters calculated from the total Pt concentration of CDDP in the absence or presence of ZMYL, CL obviously decreased from 110.52 mL h−1 kg−1 to 66.12 mL h−1 kg−1, MRT was extended from 63.10 h to 164.54 h, AUC0–∞ increased from 86.58 µg h mL−1 to 152.93 µg h mL−1, T1/2 was extended from 48.38 h to 126.40 h, and Ke decreased from 0.02 h to 0.01 h in the ZMYL combination group. All these pharmacokinetic parameters exhibited statistically significant differences (p < 0.05).
Table 4. The pharmacokinetic parameters of total and free Pt in rats (means ± SD, n = 7).
The pharmacokinetic parameters calculated from the free Pt concentrations from 0 to 24 h after the administration of CDDP are listed in . Comparing the pharmacokinetic parameters of CDDP from the ZMYL combination group with those of the CDDP only group, the values of AUC, MRT, and T1/2 decreased, whereas Vd, CL, and Ke increased in the ZMYL combination group. However, statistically significant difference (p < 0.05) was only observed in Vd and CL.
Statistical analysis (p < 0.05) of the main pharmacokinetic parameters between the two treatment groups demonstrated that ZMYL could significantly affect the pharmacokinetic properties of CDDP. High free Pt CL was apparent, which demonstrates that free Pt is more quickly cleared from the blood and that the accumulation and toxic possibility of CDDP could be reduced by the combination with ZMYL. The integrative toxicity induced by CDDP was also observed to be alleviated in the ZMYL combination group. Diarrhea was not observed in all animals in the ZMYL combination group, whereas in the CDDP group, severe diarrhea was observed.
Studying the plasma protein binding ratio can provide reference for clinical medication. Medicines with high plasma protein binding ratio have low unbound drug concentration; hence, these drugs slowly pass through biological membranes. In the analysis of CDDP pharmacokinetic parameters by measuring the total and free Pt plasma concentrations, the CDDP plasma protein binding rate for the individual sample time point was determined and compared for CDDP in the absence or presence of ZMYL (). The results indicate that the CDDP plasma protein binding rate within 72 h after CDDP administration was between 72.44% and 95.39% for the CDDP only group and was between 76.42% and 95.26% for the ZMYL combination group. The results were identical with those reported in the literature; CDDP rapidly binds to plasma proteins and 24 h after CDDP administration, and more than 95% of CDDP becomes protein-bound (Vermorken et al., Citation1984; Zemlickis et al., Citation2006).
Figure 2. Comparison of Pt plasma protein-binding rate after CDDP administration between the CDDP only group and the ZMYL combination group (**p < 0.01) (means ± SD, n = 7).
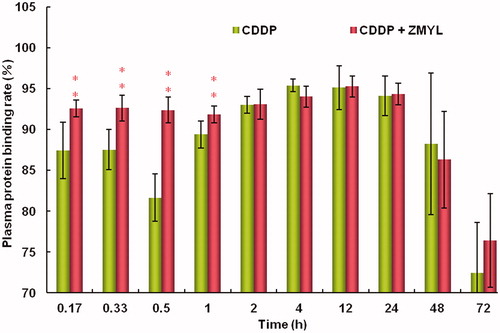
Pt plasma protein-binding ratio in the early stages of drug dosing (at 0.17, 0.33, 0.5, and 1 h after CDDP dosing) was significantly boosted by the co-administration of ZMYL (p < 0.01). In general, the unbound concentration of a drug in the blood more accurately reflects the pharmacological effects and toxicity of the drug than its total concentration in blood because only the drug unbound to blood components is able to diffuse through the membranes and ultimately reach the target organ (Du Souich et al., Citation1993). Furthermore, binding to plasma proteins is also related to the drug distribution volume and clearance, and free Pt gives a better measure of the active portion of Pt in the plasma. Clinical studies have shown correlations between free Pt and nephrotoxicity, as well as with tumoricidal effect (Johnsson et al., Citation1996).
The protein-bound drug is considered to be biologically inactive and functions as the stockpile of free Pt. CDDP belongs to high plasma protein binding rate drug. It was demonstrated to have low free drug and to have a comparatively slow speed of tissue transfer; hence, CDDP does not easily lead to adverse reactions and it can guarantee security in medication. Changes in plasma protein binding of highly bound drugs are generally recognized to often result in changes in the apparent volumes of distribution (Gibaldi & Perrier, Citation1977). By co-administration with ZMYL, the Pt plasma protein binding rate within the initial phase after CDDP administration was further improved. Free Pt concentration decreased, and the result seems to support that CDDP-induced side effects could be avoided by reducing the initial high blood concentration. However, whether or not the decline in initial blood drug concentration will affect pharmacological efficacy remains a question for further studies.
Herbs–drug interactions are an on-going concern in the treatment of chemotherapy. It can occur at all levels, including in pharmacokinetics and pharmacological, and affect plasma drug concentration, which is an important factor to determine the anti-tumor effect and drug toxicity towards normal tissues. Failure to recognize them can lead to overdosing or under treatment, with severe clinical consequences. As a potential complementary and alternative medicine during CDDP chemotherapy, ZMYL has already been proved to enhance the tumor inhibitory effect and reduce side effect of CDDP (Li et al., Citation2012; Qi et al., Citation2012; Zhang et al., Citation2011). The present results indicate that some pharmacokinetic herbs–drug interactions have taken place in ZMYL and CDDP, and it could provide a powerful pharmacokinetic evidence for the combination of CDDP and ZMYL. However, it is worth knowing how the pharmacokinetic herbs–drug interactions take place.
Conclusion
In summary, ICP-MS combined with ultra-filtration and microwave-assisted digestion was established and validated for determining the total and free Pt concentration in rat plasma as well as the plasma protein binding rate after intraperitoneal administration of CDDP with or without ZMYL. Based on the results, the main pharmacokinetic parameters and plasma protein binding rate of CDDP in normal animals are conclusively influenced by ZMYL. However, whether or not ZMYL could produce clinically significant pharmacokinetic interactions with CDDP is yet to be proven in tumor-bearing animal models or in patients in clinical studies.
Declaration of interest
The authors have declared that there is no conflict of interest. The authors gratefully acknowledge grants from the National Nature Science Foundation of China (No. 81173291), the Construction Program for Innovative Research Team in Shanghai Institutions of Higher Education (2009), and the Program for Science and Technology Commission of Shanghai Municipality (06DZ19704) for their financial support in this study.
References
- Alderden RA, Hall MD, Hambley TW. (2006). The discovery and development of cisplatin. J Chem Educ 83:728–34
- Attia SM. (2011). Influence of resveratrol on oxidative damage in genomic DNA and apoptosis induced by cisplatin. Mutat Res Gen Tox EM 741:22–31
- Besançon OG, Tytgat GAM, Meinsma R, et al. (2012). Synergistic interaction between cisplatin and gemcitabine in neuroblastoma cell lines and muticellular tumor spheroids. Cancer Lett 319:23–30
- Cao Y, Xia QH, Ma H, Zhong AP. (2008). Pharmacological effects of serum containing Chinese medicine Bushen Huayu Jiedu compound recipe in lung cancer drug-resistance cells. Chin J Integr Med 14:46–50
- Chen C, Wang HC, An X, et al. (2012). Triplet combination with paclitaxel, cisplatin and 5-FU is effective in metastatic and/or recurrent nasopharyngeal carcinoma. Cancer Chemother Pharmacol 11:1–8
- Chen LG, Hung LY, Tsai KW, et al. (2008). Wogonin, a bioactive flavonoid in herbal tea, inhibits inflammatory cyclooxygenase-2 gene expression in human lung epithelial cancer cells. Mol Nutr Food Res 52:1349–57
- Du Souich P, Verges J, Erill S. (1993). Plasma protein binding and pharmacological response. Clin Pharmacokinet 24:435–5
- Dwivedi C, Agrawal P, Natarajan K, Sharma H. (2005). Antioxidant and protective effects of amrit nectar tablets on adriamycin- and cisplatin-induced toxicities. J Alternat Complement Med 11:143–8
- Erdlenbruch B, Nier M, Kern W, et al. (2001). Pharmacokinetics of cisplatin and relation to nephrotoxicity in paediatric patients. Eur J Clin Pharmacol 57:393–402
- Gibaldi M, Perrier D. (1977). Drug distribution in renal failure. Am J Med 62:471–4
- Gong C, Qian L, Yang H, et al. (2014). Simultaneous determination of five active ingredients in Zengmian Yiliu granules by UPLC-MS. Chin Tradit Patent Med 36:301–5
- Hanada T, Isobe H, Saitoh T, et al. (1998). Inductively coupled plasma mass spectrometry for the determination of platinum accumulation in human non-small cell lung cancer cell lines. Int J Clin Oncol 3:98–101
- Hu XX, Zhang QH, Qi C, Li JX. (2012). Anti-angiogenic effects of Zengmian Yiliu granule on ovarian carcinoma xenograft. Chin J Integr Med 32:970–4
- Huang ZH, Qi C, Zhang QH. (2004). Effect on bcl-2, bax gene expression in rat with ovarian cancer treated by cisplatin and “Zengmian Yiliu Mixture”. J Tradit Chin Med 2:63–5
- Johnsson A, Björk H, Schütz A, Skärby T. (1997). Sample handling for determination of free platinum in blood after cisplatin exposure. Cancer Chemother Pharmacol 41:248–51
- Johnsson A, Höglund P, Grubb A, Cavallin-Sta E. (1996). Cisplatin pharmacokinetics and pharmacodynamics in patients with squamous-cell carcinoma of the head/neck or esophagus. Cancer Chemother Pharmacol 39:25–33
- Johnsson A, Olsson C, Nygren O, et al. (1995). Pharmacokinetics and tissue distribution of cisplatin in nude mice: Platinum levels and cisplatin-DNA adducts. Cancer Chemother Pharmacol 37:23–31
- Laghari AJ, Khuhawar MY, Zardari LA, Bhatti AG. (2008). GC determination of cisplatin in serum and urine of cancer patients after chemotherapy as platinum (II) pyrrolidinedithiocarbamate chelate. Chromatographia 67:749–54
- Li JX, Zhang QH, Hu XX, Qi C. (2012). Effects of Zengmian Yiliu decoction on expression of resistance related genes HIF-1, Glut1, MDR1, P-gp in nude mice with cisplatin-resistant ovarian cancer. J Tradit Chin Med 46:61–4
- Mills PC, Ng JC, Auer DE. (1997). The effect of the acute-phase response on in vitro drug metabolism and plasma protein binding in the horse. Vet Res Commun 21:361–8
- Minakata K, Suzuki M, Nozawa H, et al. (2006). Platinum levels in various tissues of a patient who died 181 days after cisplatin overdosing determined by electrospray ionization mass spectrometry. Forensic Toxicol 24:83–7
- Minami T, Ichii M, Okazaki Y. (1995). Comparison of three different methods for measurement of tissue platinum level. Biol Trace Elem Res 48:37–45
- Nygren O, Lundgren C. (1997). Determination of platinum in workroom air and in blood and urine from nursing staff attending patients receiving cisplatin chemotherapy. Int Arch Occ Env Hea 70:209–14
- Paksoy M, Ayduran E, Sanli A, et al. (2011). The protective effects of intratympanic dexamethasone and vitamin E on cisplatin-induced ototoxicity are demonstrated in rats. Med Oncol 28:615–21
- Park HR, Ju EJ, Jo SK, et al. (2009). Enhanced antitumor efficacy of cisplatin in combination with HemoHIM in tumor-bearing mice. BMC Cancer 9:85–95
- Qi C, Zhang QH, Li JX, Hu XX. (2012). Effects of Zengmian Yiliu recipe combined cisplatin on the tumor inhibition rate in platinum-resistant ovarian cancer. Chin J Integr Med 32:817–21
- Rosenberg B. (1985). Fundamental studies with cisplatin. Cancer 55:2303–16
- Suddek GM. (2010). Sunitinib improves chemotherapeutic efficacy and ameliorates cisplatin-induced nephrotoxicity in experimental animals. Cancer Chemother Pharmacol 67:1035–44
- Türk G, Ateşşahin A, Sőnmez M, et al. (2008). Improvement of cisplatin-induced injuries to sperm quality, the oxidant-antioxidant system, and the histologic structure of the rat testis by ellagic acid. Fertil Steril 89:1474–81
- Vermorken JB, Vander Vijgh WJ, Klein I, et al. (1984). Pharmacokinetics of free and total platinum species after short-term infusion of cisplatin. Cancer Treat Rep 68:505–13
- Wang CZ, Fishbein A, Aung HH, et al. (2005). Polyphenol contents in grape-seed extracts correlate with antipica effects in cisplatin-treated rats. J Alternat Complement Med 11:1059–65
- Wang D, Song MS, Yang GL. (2003). Protein binding study of perillyl alcohol enantiomers by high performance frontal analysis. J Liq Chromatogr RT 26:2861–71
- Xian GY, Wang S. (2010). The research of Zingiberis recentis decoction for purging stomach-fire against cisplatin-induced vomiting in mice. Guide Chin Med 8:47–8
- Xu WZ, Zhou L, Ma XN, et al. (2011). Therapeutic effects of combination of paeoniflorin and albiflorin from Paeonia radix on radiation and chemotherapy-induced myelosuppression in mice and rabbits. Asian Pac J Cancer Prev 12:2031–7
- Yamada K, Kato N, Takati A, et al. (2005). One-milliliter wet-digestion for inductively coupled plasma mass spectrometry (ICP-MS): Determination of platinum-DNA adducts in cells treated with platinum (II) complexes. Anal Bioanal Chem 382:1702–7
- Yu JQ, Liu HB, Lei JC, et al. (2007). Antitumor activity of chloroform fraction of Scutellaria barbata and its active constituents. Phytother Res 21:817–22
- Zemlickis D, Klein J, Moselhy G, Koren G. (2006). Cisplatin protein binding in pregnancy and the neonatal period. Med Pediatr Oncol 23:476–9
- Zhang QH, Hu XX, Qi C, Li JX. (2011). Experimental study on regulating effects of Zengmian Yiliu granule on immunosuppression and myelosuppression following large-dose chemotherapy in ovarian carcinoma. J Tradit Chin Med 45:71–4
- Zhang QH, Qi C, Wang RJ. (2012). Influence of Zengmian Yiliu granule on quality of life in patients with advanced ovarian cancer during chemotherapy period. J Tradit Chin Med 46:41–4