Abstract
Context and objective: The herb fenugreek, Trigonella foenum-graecum Linn (Fabaceae), seeds have been traditionally used in the treatment of diabetes but its effect on oxidative stress and pro-inflammatory cytokines in the improvement of exocrine function of diabetes has not been studied. The effect of hydroalcoholic extract of Trigonella foenum-graecum seeds (HEF) on alloxan-induced type-II diabetic rat model was investigated.
Materials and methods: Effect of HEF (500, 1000, and 2000 mg/kg), glimepiride (4 mg/kg), and combination of HEF (500 mg/kg) + glimepiride (2 mg/kg), on alloxan-induced diabetic rats was evaluated by assaying (blood glucose, serum protein, glycosylated hemoglobin, muscle and liver glycogen, glucose uptake by diaphragm, liver glucose transport, serum pancreatic enzymes (α-amylase, lipase), pro-inflammatory cytokines (TNF-α, IL-6), antioxidant enzymes [glutathione (GSH), superoxide dismutase (SOD)], lipid peroxides (liver and pancreas), and histoarchitecture (liver, pancreas).
Results: Treatment with HEF (at different doses), glimepiride, and HEF + glimepiride increased body weight and glucose uptake, reduced plasma glucose, glycosylated hemoglobin, liver glucose transport, pro-inflammatory cytokines, pancreatic enzymes and restored depleted glycogen (muscle, liver) and total protein significantly (p < 0.01) and dose dependently, including prevention of lipid peroxidation and restoration of GSH and SOD (liver and pancreas). Treatment with HEF + glimepiride potentiated hypoglycemic activity of glimepiride. Histoarchitecture of liver and pancreas showed marked improvement.
Conclusion: Present experimental findings suggest that HEF possesses promising hypoglycemic activity, presumably by amelioration of oxidative stress and pro-inflammatory cytokines. HEF may be useful as an adjuvant with clinically effective antidiabetic drugs in the management of type-II diabetes.
Introduction
Diabetes mellitus, a life-threatening metabolic disorder of which type-II diabetes is more prevalent (more than 90% of all diabetes cases), affects 5.9% of the world's adult population with almost 80% of the total in developing countries (Sicree et al., Citation2006). Increased blood glucose level in diabetes mellitus (due to impaired carbohydrate metabolism) causes increased formation of free radical production, reactive oxygen species (ROS), serum triglyceride, and lipoproteinemia. Increased free radicals and ROS will trigger peroxidation of lipids, which in turn stimulate glycation of proteins, depletion of antioxidant enzymes, and alteration in signaling pathways, ultimately manifest low-grade inflammatory reactions responsible for diabetic complications (Dominiczak, Citation2003; Evans et al., Citation2002). Epidemiological, clinical, and experimental studies have established a close relationship between oxidative stress and low-grade inflammation which are major cellular events for the development and progression of type-II diabetes mellitus (Hays et al., Citation2008). Further, glucose (which itself is a pro-inflammatory) and the elevated acute-phase inflammatory markers, tumor necrosis factor-alpha (TNF-α), interleukin (IL-6), and C-reactive protein (CRP) are actively participating in the development of insulin resistance and metabolic syndrome and play a special role in the genesis of chronic low-grade inflammation in type-II diabetes mellitus (Niehoff et al., Citation2007; Sjöholm & Nyström, Citation2005). Keeping in mind, the occurrence of multi physiological, cellular events and existence of close relationship between insulin resistance and inflammatory process, it is envisaged that therapeutic strategies must reduce inflammation biomarkers which might serve a promising avenue for diagnosis as well as development of more effective therapeutics (Evans et al., Citation2002; Guest et al., Citation2008; Marx et al., Citation2003).
Alloxan has two distinct pathological effects: it selectively inhibits glucose-induced insulin secretion through selective inhibition of glucokinase (a glucose sensor of the β-islet) that causes a state of diabetes through the induction of ROS formation, causing selective necrosis of β-islet (Lenzen, Citation2008). Thus, among the numerous animal models, alloxan-induced diabetic model represents both type-I and type-II diabetes mellitus by selecting appropriate doses (Cooperstein & Watkins, Citation1981).
Currently used antidiabetic drugs are associated with numerous side effects, high cost, and poor availability for a large part of the rural population. Hence, efforts are made to develop antidiabetic drugs from traditional herbal remedies, as they are devoid of side effects and help us to develop complementary drug therapy in the management of diabetes (Baig et al., Citation2012; Martinello et al., Citation2006).
It has been documented that fenugreek [Trigonella foenum-graecum Linn (Fabaceae)] seeds treatment to alloxan-induced diabetic rats decreased plasma glucose, increased plasma insulin level (Yadav et al., Citation2008), reduced oxidative stress by preventing lipid peroxidative products, and restoring enzymatic antioxidants (Devasena & Menon, Citation2002; Hamadi, Citation2012). The whole powder of fenugreek seeds and its methanol extract showed a significant hypoglycemic activity, when fed simultaneously with glucose to rats (Ali et al., Citation1995). The seed fiber of fenugreek reduces the rate of glucose absorption from gastro-intestinal tract and delay gastric emptying; thereby preventing hyperglycemia after meal (Gupta et al., Citation2001), in addition, aqueous seed extract also showed a significant fall in blood glucose in normoglycaemic mice (Zia et al., Citation2001). Thus, fenugreek seeds may be useful as a functional food with therapeutic value.
Fenugreek has not been investigated so far for its effect on pro-inflammatory mediators and pancreatic enzymes in alloxan-induced diabetes. Therefore, the objective of the present study was to explore probable involvement of pro-inflammatory markers, oxidative stress, and pancreatic enzymes in diabetes mellitus, and to evaluate the effect of fenugreek on such sensitive parameters. Efforts were also made to understand the probable correlation among hypoglycemic activity, oxidative stress, pro-inflammatory markers, and pancreatic enzymes, in alloxan-induced diabetes. The likely mechanism(s) of antidiabetic activity of fenugreek is also discussed.
Materials and methods
Drugs and chemicals
The pure and standard powdered hydroalcoholic extract of Trigonella foenum-graecum (HEF) was obtained as a gift sample from Natural Remedies Pvt Ltd., Bangalore, India. Alloxan monohydrate (ALX) (Explicit Chemicals Pvt Ltd, Pune, India) and glimepiride (Torrent Pharmaceuticals, Ahmedabad, India) were obtained as a gift sample. Trichloro-acetic acid, potassium dihydrogen phosphate, disodium hydrogen phosphate (Merck, Darmstadt, Germany), thiobarbituric acid, anthrone (Loba Chemie, Mumbai, India), GOD/POD kit (Biolab Diagnostics, Mumbai, India), 5,5-dithiobis (2-nitrobenzoic acid) (Sisco Research Laboratories, Mumbai, India), rat TNF-α and IL-6, ELISA kits (Thermofisher Scientific, Waltham, MA), and other required diagnostic reagents (Biolab, Span and Tulip Diagnostic Pvt. Ltd., Mumbai, India) for various biochemical assays were procured. All other reagents and chemicals used were of analytical grade and purchased from Qualigens (India) and local suppliers.
Phytochemical properties of HEF
The preliminary phytochemical analysis of hydroalcoholic extract of Trigonella foenum-graecum (HEF) was performed (Khandelwal, Citation2009; Kokate, Citation2008) for carbohydrates (Molisch test), reducing sugar (Fehling's and Benedict's test), monosaccharide (Barfoed's test), pentose sugar (Bial's test), hexose sugar (Selwinoff's test and Cobalt chloride test), protein (Biuret test, Millon's test, and Xanthoprotein test), alkaloid (Mayer's, Wagner's, Hager's, and Dragendorff's test), amino acids (Ninhydrine test), glycosides (Borntrager's, Legal's, and Keller Kelliani's test), saponins (Foam and bromine water test), flavonoids (Ferric chloride and Shinoda test), tannins (Ferric chloride and bromine water test), steroids and triterpenoids (Salkowaski's and Liebermann–Burchardt test).
Physico-chemical properties of HEF
The physico-chemical properties of HEF, namely, physical appearance, solubility, UV, and FTIR and HPTLC, were studied.
High performance thin layer chromatography (HPTLC) analysis of HEF
Sample preparation: HEF 50 mg was dissolved in 1 ml of methanol and sample of 5, 10, and 20 µl were applied as 8 mm wide bands, under a continuous flow of nitrogen, using a CAMAG LINOMATE V automatic sample applicator. Sample was applied with a 100 µl syringe (Hamilton, Bonaduz, Switzerland) at a constant rate (0.1 µl/s) and the distance between adjacent bands was 15 mm.
Stationary phase: the 10 × 10 cm aluminum-backed HPTLC plate coated with 250 µm layers of silica gel G 60 F254 (E. Merck, Darmstadt, Germany) was prewashed with methanol and activated at 110° C for 10 min was used as a stationary phase.
A mixture of n-butanol:acetic acid:water [(3:1:1) (v/v/v)] was used as a mobile phase.
The plate was developed in an ascending manner with a solvent system, as mentioned above, in a chamber pre-saturated with the solvent system. Developing distance was 1 cm from lower edge of the plate. The length of each chromatogram run was 8 cm. The developed plates were air dried. Plate was scanned at 580 nm using scanner-4 (CAMAG) operated in reflectance–absorbance mode and controlled by Win CATS software (Version 1.43, Camag, Muttenz, Switzerland). The slit dimensions were 5 × 0.45 mm and the scanning speed was 20 mm/s. The source of radiation used was deuterium lamp emitting continuous UV radiation between 200 and 400 nm. Plates were derivatized using the ninhydrine reagent. The obtained profile was analyzed at 580 nm, before and after derivatization of the plate (Brandsteterova & Ziakova-Caniova, Citation2002).
Experimental animals
Sprague–Dawley rats of either sex (150–200 g) were obtained from Haffkine's Institute for Training Research and Testing, Mumbai, India. Animals were maintained in our animal house, under standard laboratory conditions: temperature (25 ± 2 °C), relative humidity (50 ± 10%), and 12 h (light) and 12 h (dark) cycles throughout the experimental period. Animals had free access to feed (Standard pellet diet, Amrut feed, Pune, India) and water ad libitum. The experimental protocols were approved by Institutional Animal Ethics Committee (SIPS/IAEC/2011-12/12) and animals were handled by adhering the guidelines of Committee for the Purpose of Control and Supervision of Experiments on Animals (CPCSEA).
Induction of diabetes
Alloxan (ALX) is a urea derivative which causes selective necrosis of the pancreatic islets of β cells. It is used to produce experimental diabetes in animals such as rabbits, rats, mice, and dogs. Diabetes was induced in overnight fasted rats by injecting freshly prepared ALX (in normal saline) at a single dose of 150 mg/kg, intra-peritoneally (i.p.). The blood samples were collected through tail vein on the 5th day and glucose was assayed by the glucose oxidase–peroxidase (GOD/POD) method to confirm diabetes. The rats showing blood glucose level >250 mg % were considered as diabetic and selected for the studies (Etuk, Citation2010; Mandlik et al., Citation2008; Shikarvar & Patil, Citation2010).
Experimental design and treatment schedule
In this experiment, a total of 48 rats (12 normal; 36 diabetic rats) were used. The normal control rats, two groups (six rats/group) and randomly divided diabetic rats (5 d after ALX treatment) six groups (six rats/group) were received the treatment as mentioned below.
Group I: normal control rats (NC) received 1% w/v CMC (1 ml/100 g, B/W), daily orally for 30 d.
Group II: normal control rats (NC) received HEF 1000 (mg/kg), daily orally for 30 d.
Group III: diabetic control rats (DNC) received 1% w/v CMC (1 ml/100 g, B/W), daily orally for 30 d.
Group IV: diabetic rats received HEF (500 mg/kg), daily orally for 30 d.
Group V: diabetic rats received HEF (1000 mg/kg), daily orally for 30 d.
Group VI: diabetic rats received HEF (2000 mg/kg), daily orally for 30 d.
Group VII: diabetic rats received glimepiride (4 mg/kg), daily orally for 30 d.
Group VIII: diabetic rats received HEF (500 mg/kg, p.o.) + glimepiride (2 mg/kg), daily orally for 30 d.
On the 31st day, the overnight fasted rats were anaesthetized with ketamine (80 mg/kg, i.p.), and blood samples were collected, directly from the heart, with and without anticoagulant for plasma and serum separation, respectively. The rats were sacrificed by cervical decapitation. The tissues, namely, liver, muscle, diaphragms, and pancreas were excised, blotted, weighed, and stored at −80 °C for the further studies. Tissue homogenates of liver and pancreas were preserved in 0.2 M phosphate buffer pH 7.4.
Determination of body weight and plasma glucose
During the treatment period, body weights of the rats were determined on 0 and 31st day. One h after vehicle/drug/extract treatment, blood samples were collected from retro-orbital plexus of each rat under mild anesthesia on 0 (i.e., 5th day after alloxan treatment), 7th, 14th, 21st, and 31st day, and plasma glucose was assayed by the glucose oxidase method (Trinder, Citation1969).
Glycosylated hemoglobin (HbA1c) and tissue glycogen
HbA1c and glycogen content of liver and muscle tissues were determined by the methods of Parker et al. (Citation1981) and Carroll et al. (Citation1956), respectively.
Glucose uptake by hemidiaphragm and glucose transport in liver
The isolated diaphragms and livers from treated rats were excised, placed in ice cold perfusion solution, and incubated with glucose (400 mg %) for 1 h. The glucose uptake by diaphragm was expressed as mg/100 mg dry weight of hemidiaphragm/h.
The glucose transport (glycogenolysis) was studied in liver and expressed as mg of glycogen formation per g dry weight of liver/h (Carroll et al., Citation1956).
Serum total protein
Serum total protein (Lowry et al., Citation1951) of rats was determined using reagents kits.
Pro-inflammatory mediators (TNF-α and IL-6) in serum
Groups of rats treated with HEF (500 and 1000 mg/kg, p.o.), glimepiride (4 mg/kg, p.o.), and HEF (500 mg/kg, p.o.) + glimepiride (2 mg/kg, p.o.) were used for serum TNF-α and IL-6 assays. Assays of TNF-α and IL-6 in rat serum were performed using ELISA reagents, following the manufacturer's instructions. Both the assays are based on the solid phase indirect enzyme linked immuno-sorbent assay (ELISA) and performed using readymade reagents and microtitre plate. The final reaction was read at 450 nm. The concentration of serum TNF-α and IL-6 was calculated from the plotted standard curve of concentration versus absorbance and expressed as pg/ml (Ashcroft et al., Citation1988).
Pancreatic enzymes assay
Serum α-amylase
The direct amylase assay involves the use of a chromogenic substrate CNP G3 (2-chloro-4-nitrophenyl linked with galacto maltoside) which acts on α-amylase to release more than 95% of 2-chloro-4-nitrophenyl (CNP) and forms 2-chloro-4-nitrophenyl-α-d-maltoside (CNP G2), maltotriose (G3), and glucose (G). The rate of formation of CNP G2 is proportional to the α-amylase activity of the sample, and the assay was performed by sandwich ELISA at 405 nm. The activity of α-amylase was expressed as U/L (Jung et al., Citation1989). The activity of α-amylase in IU/L = absorb/min × 4640 (kinetic factor). A kinetic factor 4640 is a millimolar extinction coefficient of CNPG3 at 405 nm.
Serum lipase
A lipase specific antibody, immobilized on a test tube wall, binds lipase. A second antibody, peroxidase conjugated specific for a separate lipase antigen, binds to the lipase molecule and thus forms a sandwich. After a wash, incubated for 60 min with a chromogen substrate solution, the increase in absorbance was measured at 492 nm using an ELISA reader and the activity of α-amylase was expressed as U/L (Lott et al., Citation1986).
Tissue antioxidants {lipid peroxidation [malondialdehyde (MDA) formation], reduced GSH, and SOD}
Quantitative assay of MDA formation was performed by measuring the concentration of thiobarbituric acid-reactive substances (TBARS) in 10% liver and pancreas's homogenates by the method of Ohkawa et al. (Citation1995). The results were expressed as nM of MDA/g of wet tissue using a molar extinction coefficient of chromophore (1.56 × 10−5/M/cm) and 1,1,3,3-tetraethoxypropane as a standard.
GSH was assayed in liver and pancreas homogenates, by the method of Buetler et al. (Citation1963) using DTNB. The color developed was read at 412 nm against blank on a UV spectrophotometer. The amount of reduced GSH was expressed as μg/g of wet tissue.
The activity of SOD in liver and pancreas was assayed by the method of Mishra and Fridovich (Citation1972). The absorbance was read at 480 nm against blank on a spectrophotometer. The SOD activity was expressed as units/g of wet tissue (Mishra & Fridovich, Citation1972).
Histoarchitecture studies of pancreas and liver
The pancreas and liver sections from normal control, diabetic control, and the drug-treated groups were blotted free of mucus. The tissues were washed in normal saline, cut into pieces of 5 µm thickness, and fixed in 10% neutral buffered formalin solution for 24 h. After fixation, the tissues were cleaned and embedded in paraffin. Tissue sections were mounted on slides, stained with hematoxylin and eosin and observed under light microscope by multiple magnifications for histoarchitecture alterations. The assessment was conducted by a pathologist in a blinded fashion.
Statistical analysis
Results were expressed as mean ± S.E.M. A one-way analysis of variance (ANOVA) followed by Dunnet's test was used for statistical evaluation. p Values less than 0.05 were considered statistically significant.
Results
Phytochemical properties of HEF
The phytochemical analysis of HEF showed the presence of flavonoids, glycosides, amino acids, alkaloids, carbohydrates, phenolic compounds tannins, and proteins.
Physico-chemical properties of HEF
The physico-chemical properties of HEF, namely, physical appearance, solubility, UV, and FTIR, and HPTLC, are outlined in .
Table 1. Phytochemical properties of HEF.
The optimized HPTLC 3-D fingerprint of HEF using a CAMAG TLC scanner is shown in and . The results of the HPTLC chromatogram at 254 nm showed the presence of total seven components, out of which three important components along with their Rf values and area (%) details are outlined sequentially as − 0.37 (9.19%), 0.63 (53.89%), and 0.81 (33.57%). Component number 5 (Rf −0.63) showed maximum concentration (53.89%) and is found to be 4-hydroxyisoleucine, whereas Rf −0.81(33.57%) may be quercetin, on the basis of Rf−0.89 value, reported by our laboratory under similar condition.
Determination of plasma glucose
The initial plasma glucose of the diabetic rats, on the 5th day of alloxan treatment, was 252 mg%, and increased to 279 ± 10 mg% on the 31st day. HEF (500, 1000, and 2000 mg/kg) treatment to alloxan diabetic rats produced a steady, dose-dependent decrease in plasma glucose during the study period (7, 14, 21, and 31st day). Glimepiride (4 mg/kg) and HEF (500 mg/kg) + glimepiride (2 mg/kg) treatment also lowered the plasma glucose significantly on different days. The plasma glucose lowering effect of combined treatment of HEF (500 mg/kg) + glimepiride (2 mg/kg) was comparable with that of glimepiride (4 mg/kg). HEF 1000 (mg/kg) per se treatment to normal rats did not elicit any significant alterations in plasma glucose level on different days studied ().
Table 2. Effect of HEF treatment (30 d) on plasma glucose levels (mg %) of alloxan-induced diabetic rats.
Treatment with HEF 1000 (mg/kg) to normal rats, for 30 d, failed to produce a significant change in antioxidants, tissue glycogen content, and other glucose utilization process including the pancreatic enzymes. Hence, the results are not included.
HbA1c and tissue glycogen
A significant elevation of glycosylated hemoglobin was observed (10.78%) in alloxan-induced diabetic control rats. The treatment of diabetic rats with HEF (500, 1000, and 2000 mg/kg) and glimepiride (4 mg/kg), for 30 d, decreased the elevated glycosylated hemoglobin significantly. Similarly, treatment with HEF (500 mg/kg) + glimepiride (2 mg/kg) also reduced glycosylated hemoglobin significantly and the effect was comparable with that of glimepiride (4 mg/kg) treatment.
There was a significant depletion (p < 0.01) of liver and muscle glycogen of diabetic control rats. The treatment of diabetic rats with HEF (500, 1000, and 2000 mg/kg) prevented the depletion of liver and muscle glycogen significantly. Glimepiride (4 mg/kg) treatment also showed similar effects on muscle and liver glycogen. The prevention of the depletion of muscle and liver glycogen by combined treatment of HEF (500 mg/kg) + glimepiride (2 mg/kg) was comparable with that of the glimepiride (4 mg/kg) treatment group ().
Table 3. Effect of HEF treatment (30 d) on HbA1c, liver and muscle glycogen of alloxan-induced diabetic rats.
Glucose uptake by hemidiaphragm and glucose transport in liver
Diabetic rats treated with HEF (500, 1000, and 2000 mg/kg), glimepiride (4 mg/kg), and HEF (500 mg/kg) + glimepiride (2 mg/kg) for 30 d significantly enhanced glucose uptake by hemidiaphragm and reduced glucose transport (glycogenolysis, in vitro) from liver. The effect of combined treatment of HEF (500 mg/kg) + glimepiride (2 mg/kg) was comparable with that of the glimepiride (4 mg/kg) treatment group. Serum protein level and body weight were significantly reduced in diabetic rats. Treatment with HEF and glimepiride to diabetic rats restored the decreased serum protein as well as body weight significantly. The treatment with HEF 1000 (mg/kg) to normal rats improved the body weight significantly ().
Table 4. Effect of HEF treatment (30 d) on glucose uptake by hemidiaphragm and glucose transport in liver and total protein and body weights in alloxan-induced diabetic rats.
Pro-inflammatory mediators (TNF-α and IL-6) in serum
Serum TNF-α and IL-6 levels were elevated significantly (p < 0.01) in diabetic control rats. Treatment of diabetic rats with HEF (500 and 1000 mg/kg), glimepiride (4 mg/kg), and HEF (500 mg/kg) + glimepiride (2 mg/kg) for 30 d, significantly (p < 0.01 and p < 0.001, respectively) decreased serum TNF-α and IL-6 levels ().
Table 5. Effect of HEF treatment (30 d) on serum cytokines (TNF-α and IL-6) and pancreatic enzymes (α-amylase and lipase) of alloxan-induced diabetic rats.
Pancreatic enzymes
Serum α-amylase and lipase were elevated significantly (p < 0.01) in alloxan-induced diabetic control rats, on the 31st day. The treatment of diabetic rats with HEF (500, 1000, and 2000 mg/kg), glimepiride (4 mg/kg), for 30 d, significantly (p < 0.01) decreased the elevated α-amylase and lipase activity. The effect of combined treatment of HEF (500 mg/kg) + glimepiride (2 mg/kg) was equal to that of the glimepiride (4 mg/kg) treatment group ().
Tissue antioxidants
A significant increase in the MDA formation in liver and pancreas of diabetic rats was observed. Oral administration of HEF (500, 1000, and 2000 mg/kg), glimepiride (4 mg/kg), and HEF (500 mg/kg) + glimepiride (2 mg/kg) to diabetic rats significantly prevented the increased formation of MDA, both in liver and in pancreas ().
Table 6. Effect of HEF treatment (30 d) on GSH, SOD and MDA in liver and pancreas of alloxan induced diabetic rats.
The antioxidants, GSH and SOD, were significantly decreased in liver and pancreas of diabetic rats. Treatment of diabetic rats with HEF (500, 1000, and 2000 mg/kg), glimepiride (4 mg/kg), and HEF (500 mg/kg) + glimepiride (2 mg/kg), significantly restored the depleted GSH and SOD levels in liver and pancreas. The effect of HEF (2000 mg/kg) treatment was found to be equivalent to that of glimepiride (4 mg/kg). Further the treatment of HEF (500 mg/kg) + glimepiride (2 mg/kg) showed an equivalent effect to that of glimepiride (4 mg/kg) treatment ().
Histoarchitecture studies of islets of β-Langerhans and liver
Photomicrographs of histoarchitecture of rat pancreas of β-islets of Langerhans: normal control rats [A] showed a typical histoarchitecture of rat pancreas with normal β-islets and acini cells, diabetic control rats [B] showed marked shrinkage of β-islets (both in size and number), inflammation and shrinkage of acini cells and HEF (1000 mg/kg) treated rats [C] showed improved (mild to moderate) histoarchitecture of β-islets of pancreas, and improved acini cells morphology. Glimepiride (4 mg/kg) treated rats [D] showed improved (moderate) β-islets architecture and acini cells morphology. The HEF (500 mg/kg) + glimepiride (2 mg/kg) treated rats [E] showed improved (mild to moderate) histoarchitecture of β-islets of pancreas, along with acini cells, and was similar to that of glimepiride (4 mg/kg) treatment ().
Figure 3. Histoarchitecture studies of rat pancreas of islets of Langerhans at magnification of 100×. (A) Normal control, (B) diabetic control, (C) HEF (1000 mg/kg, p.o.), (D) glimepiride (4 mg/kg, p.o.), and (E) combination (HEF extract 500 mg/kg, p.o. and glimepiride 2 mg/kg, p.o.).
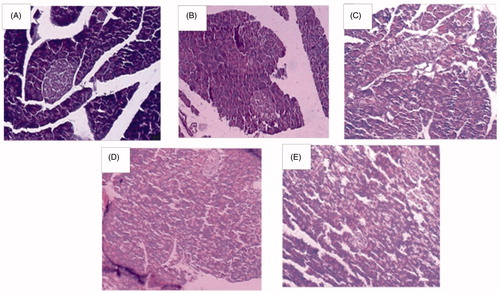
Photomicrographs of histoarchitecture of rat liver: normal control rats [A] showed a typical normal histoarchitecture, diabetic control rats [B] showed (marked) liver infiltration and hypertrophy of hepatocytes, HEF (1000 mg/kg) treated rats [C] liver showed mild decreased in infiltration and moderately preserved hepatocytes architecture, Glimepiride (4 mg/kg) treatment [D] showed improved hepatocytes histoarchitecture with moderate infiltration. The HEF (500 mg/kg) + glimepiride (2 mg/kg) treatment [E] showed improved (mild infiltration) hepatocytes histoarchitecture ().
Discussion
Development of hyperglycemia in experimental animals following alloxan administration is primary due to (a) specific inhibition of glucokinase and (b) selective necrosis of β-islets of Langerhans of pancreas (Lenzen, Citation2008) causing insulin deficiency. Alloxan and its major metabolic product, dialuric acid establishes a redox cycle with the formation of superoxide radicals and undergo dismutation to form hydrogen peroxides. Hydrogen peroxides directly elicit toxic effects on cells through the formation of highly toxic hydroxyl radicals. These radicals also react with iron or copper to form stable aldehydes (such as MDA) which are known to damage cell membranes (Maritim et al., Citation2003). The action of such ROS together with elevated cytosolic calcium causes rapid destruction of β-islets of Langerhans and acini (Mandlik et al., Citation2008). Such cellular events lead to hyperglycemia that further triggers increased formation of free radicals which subsequently generates ROS and finally manifests in oxidative stress. Oxidative stress affects adversely endogenous system, major signaling pathways and cellular mechanisms, including elevation of pro-inflammatory markers like tumor necrosis factor-alpha (TNF-α), interleukin (IL-6), and C-reactive proteins (CRP), which are directly associated with insulin resistance type-II diabetes mellitus (Dominiczak, 2003; Niehoff et al., Citation2007; Sjöholm & Nyström, Citation2005).
In the present study, elevated serum TNF-α and IL-6 (in alloxan diabetic rats) were significantly reduced with the treatment of HEF dose dependently, thereby indicating its selective effect on cellular mediator involved in insulin resistance type-II diabetes. Oxidative stress induced by hyperglycemia in diabetes is a major causative factor for the development and progression of diabetic microvascular complications (Evans et al., Citation2002). Antioxidants are capable of scavenging, neutralizing free radicals, and also impede free radicals actions at different stages. Thus, endogenous antioxidants are involved in prevention, interception, and repair of oxidative status/system. Free radical-mediated lipid peroxidation has been proposed to be a major event in cancer, diabetes, rheumatoid arthritis, drug- associated toxicity, and aging process (Lowry et al., Citation1951). SOD catalyses dismutation of superoxide anions (the first product of O2 radicals to form H2O2) thereby diminishing the toxic effects of such radicals or other free radicals derived from secondary reactions (Ahmed, Citation2005; Jakus, Citation2000; Wohaieb & Godin, Citation1987). It is also reported that the elevation of GSH and SOD may be a compensatory mechanism(s) during chronic diseases like diabetes, due to over production of free radicals causing oxidative stress (Hazem et al., Citation2007). In the present study, the depleted enzymatic and non-enzymatic anti-oxidants of diabetic rats were restored significantly with the treatment of HEF. Such effects may be mediated through the active phytoconstituents present in HEF, like 4-hydroxy isoleucine, diosgenin, orientin, quercetin, etc., either by scavenging or quenching or neutralizing the free radicals or other ROS (Baig et al., Citation2012; Punitha et al., Citation2005). Our findings on up-regulation of SOD and GSH in diabetic rats are consistent with the documented reports.
The involvement of hepatic and extra hepatic mechanisms, namely, decrease glycogenolysis, increased glycogenesis in liver, and enhanced transport of blood glucose to peripheral tissues, is clearly reflected by the stimulatory effect on glucose uptake by diaphragm and muscle (Fernandes et al., Citation2007; Lenzen, Citation2008) and regeneration of β-islets of Langerhans (histologically observed) by HEF treatment have been demonstrated experimentally. These findings suggest the intrinsic ability of HEF to stimulate sufficient production of insulin there by helping in repair and/or regeneration of β-islets and acini cells to enhance utilization of glucose by the peripheral tissues.
During the progression of diabetes, the access of glucose in blood reacts with hemoglobin to form glycated hemoglobin complex (Mohammadi & Naik, Citation2008; Senthilkumar et al., Citation2006). In our present study, glycosylated hemoglobin was elevated to nearly two times the above normal in diabetic rats. Treatment with HEF reduced the elevated glycated hemoglobin, elevated plasma glucose, and increased body weight both in normal as well as diabetic rats, which seem to be an ideal property of a hypoglycemic drug. The other important findings of the present experiment was combined treatment of HEF with lower dose of glimepiride enhanced the hypoglycemic and other effects of glimepiride, thereby suggesting that HEF can be used as an adjuvant with other clinically effective antidiabetic drugs.
The pancreas and integrated gastrointestinal gland consist of exocrine and endocrine parts. Its endocrine part is largely included the islets of Langerhans and many other discrete cells (approximately 1–2% of glandular mass) scattered among the exocrine part. The co-existence of these two structurally and functionally different parts has postulated the direct influence of exocrine hormones on the physiology of acini cells. Acini cells produce amylase and lipase and many other hydrolases, which are normally channeled into the gastro-intestinal tract as a part of pancreatic juice for the digestion of polysaccharides and lipids (Bolender, Citation1974; Guyton & Hall, Citation2000; Szkudelski, Citation2001). Alloxan induces morphological changes in acini cells (necrosis, inflammation, and alpha cell neogenesis) and beta cell neogenesis of pancreas. Interestingly, the relation between insulin and glucagon concentration in fluid and other antagonistic action also likely play a major role in pancreatic exocrine functions. However, insulin is a main factor for the stimulation of exocrine activity, whilst glucagon is inhibitory, not only in the process of synthesis but also in the secretion of pancreatic enzymes (Harding et al., Citation2001; Taniyama et al., Citation1999).
Damage to acini cells through the oxidative stress generated by alloxan results in the leakage of amylase and lipase into blood circulation, and thus, raises their serum concentration as observed in diabetic control rats (Akpan et al., Citation2012). Earlier studies have reported a decrease (at early phase) and increase (late phase) in rabbits sera treated with alloxan and attributed to impaired pancreatic exocrine secretion due to decreased stimulatory action (Hardt et al., Citation2000; Shimizu et al., Citation2000) and increased release of enzyme from cellular compartment caused by increased destruction of pancreatic tissue (Burski et al., Citation2004). In the present experiments, there was a dose-related reduction in serum amylase and lipase in diabetic rats with HEF. However, normal rats treated with HEF/glimepiride did not alter serum enzymes as well as plasma glucose. The earlier reports demonstrated that pancreatic amylase and lipase were reduced in streptozotocin-induced diabetic rats (Aughsteen & Mohammed, Citation2002). Hence considering earlier reports and our findings, it is presumed that the reduction of serum enzymes in vivo following HEF treatment may not be associated with the inhibitory activity of extract but rather on its ability to stabilize acini cell membrane, thereby reducing the release of enzymes into circulation. It is possible that its antidiabetic activity may be related to increased repair of damaged beta cells and acini cells and regeneration of new ones to increase numbers (histologically seen) as wells as the functioning of β-islets for the improved synthesis of insulin, that may improve glucose utilization process. It was also observed that increased body weight of normal rats with HEF treatment as compared with untreated rats. Hence, it is likely that some phytoconstituents of HEF, either independently or together, may exert strong antioxidant and/or stimulate the actions of growth factors, which may be responsible for enhancing the repairing and regeneration of damaged pancreatic tissues.
Conclusion
The present study demonstrated that HEF exerts promising antidiabetic activity in alloxan diabetic rats. The observed antidiabetic activity is mediated by extra hepatic mechanisms via regeneration of β-islets of Langerhans. These effects may largely be achieved through inhibition of increased pro-inflammatory mediators, amelioration of oxidative stress induced by alloxan by the presence of phytoconstituents, causing hypoglycemic effects. The restoration of enzymes release may not be associated with hypoglycemic effect but may be directly related to acini cell membrane stabilization.
Acknowledgements
Authors are thankful to Prof. M. N. Navale, President (Sinhgad Technical Education Society, Pune) and Dr. S. B. Bhise, Principal (Sinhgad Institute of Pharmaceutical Sciences, Lonavala), for providing facilities and encouragement during the execution of research project.
Declaration of interest
All the authors report no declaration of interest.
References
- Ahmed RG. (2005). The physiological and biochemical effects of diabetes on the balance between oxidative stress and antioxidant defense system. Med J Islam World Acad Sci 15:31–42
- Akpan HD, Ekaidem IS, Usoh IF, et al. (2012). Effect of aqueous extract of Azadirachta indica (Neem) leaves on some indices of pancreatic function in alloxan-induced diabetic Wistar rats. Pharmacologia 3:420–5
- Ali L, Azad Khan AK, Hassan Z, et al. (1995). Characterization of the hypoglycemic effects of Trigonella foenum graecum seed. Planta Med 61:358–60
- Ashcroft T, Simpson JM, Timbrell V. (1988). Simple method of estimating severity of pulmonary fibrosis on a numerical scale. J Clin Pathol 41:467–70
- Aughsteen AA, Mohammed FI. (2002). Insulin enhances amylase and lipase activity in the pancreas of streptozotocin-diabetic rats. An in vivo study. Saudi Med J 23:838–44
- Baig MA, Gawali VB, Patil RR, Naik SR. (2012). Protective effect of herbomineral formulation (Dolabi) on early diabetic nephropathy in streptozotocin-induced diabetic rats. J Nat Med 66:500–9
- Bolender RP. (1974). Stereological analysis of guinea-pig pancreas. 1 Analytic model and quantitative description of nonstimulate dexocrine cells. J Cell Biol 61:269–87
- Brandsteterova E, Ziakova-Caniova A. (2002). Encyclopedia of Chromatography. New York: Marcel Decker
- Buetler E, Duron O, Kelly BM. (1963). Improved method for determination of blood glutathione. J Lab Clin Med 61:882–8
- Burski K, Ueland T, Maciejewski R. (2004). Serum amylase activity disorders in the course of experimental diabetes in rabbits. Vet Med Czech 49:197–200
- Carroll VN, Longley RW, Roe JH. (1956). Determination of glycogen in liver and muscle by use of anthrone reagent. J Biol Chem 220:583–93
- Cooperstein SJ, Watkins D. (1981). Action of toxic drugs on islet cells. In: Cooperstein SJ, Watkins D, eds. The Islets of Langerhans. New York: Academic Press, 387–425
- Devasena T, Menon VP. (2002). Enhancement of circulatory antioxidants by fenugreek during 1,2-dimethylhydrazine-induced rat colon carcinogenesis. J Biochem Mol Biol Biophys 6:289–92
- Dominiczak MH. (2003). Obesity, glucose intolerance and diabetes and their links to cardiovascular disease. Implications for laboratory medicine. Clin Chem Lab Med 41:1266–78
- Etuk EU. (2010). Animals models for studying diabetes mellitus. Agric Biol J N Am 1:130–4
- Evans JL, Goldfine ID, Maddux B, et al. (2002). Oxidative stress and stress-activated signaling pathways: A unifying hypothesis of type II diabetes. Endocr Rev 23:599–622
- Fernandes NPS, Lagishetty CV, Panda VS, Naik SR. (2007). An experimental evaluation of the antidiabetic and anti-lipidemic properties of a standardized Momordica charantia fruit extract. BMC Compliment Altern Med 7:29–36
- Guest CB, Park MJ, Johnson DR, Freund GG. (2008). The implication of proinflammatory cytokines in type 2 diabetes. Front Biosci 1:5187–94
- Gupta A, Gupta R, Lal B. (2001). Effect of Trigonella foenum-graecum seeds on glucaemic control and insulin resistance in type 2 diabetes mellitus. J Assoc Physicians India 49:1057–61
- Guyton AC, Hall JE. (2000). A Textbook of Medical Physiology. 10th ed., Philadelphia (PA): Saunders Publishers, 322–77
- Hamadi SA. (2012). Effect of trigonelline and ethanol extract of Iraqi Fenugreek seeds on oxidative stress in alloxan diabetic rabbits. J Assoc Arab Uni Basic Appl Sci 12:23–6
- Harding HP, Zeng H, Zhang Y, et al. (2001). Diabetes mellitus and exocrine pancreatic dysfunction in perk–/– mice reveals a role for translational control in secretory cell survival. Mol Cell 7:1153–63
- Hardt PD, Krauss A, Bretz L, et al. (2000). Pancreatic exocrine function in patients with type 1 and type 2 diabetes mellitus. Acta Diabetol 37:105–10
- Hays NP, Galassetti PR, Coker RH. (2008). Prevention and treatment of type II diabetes: Current role of lifestyle, natural product, and pharmacological interventions. Pharmacol Ther 118:181–91
- Hazem H, Kataya H, AlaaEldin A. (2007). Red cabbage (Brassica oleracea) ameliorates diabetic nephropathy in rats. eCAM 11:1–7
- Jakus V. (2000). The role of free radicals, oxidative stress and antioxidant systems in diabetic vascular disease. Bratisl Lek Listy 101:541–51
- Jung W, Trogem B, Klein G, et al. (1989). Evaluation of a new assay for pancreatic amylase: Performance characteristics and estimation of reference intervals. Clin Biochem 22:109–14
- Khandelwal KR. (2009). Preliminary Phytochemical Screening. Practical Pharmacognosy Techniques and Experiments. 9th ed. Pune, India: Nirali Prakashan, 149–57
- Kokate CK. (2008). Practical Pharmacognosy. 4th ed. New Delhi, India: Vallabh Prakashan, 107–11
- Lenzen S. (2008). The mechanisms of alloxan-and streptozotocin-induced diabetes. Diabetologia 51:216–26
- Lott JA, Patell ST, Sawhney AK, et al. (1986). Assays of serum lipase: Analytical and clinical considerations. Clin Chem 32:1290–302
- Lowry OH, Rosenbrough NJ, Farr AL, Randall RJ. (1951). Protein measurement with the Folin phenol reagent. J Biol Chem 193:265–75
- Mandlik RV, Desai SK, Naik SR, et al. (2008). Antidiabetic activity of polyherbal formulation (DRF/AY/5001). Indian J Exp Biol 46:599–606
- Maritim AC, Sanders RA, Watkins JB. (2003). Diabetes, oxidative stress and antioxidants: A review. J Biochem Mol Toxicol 17:24–38
- Martinello F, Soares SM, Franco JJ, et al. (2006). Hypolipidemic and antioxidant activities from Tamarindus indica L pulp fruit extract in hypercholesterolemic hamsters. Food Chem Toxicol 44:810–18
- Marx N, Froehlich J, Siam L, et al. (2003). Antidiabetic PPAR gamma-activator rosiglitazone reduces MMP-9 serum levels in type 2 diabetic patients with coronary artery disease. Arterioscler Thromb Vasc Biol 23:283–8
- Mishra HP, Fridovich I. (1972). Role of superoxide anion in auto-oxidation of epinephrine and a simple assay for superoxide dismutase. J Biol Chem 247:3170–5
- Mohammadi J, Naik PR. (2008). Evaluation of hypoglycemic effect of Morus alba in an animal model. Indian J Pharmacol 40:15–18
- Niehoff AG, van Haeften TW, Onland-Moret NC, et al. (2007). C-reactive protein is independently associated with glucose but not with insulin resistance in healthy men. Diabetes Care 30:1627–9
- Ohkawa H, Ohishi N, Yagi K. (1995). Assay for lipid peroxides in animal tissue by thiobarbituric acid reaction. Anal Biochem 95:351
- Parker KM, England JD, Costa JD, et al. (1981). Improved colorimetric assay for glycosylated hemoglobin. Clin Chem 27:669–72
- Punitha ISR, Rajendran K, Shirwaikar A, Shirwaikar A. (2005). Alcoholic stem extract of Coscinium fenestratum regulates carbohydrate metabolism and improves antioxidant status in streptozotocin–nicotinamide induced diabetic rats. eCAM 2:375–81
- Senthilkumar GP, Arulselvan D, Sathishkumar D, Subhramanian S. (2006). Antidiabetic activity of fruit of Terminalia chebula on streptozotocin induced diabetic rats. J Health Sci 52:283–91
- Shikarvar MS, Patil MB. (2010). Antidiabetic activity of Pongamia pinnata leaf extract in alloxan induced diabetic rats. Int J Ayurveda Res 1:199–204
- Shimizu K, Shiratori K, Hayashi N, et al. (2000). Effect of troglitazone on exocrine pancreas in rats with streptozotocin-induced diabetes mellitus. Pancreas 21:421–6
- Sicree R, Shaw J, Zimmet P. (2006). The global burden: Diabetes and impaired glucose tolerance. In: Gan D, ed. Diabetes Atlas, 3rd ed. Belgium: International Diabetes Federation, 1–100
- Sjöholm D, Nyström T. (2005). Endothelial inflammation in insulin resistance. Lancet 365:610–12
- Szkudelski T. (2001). The mechanism of alloxan and streptozotocin action in B cells of the rat pancreas. Physiol Res 50:537–46
- Taniyama H, Hirayama K, Kagawa Y, et al. (1999). Histopathological and immunohistochemical analysis of the endocrine and exocrine pancreas in twelve cattle with insulin-dependent diabetes mellitus (IDDM). J Vet Med Sci 61:803–10
- Trinder P. (1969). Determination of glucose in blood using glucose oxidase with an alternative oxygen acceptor. Ann Clin Biochem 6:24–7
- Wohaieb SA, Godin DV. (1987). Alterations in free radical tissue-defense mechanisms in streptozocin-induced diabetes in rat. Effects of insulin treatment. Diabetes 36:1014–18
- Yadav M, Tomar R, Prasad GBKS, et al. (2008). Complementary hypoglycemic and anti-hyperglycemic activity of various extracts of fenugreek seeds in rats. Asian J Biochem 3:182–7
- Zia T, Hasnain SN, Hasan SK. (2001). Evaluation of the oral hypoglycemic effect of Trigonella foenum-graecum L. (methi) in normal mice. J Ethnopharmacol 75:191–5