Abstract
Context: Polyketides are bioactive natural products with diverse bioactivities, and heterologous production of polyketides in easily engineered microbial hosts is preferred for the production of structurally diverse and the therapeutically active polyketides.
Objective: In this study, heterologous expression of the biosynthetic genes encoding type I polyketide synthases (PKS) involved in biosynthesis of fostriecin, a unique phosphate monoester polyketide antibiotic, was attempted.
Materials and methods: Fostriecin PKS (Fos-PKS) biosynthetic gene cluster in a total of 48.4 kb were cloned downstream of the act I promoter in two compatible Streptomyces vectors using Red/ET recombination. The co-expression plasmids were sequentially transferred into Streptomyces lividans and Streptomyces coelicolor. Active transcription of the polyketide genes was confirmed by reverse transcription PCR (RT-PCR) analysis, and the metabolites were detected using high-performance liquid chromatography (HPLC).
Results: The recombinant strains S. lividans TK24/p6-fosAB–p4-fosCDEF and S. coelicolor M512/p6-fosAB–p4-fosCDEF were obtained for heterologous expression in Streptomyces. Pigmentation was observed in the recombinant strains, whereas the control strain with empty vector displayed no change in pigment production. Active transcription of the polyketide genes was confirmed by RT-PCR analysis and subsequent sequencing.
Conclusion: The present study is the first attempt to overexpress Fos-PKS biosynthetic gene cluster in Streptomyces. More studies on heterologous expression of the fostriecin biosynthetic gene cluster would be beneficial for further understanding the mechanisms of its structural as well as the potential pharmaceutically effect.
Introduction
Fostriecin (also called CI-920, PD110,161) belongs to a class of novel phosphate ester natural products and has a potent cytotoxic activity (Lewy et al., Citation2002). Fostriecin was originally isolated from the fermentation broth of Streptomyces pulveraceus (Boghigian & Pfeifer, Citation2008). It is structurally related to cytostatin (Jung et al., Citation2008), leptomycin (Jang et al., Citation2003), phoslactomycin (Ghatge et al., Citation2006), and leustroducsins (Miyashita et al., Citation2007), which have antitumor, antibacterial, and antifungal activities. Currently, great attention is focused on fostriecin due to its function as a cell cycle inhibitor of type II topoisomerase (Topo II) (Maki et al., Citation2005). Previous studies mainly focused on the understanding of the biological properties of fostriecin, the biosynthesis, and chemical synthesis of analogs (Chavez & Jacobsen, Citation2001; Fujii et al., Citation2003; Gao & O'Doherty, Citation2010).
Fostriecin belongs to type I polyketide antibiotics (Smith & Tsai, Citation2007). The modularity of the scaffold biosynthesis of polyketides renders the possibility of creating novel antibiotics with optimized properties toward activity, production, and pharmacokinetics (Fujii, Citation2009; Kittendorf & Sherman, Citation2006). In the previous study, the biosynthetic gene cluster of fostriecin was identified in S. pulveraceus ATCC 31906 and several biosynthetic genes involved in the modification of polyketides were characterized (Kong et al., Citation2013). The complete sequence of the putative fostriecin biosynthetic gene cluster spans totally about 73 kb, which includes 21 relevant ORFs related to fostriecin biosynthesis. Genes encoding six modular type I polyketide synthases (PKS) (FosABCDEF) were identified in the gene cluster. This cluster included one loading module in fosA, one thioesterase domain (TE) in fosF, and nine extender modules which catalyze eight rounds of decarboxylative condensations deduced from the structure of fostriecin. The modules' arrangement of Fos-PKS biosynthetic gene cluster is very unusual, since the PKS genes are organized into two different positions in the gene cluster. Furthermore, there is a putative terminator between fosF and fosG which separates these two genes.
The individual role of the biosynthetic genes involved in fostriecin formation was investigated (Kong et al., Citation2013; Liu et al., Citation2013). Extensive efforts are attempted to delete the genes encoding modification enzymes; however, almost all mutants do not produce corresponding compounds with expected structures (Liu et al., Citation2013). Heterologous expression of natural product biosynthetic pathways is a strategy to generate novel analogs (Zhang et al., Citation2011). Different gene clusters which containing PKS and non-ribosomal peptide synthetases (NRPS) have been successfully expressed in heterologous hosts to produce novel analogs (Jiang et al., Citation2013; Müller, Citation2009; Perlova et al., Citation2009). Therefore, the production of fostriecin and its analogs through heterologous expression of the entire gene cluster is promising.
In this study, we attempted to reconstitute the 48.4 kb Fos-PKS biosynthetic gene cluster via Red/ET recombination to produce the polyketide precursor compound, which can be used for further structural modification to produce novel analogs. The two separate parts of the Fos-PKS genes (fosAB and fosCDEF) were inserted into the integrating and autonomously replicating vectors, respectively. The heterologous expression in Strpetomyces strains was further analyzed to explore the fostriecin biosynthetic pathway.
Materials and methods
Bacterial strains, plasmids, and culture conditions
Escherichia coli DH5α was used as the host for standard cloning experiments. Cfos-F1, Cfos-F2, Cfos-F3, and Cfos-F4 containing overlapping regions of the fostriecin biosynthetic gene cluster were used for reconstitution of the Fos-PKS genes. The E. coli strains harboring plasmids (or cosmids) were cultured in LB (Luria–Bertani) (Sambrook & Russell, Citation2001) medium either at 37 °C or at 30 °C (for Red/ET protein expression), with the appropriate antibiotic selection: ampicillin (100 mg/ml), apramycin (50 mg/ml), thiostrepton (10 mg/ml), kanamycin (50 mg/ml), tetracycline (12.5 mg/ml), chloramphenicol (25 mg/ml), and streptomycin (75 mg/ml). The Streptomyces strains in this study included S. pulveraceus ATCC 31906, S. lividans TK24, and S. coelicolor M512 (Δred D, Δact II-ORF4 SCP1−SCP2−). FST12 liquid medium (Kong et al., Citation2013) and MS (mannitol soya flour) medium (Kieser et al., Citation2000), which was supplemented with thiostrepton (10–20 mg/ml) and apramycin (50 mg/ml) for the selection of recombinant strains, were used for the Streptomyces strains growth and production. For fostriecin production, the cultures of Streptomyces strains were cultivated under standard conditions (28 °C, 180 rpm on a rotary shaker) and harvested after 4–6 d (Kong et al., Citation2013). Streptomyces strains were grown in yeast extract–malt extract (YEME) medium (Kieser et al., Citation2000) for genomic DNA isolation.
General molecular cloning
Construction of the co-expression vectors and cloning of the fostriecin biosynthetic gene cluster were performed with a standard Red/ET recombination (Zhang et al., Citation2000). Plasmid pSC101-BAD-γβα-tet containing genes encoding Redα and Redβ were introduced by transformation into E. coli SH996 (Heermann et al., Citation2008). After recombination, DNA isolated from clones was examined by restriction analysis and PCR. PCR products were analyzed by gel electrophoresis and further confirmed by DNA sequencing.
PCR reactions were performed with LA Taq or Pyrobest DNA polymerases (TaKaRa, Shiga, Japan). The general conditions included an initial denaturation at 98 °C for 2 min, followed by 30 cycles with 95 °C for 30 s, annealing temperature (an optimal annealing temperature was chosen for each primer pair) for 30 s, and 72 °C for 1–2 min (depending on the length of the amplified product). The conditions used in the generation of plasmid constructs were optimized for the amplification of GC-rich Streptomyces DNA by the addition of 10% DMSO (dimethyl sulfoxide) to each sample and raising the annealing temperature to 65 °C. The oligonucleotide primers are listed in .
Table 1. Oligonucleotide primers used in this study.
Cloning of Fos-PKS biosynthetic gene cluster
To construct the Fos-PKS co-expression system of Streptomyces, the fosAB and fosCDEF genes were cloned downstream of the act I promoter in two compatible Streptomyces vectors (the integrating vector p126 and autonomously replicating vector p124) using Red/ET recombination.
In order to construct expression vector p6-fosAB by Red/ET recombination, the fosB gene from cosmid Cfos-F1 and fosA gene from cosmid Cfos-F3 were successively homologous recombined into the p126 vector. Meanwhile, the fosCDEF genes from cosmid Cfos-F2 were homologously recombined into the p124 linear vector to form the expression vector p4-fosCDEF ().
Figure 1. Strategy of Fos-PKS biosynthetic gene cluster cloning by Red/ET recombination and confirmation of constructed expression plasmids: (a) distribution of sequenced cosmids, organization of the fostriecin biosynthetic gene cluster, and strategy of Fos-PKS biosynthetic gene cluster cloning using Red/ET recombination. Each arrow represents an open reading frame (ORF). The direction of transcription and the relative sizes of ORFs are indicated; (b) maps of plasmids for the expression of Fos-PKS biosynthetic gene cluster in Streptomyces. The expression plasmid p6-fosAB containing fosAB and the expression plasmid p4-fosCDEF containing fosCDEF; (c) restriction analysis of the constructed plasmid p6-fosAB by digestion with Xho I. M: λ-Hind III digest Marker and DNA Marker III; p126, F1, and F3: vector p126, cosmid Cfos-F1, and Cfos-F3 digestion with Xho I for compared; 1, 2, 3 and 4: four correct constructs of plasmid p8-fosAB digestion with Xho I; (d) restriction analysis of the constructed plasmid p4-fosCDEF by digestion with Mlu I. M: λ-Hind III digest Marker and DNA Marker III; p124, and F2: vector p124 and cosmid Cfos-F2 digestion with Mlu I for compared; 1 and 2: two correct constructs of plasmid pM-fosCDEF digestion with Mlu I.
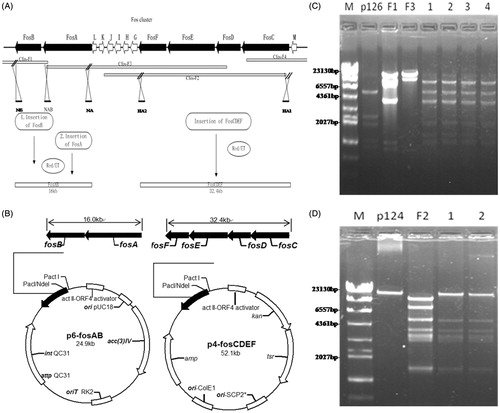
Transformation, expression, and analysis of gene expression and metabolites
Transformation of the plasmids p6-fosAB and p4-fosCDEF into S. lividans TK24 or S. coelicolor M512 protoplasts was performed according to the standard protocol (Kieser et al., Citation2000) with minor modifications, namely higher amount of protoplast and plasmids were used in the present study. The protoplast regeneration time was extended to achieve sufficient transformation efficiency. Streptomyces host strains with empty vectors (p124 and p126) were used as a control. All the successful transformants were confirmed by restriction enzyme digestions of the isolated plasmid, followed by sequencing.
TK24/p6-fosAB–p4-fosCDEF and M512/p6-fosAB–p4-fosCDEF were cultivated at 28 °C in yeast extract peptone dextrose (YPD) medium containing apramycin (50 mg/ml) and thiostrepton (10–20 mg/ml) for 24 h, and then the resulting seed culture was inoculated into the fermentation medium (FST12 liquid medium) at 20 °C (220 rpm) and harvested after 4–6 d. The fermentation broth was extracted and analyzed by high-performance liquid chromatography (HPLC) according to the previous study (Kong et al., Citation2013).
After the fermentation, the cells were harvested by centrifuge and washed with PBS buffer. RNA isolation and reverse transcript reactions were, respectively, performed with MINI RNA extraction kit (Sangon, Shanghai, China) and reverse transcriptase M-MLV (TaKaRa, Shiga, Japan) according to the manufacturer's protocol. PCR operation was performed according to the procedure previously described above, and the PCR products were further confirmed by DNA sequencing.
Results
The co-expression system of Streptomyces
Genes involved in fostriecin biosynthesis were located on four individual cosmids from a gene library of S. pulveraceus ATCC 31906, and the 48.4 kb Fos-PKS biosynthetic gene cluster were divided into two different regions: fosAB and fosCDEF. The fosAB genes which containing upstream region of the biosynthetic gene clusters with a loading module were located on cosmids Cfos-F1 and Cfos-F3. The fosCDEF genes that containing downstream region of the cluster with a TE were located on Cfos-F2 and Cfos-F4. The clone strategy is shown in .
Integrating vector p6-fosAB and replicating vector p4-fosCDEF () were constructed for co-expression of the Fos-PKS biosynthetic gene cluster in Streptomyces. The integrating vector p126 with phage ø C31 attachment region (att P) and integrase gene (int) as well as the gene encoding apramycin resistance, a derivative of pSET152, which contains the phage fd transcription terminator and genes encoding the act II-ORF IV activator is required for expression from the act I promoter originally from pRM5. The fosAB gene cluster was then cloned into the p126 downstream of the act I promoter resulting in p6-fosAB, and the correct constructs were analyzed by restriction endonucleases (). Plasmid p124 was an E. coli/Streptomyces shuttle vector with resistance markers of kanamycin, thiostrepton, and ampicillin. Plasmid p124 contains the act II-ORF IV activator, as well as the SCP2* and the ColE1 origins of replication. Plasmid p4-fosCDEF carried the fosCDEF gene cluster cloned downstream of the act I promoter on p124 ().
The constructed p6-fosAB and p4-fosCDEF, which harbor the Fos-PKS genes, were successively introduced into the host strains including S. lividans TK24 and S. coelicolor M512 by protoplast transformation, and TK24/p6-fosAB–p4-fosCDEF and M512/p6-fosAB–p4-fosCDEF were obtained for heterologous expression in Streptomyces.
Heterologous expression of Fos-PKS biosynthetic gene cluster
The recombinant strains TK24/p6-fosAB–p4-fosCDEF and M512/p6-fosAB–p4-fosCDEF were cultivated in the production medium and compared with strains containing empty vectors (TK24/p126–p124 and M512/p126–p124). When the growth of the strains containing the Fos-PKS biosynthetic gene cluster was investigated, we noticed that the cells grew well only until the 5th day after inoculation, i.e., until the stationary phase was reached. The cultures of TK24/p6-fosAB–p4-fosCDEF and M512/p6-fosAB–p4-fosCDEF, respectively, developed red and blue color after 3 d, while the control strains containing only the empty vectors (TK24/p126–p124 and M512/p126–p124) showed no change in color ().
Figure 2. Analysis of heterologous expression of Fos-PKS biosynthetic genes cluster in Streptomyces: (A) phenotype of the Streptomyces heterologous expression strains. TK24-V: S. lividans TK24/p126–p124 in FST12 liquid fermentation medium, TK24-G: S. lividans TK24/p6-fosAB–p4-fosCDEF in FST12 liquid fermentation medium, TK24-P: S. lividans TK24/p6-fosAB–p4-fosCDEF on MS medium; M512-V: S. coelicolor M512/p126–p124 in FST12 liquid fermentation medium, M512-G: S. coelicolor M512/p6-fosAB–p4-fosCDEF in FST12 liquid fermentation medium, M512-P: S. coelicolor M512/p6-fosAB–p4-fosCDEF on MS medium; (B) RT-PCR of S. coelicolor M512/p6-fosAB–p4-fosCDEF. A′–F′: the RNA as the template; A–F: the cDNA as the template.
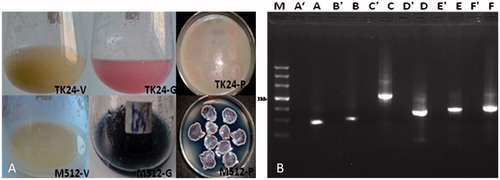
To evaluate the heterologous expression at the gene level of the M512/p6-fosAB–p4-fosCDEF co-expression system, RNA was isolated from the successful integration cluster and reversely transcripted to gain the cDNA. The cDNA containing internal fragments of the genes fosA, fosB, fosC, fosD, fosE, and fosF was used as a template for RT-PCR analysis. The amplification products of all the above-mentioned genes were detected using cDNA as the template, and no contamination of genomic DNA was confirmed using RNA as a template without the addition of reverse transcriptase (). Subsequently, the correct DNA sequences were also confirmed. These results demonstrated that the Fos-PKS genes were indeed expressed in the heterologous strains. However, when the extracts of heterologous strains were analyzed by HPLC/MS, no available data were obtained in the present study. Combined the results of this study and those in our recent work (Kong et al., Citation2013), we deduced that additional enzyme is needed in the release of polyketide precursor compound from Fos-PKS for fostriecin biosynthesis, and the details were discussed in the following text.
Discussion
Selection of the suitable host organism is of great importance to achieve significant production levels in the heterologous expression of a foreign gene cluster (Wenzel & Müller, Citation2005). The endogenous production of the antibiotics such as prodiginin (Red), actinorhodin (Act), and calcium-dependent antibiotic (CDA) in S. lividans and S. coelicolor might hamper the simultaneous biosynthesis and detection of a desired new compound. Therefore, S. coelicolor M512, in which Red and Act are not produced (Eustáquio et al., Citation2005), was also used as a heterologous host. Only polyketide precursor analog of fostriecin was detected in S. coelicolor M512 transformant (data not shown), although the results of RT-PCR were positive. Extensive efforts have been made to improve the secondary metabolite formation in the heterologous hosts, including trying different cultivation conditions, e.g. diverse media and temperatures, as well as supplementation with the putative biosynthetic precursor (sodium propionate), but no difference was observed in HPLC analysis. The entire 48.4 kb Fos-PKS biosynthetic genes’ cluster was also reconstituted on two compatible vectors and overexpressed in E. coli BAP1 (Pfeifer et al., Citation2001). However, there was no corresponding compound came from the extracts of the E. coli heterologous expression strain (data not shown). These results made us recheck the putative pathway for fostriecin biosynthesis.
General PKS chain elongation is terminated or cycled to form a lactone ring that is liberated by the TE enzyme. However, in special cases of phoslactomycin and leptomycin biosyntheses, the formation of an unsaturated six-membered lactone was demonstrated. Just like the PKS genes in the biosynthetic gene clusters of phoslactomycin and leptomycin (the DH domain in the last module is absent in Fos-PKS) (Khosla et al., Citation1999). The isolation of a series of malonylated compounds including malonylated polyketide precursor compound described in our recent study (Kong et al., Citation2013) strongly indicated that malonic acid moiety was linked to the PKS chain during PKS elongation; most likely, it precedes the release of the full length polyketide intermediate from Fos-PKS. Based on the results presented in this study, a biosynthesis pathway featuring specific chemistry for unsaturated six-membered lactone was proposed. A modification enzyme must function besides the Fos-PKS to add malonic acid moiety to fostriecin polyketide precursor during PKS elongation, then the malonylated polyketide precursor compound was liberated from PKS by the TE enzyme ().
To search for other enzyme participating in the release of polyketide precursor compound from Fos-PKS, FosM was identified as a NAD-dependent epimerase/dehydratase family protein, which showed an up to 42% sequence similarity to PlmT2 involved in the phoslactomycin biosynthesis (Palaniappan et al., Citation2003). The enzyme of FosM was established to facilitate the formation of cis-2, 3-double bond even if the targeting compound M-PLM B could be transferred into the PLM B without the function of PlmT2 (Palaniappan et al., Citation2008). We proposed that FosM might accelerate the elimination of the malonic acid moiety from the six-member ring and meanwhile forming the cis-2, 3-double bond, as well as that a similar process of malonylation and decarboxylative elimination might occur in fostriecin biosynthesis similar to phoslactmycin biosynthesis. At the same time, a series of malonylated compounds (Kong et al., Citation2013) indicate that malonylation was an efficient process and occurs in the early stage, following the post-PKS modification steps in fostriecin biosynthesis and providing the primary basis for following the post-PKS modification pathway in fostriecin biosynthesis (). Future efforts to understand the enzyme that adds malonic acid moiety to fostriecin polyketide precursor in the fostriecin biosynthetic gene cluster would contribute to the co-expression with the Fos-PKS biosynthetic gene cluster to gain the heterologous production. There were also some limitations in the present study, such as poor extraction and conversion efficiency .
Figure 3. Proposed post-PKS modification in a fostriecin polyketide biosynthetic pathway. ACP, acyl carrier protein; TE, thioesterase domain; “a”, FosM might play a role here.
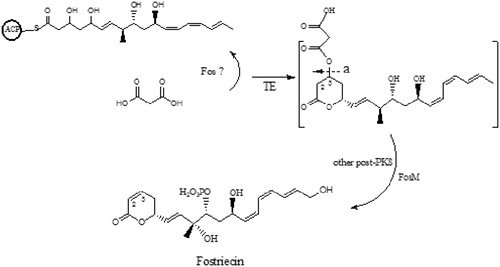
In conclusion, we proposed that it is necessary that a specific enzyme functioned to add malonic acid moiety to the fostriecin polyketide precursor during Fos-PKS elongation before the malonylated polyketide precursor compound was liberated from Fos-PKS by the TE enzyme. The current study is the first attempt to overexpress Fos-PKS biosynthetic gene cluster in Streptomyces, and more studies on heterologous expression of the fostriecin biosynthetic gene cluster will provide new opportunities for further understanding the mechanisms that build these structurally unique and pharmaceutically important agents.
Declaration of interest
The authors report no declarations of interest. This study was supported by National Natural Science Funds for Distinguished Young Scholar 30688003 to L. T.
References
- Boghigian BA, Pfeifer BA. (2008). Current status, strategies, and potential for the metabolic engineering of heterologous polyketides in Escherichia coli. Biotech Lett 30:1323–30
- Chavez DE, Jacobsen EN. (2001). Total synthesis of fostriecin (CI-920). Angew Chem 113:3779–82
- Eustáquio AS, Gust B, Galm U, et al. (2005). Heterologous expression of novobiocin and clorobiocin biosynthetic gene clusters. Appl Environ Microbiol 71:2452–9
- Fujii I. (2009). Heterologous expression systems for polyketide synthases. Nat Prod Rep 26:155–69
- Fujii K, Maki K, Kanai M, Shibasaki M. (2003). Formal catalytic asymmetric total synthesis of fostriecin. Org Lett 5:733–6
- Gao D, O'Doherty GA. (2010). Total synthesis of fostriecin: Via a regio-and stereoselective polyene hydration, oxidation and hydroboration sequence. Org Lett 12:3752–5
- Ghatge M, Palaniappan N, Das Choudhuri S, Reynolds K. (2006). Genetic manipulation of the biosynthetic process leading to phoslactomycins, potent protein phosphatase 2A inhibitors. J Ind Microbiol Biotechnol 33:589–99
- Heermann R, Zeppenfeld T, Jung K. (2008). Simple generation of site-directed point mutations in the Escherichia coli chromosome using Red®/ET® recombination. Microb Cell Fact 7:14
- Jang B-C, Muñoz-Najar U, Paik J-H, et al. (2003). Leptomycin B, an inhibitor of the nuclear export receptor CRM1, inhibits COX-2 expression. J Biol Chem 278:2773–6
- Jiang M, Zhang H, Pfeifer B. (2013). The logic, experimental steps, and potential of heterologous natural product biosynthesis featuring the complex antibiotic erythromycin A produced through E. coli. J Visualized Exp: JoVE 71:e4346
- Jung WH, Guyenne S, Riesco-Fagundo C, et al. (2008). Confirmation of the stereostructure of (+)-cytostatin by fluorous mixture synthesis of four candidate stereoisomers. Angew Chem 120:1146–9
- Khosla C, Gokhale RS, Jacobsen JR, Cane DE. (1999). Tolerance and specificity of polyketide synthases. Annu Rev Biochem 68:219–53
- Kieser T, Bibb MJ, Buttner MJ, et al. (2000). Practical Streptomyces Genetics. UK: John Innes Foundation Norwich
- Kittendorf JD, Sherman DH. (2006). Developing tools for engineering hybrid polyketide synthetic pathways. Curr Opin Biotechnol 17:597–605
- Kong R, Liu X, Su C, et al. (2013). Elucidation of the biosynthetic gene cluster and the post-PKS modification mechanism for fostriecin in Streptomyces pulveraceus. Chem Biol 20:45–54
- Lewy D, Soenen D, Boger D. (2002). Fostriecin: Chemistry and biology. Curr Med Chem 9:2005–32
- Liu X-j, Kong R-x, Niu M-s, et al. (2013). Identification of the post-polyketide synthase modification enzymes for fostriecin biosynthesis in Streptomyces pulveraceus. J Nat Prod 76:524–9
- Maki K, Motoki R, Fujii K, et al. (2005). Catalyst-controlled asymmetric synthesis of fostriecin and 8-epi-fostriecin. J Am Chem Soc 127:17111–17
- Miyashita K, Tsunemi T, Hosokawa T, et al. (2007). Total synthesis of leustroducsin B via a convergent route. Tetrahedron Lett 48:3829–33
- Müller R. (2009). Biosynthesis and heterologous production of epothilones. The Epothilones: An Outstanding Family of Anti-Tumor Agents. Vienna: Springer
- Palaniappan N, Alhamadsheh MM, Reynolds KA. (2008). cis-Δ2, 3-Double bond of phoslactomycins is generated by a post-PKS tailoring enzyme. J Am Chem Soc 130:12236–7
- Palaniappan N, Kim BS, Sekiyama Y, et al. (2003). Enhancement and selective production of phoslactomycin B, a protein phosphatase II a inhibitor, through identification and engineering of the corresponding biosynthetic gene cluster. J Biol Chem 278:35552–7
- Perlova O, Gerth K, Kuhlmann S, et al. (2009). Novel expression hosts for complex secondary metabolite megasynthetases: Production of myxochromide in the thermopilic isolate corallococcus macrosporus GT-2. Microb Cell Fact 8:1
- Pfeifer BA, Admiraal SJ, Gramajo H, et al. (2001). Biosynthesis of complex polyketides in a metabolically engineered strain of E. coli. Science 291:1790–2
- Sambrook J, Russell DW. (2001). Molecular Cloning: A Laboratory Manual. Cold Spring Harbor: CSHL Press
- Smith S, Tsai S-C. (2007). The type I fatty acid and polyketide synthases: A tale of two megasynthases. Nat Prod Rep 24:1041–72
- Wenzel SC, Müller R. (2005). Recent developments towards the heterologous expression of complex bacterial natural product biosynthetic pathways. Curr Opin Biotechnol 16:594–606
- Zhang H, Boghigian BA, Armando J, Pfeifer BA. (2011). Methods and options for the heterologous production of complex natural products. Nat Prod Rep 28:125–51
- Zhang Y, Muyrers JP, Testa G, Stewart AF. (2000). DNA cloning by homologous recombination in Escherichia coli. Nat Biotechnol 18:1314–17