Abstract
Context: Herb–drug interactions are a serious problem especially for drugs with a narrow therapeutic index, taking into consideration that herbal medicines are commonly used in various parts of the world.
Objective: The present study investigates the effect of fenugreek, garden cress, and black seed on the pharmacokinetics of theophylline in beagle dogs.
Materials and methods: Beagle dogs received theophylline (200 mg) orally and blood samples were withdrawn at different time intervals (0.33, 0.66, 1.0, 1.5, 2, 3, 4, 6, 8, 12, 24, and 30 h). After a suitable washout period, each herb was given orally at doses of 25, 7.5, and 2.5 g, twice daily for 7 d. On the eighth day, theophylline was re-administrated orally and blood samples were collected. Plasma concentrations of theophylline were determined using HPLC and pharmacokinetic parameters were calculated using a non-compartmental analysis.
Results: Treatment with fenugreek (25 g, orally) lead to a decrease in Cmax and AUC0–t of theophylline of about 28% (p < 0.05) and 22% (p < 0.05), respectively, with no significant changes in T1/2λ compared with the baseline values. Garden cress caused a decrease in Cmax to a lesser extent and delayed Tmax of theophylline (2.10 ± 0.24 h versus 3.40 ± 0.74 h), while AUC0–∞ increased by 37.44%. No significant effect was observed for the black seed treatment on theophylline disposition as measured by Cmax, Tmax, AUC0–∞, and CL/F.
Discussion and conclusion: The concurrent use of fenugreek or garden cress alters theophylline pharmacokinetic behavior in an animal model. This could represent a modulation in cytochrome P450 activity, which is responsible for theophylline metabolism in beagle dogs. Further confirmation of these results in humans will warrant changes in theophylline dosing before the co-administration of such herbs.
Introduction
Theophylline has been used to treat asthma and chronic obstructive pulmonary disease for many years (Ohnishi, Citation2000; Sullivan et al., Citation1994). Its oral absorption is almost complete with a peak plasma concentration generally achieved 2 h after administration (Page et al., Citation1998). Theophylline is characterized by a narrow therapeutic window of 5–20 µg/mL (Desoky et al., Citation1993; Qiu et al., Citation2007). In humans, it is extensively metabolized by cytochrome P450 (CYP) CYP1A2-mediated hepatic oxidation, predominantly to produce the metabolites 1,3-dimethyluric acid, 1-methyluric acid, and 3-methylxanthine, and to a lesser extent by CYP2E1 to 1,3-dimethyluric acid (Sarkar et al., Citation1992). Hence, it could be expected that the co-administration of drugs or herbal products that inhibit CYPlA2 activity could increase theophylline plasma concentration by reducing its metabolism, which may contribute to the emergence of adverse effects. In contrast, induction of CYP isoenzymes possibly will enhance theophylline clearance, leading to sub-therapeutic concentrations (Jan et al., Citation2005).
The global increase in the popularity of alternative medicines has raised renewed concerns regarding herb–drug interactions (Blanc et al., 2001). These interactions are especially important for drugs with narrow therapeutic indices, and may either be pharmacodynamic or pharmacokinetic in nature (Shi & Klotz, Citation2012; Tarirai et al., Citation2010). According to the World Health Organization (WHO), a significant percentage of the population living in developing countries relies on the traditional medicine for their primary health-care needs (Al-Faris, Citation2000; Mukherjee & Wahile, Citation2006; WHO, Citation2003).
Nigella sativa Linn. (Ranunculaceae), commonly known as black seed, is an annual herb that grows in countries bordering the Mediterranean Sea, as well as, in Pakistan and India (Gali-Muhtasib et al., Citation2006). The seeds have been used as a natural remedy over thousand years to promote health and treat diseases. For example, the oil of Nigella sativa is used as a traditional medicine for the treatment of arthritis, lung diseases, and hypercholesterolemia (Alkharfy et al., 2013b; Khader et al., Citation2009). Some important constituents of the seeds include thymoquinone, nigellone, and isoquinoline alkaloid (nigellimine N-oxide). Thymoquinone has been reported to be an effective antimicrobial and anthelmintic agent. It may also play a role in women's health, stimulating menstruation, and increasing milk flow (Ajayi et al., Citation2006).
Garden cress is the dried ripe seeds of Lepidium sativum Linn. (Cruciferae). The major constituents of Lepidium sativum are glucosinolates. Other constituents include ascorbic acid, cucurbitacins, and cardenolides. It has been used for respiratory disorders, vitamin C deficiency, constipation, poor immunity, and as a diuretic. The practitioners of Indian medicine consider its seeds useful in dysenteric diarrhea as well as in febrile and catarrhal infections (Gilani et al., 2013).
Fenugreek is the dried ripe seed of Trigonella foenum-graecum Linn. (Fabaceae). The constituents of fenugreek are simple alkaloids consisting mainly of trigonelline, choline, gentianine, carpaine, and saponine. It is used traditionally as a nutrient and in treating kidney ailments, diabetes, cellulitis, and tuberculosis. Fenugreek has also been utilized to reduce plasma cholesterol level and enhance blood anticoagulant activity (Basch et al., Citation2003; Sowmya & Rajyalakshmi, Citation1999). Enhancement of male libido has also been seen with the standardized fenugreek and its mineral formulation (Steels et al., Citation2011).
Given the fact that self-medication is a prevalent practice in many Asian cultures, more information about possible drug interactions with local herbal products is of critical importance. Therefore, effects of garden cress, black seed, and fenugreek on the disposition of theophylline are being explored by the current study.
Materials and methods
Chemicals and materials
Theophylline and hydroxyethyl theophylline (internal standard; I.S.) were obtained from Sigma-Aldrich (St. Louis, MO). High-performance liquid chromatography (HPLC) grade methanol and acetonitrile were purchased from Merck (Darmstadt, Germany). The tested herbs were procured in dry form from the local market and authenticated at the College of Pharmacy, King Saud University, Riyadh, Saudi Arabia. All other chemicals used were of the highest available commercial purity.
Animals and study design
Healthy male beagle dogs (n = 5) weighing 15–20 kg were used in the study after it was duly approved by the Experimental Animal Care Center, College of Pharmacy, King Saud University, Riyadh, Saudi Arabia (Reference no. C.P.R-153). Animals were maintained in accordance with the recommendations of the “Guide for the Care and Use of Laboratory Animals” approved by the center. They were allowed free water and food ad libitum during the study except that the chow was pulled 12 h prior to animals' dosing. After the overnight fasting, dogs were given dog food (30 g) alone to serve as controls. Fifteen minutes later, manually prepared 200 mg of theophylline hard gelatin capsule was administered orally to each dog. Blood samples (2 mL) were collected from a leg vein through an indwelling vein catheter into heparinized vacutainer tubes before theophylline administration and at 0.33, 0.66, 1.0, 1.5, 2, 3, 4, 6, 8, 12, 24, and 30 h after dosing. Plasma was then immediately separated by centrifugation of blood at 2500 g for 10 min and stored at −20 °C until analysis. In all experiments, equal volumes of normal saline were injected through the cannula to replace the fluid loss.
After a suitable washout period, animals were started on a regimen of the studied herb where fenugreek, garden cress, and black seed were given to various groups at doses of 25, 7.5, and 2.5 g, respectively. To achieve proper herbal treatment, seeds of the herbs were grounded and mixed with 30 g of dog food and administered twice daily for 7 d. On the morning of the last day and after an overnight fasting, the last dose of the herb was given and theophylline (200 mg orally) was re-administered 15 min later. The same sampling scheme was repeated as described above to determine theophylline plasma concentrations and evaluate the effect of herbal therapy on theophylline disposition in dogs.
Theophylline plasma assay
An integrated HPLC system (LC 2010C; Shimadzu Corporation, Kyoto, Japan) equipped with a quaternary pump (LC20AD), a degasser, an auto-sampler (CIL20A), a column oven, a UV detector, and a data analysis software was used to determine theophylline plasma concentrations. Drug separation was achieved by a C18 column (5 µm, 150 mm × 4.6 mm; Waters Corporation, Milford, MA) at 25 °C. The mobile phase was a mixture of HPLC water/acetonitrile (93:7, v/v) with a pH of 4.2 adjusted with glacial acetic acid (0.5 mL/L) and pumped at a flow rate of 1.0 mL/min. Detection was set at a wavelength of 272 nm.
Sample preparation
Plasma sample (0.2 mL) was pipetted into micro-centrifuge tubes, then 0.1 mL of I.S. working solution (250 µg/mL) was added and the mixture was vortex mixed for 30 s. Then, 700 µL of zinc sulfate solution (2%) was added for protein precipitation followed by vortex-mixing for 1 min. The sample was centrifuged at 3000 g for 15 min at 25 °C. The clear supernatant was transferred into injector vials and a 50 µL aliquot was injected into the chromatographic system.
Calibration and control samples
Standard stock solutions of theophylline (1 mg/mL) and I.S. (1 mg/mL) were prepared in methanol. The I.S. working solution (250 µg/mL) was prepared by diluting stock solution with a mobile phase. Working solutions of theophylline and I.S. (0.2 mL) were added to drug-free plasma (9.6 mL) to obtain final concentrations of 0.1, 0.25, 0.5, 1.0, 2.5, 5.0, 10.0 and 25.0 μg/mL. The quality control samples at three different concentrations were also prepared in a similar manner as the calibration standards. Spiked plasma calibration standards and quality control samples were stored at −20 °C until analyzed.
Pharmacokinetic analysis
Non-compartmental analysis was used to determine the pharmacokinetic parameters of theophylline after oral administration in the beagle dogs before and after herbal treatment. The maximum observed plasma concentration (Cmax) and the time to reach the maximum observed plasma concentration (Tmax) following oral administration were determined from the observed data. The area under the concentration versus time curve (AUC0–t) was calculated by the trapezoidal rule with extrapolation to infinity. AUC from time 0 to infinity (AUC0–∞) was calculated as the sum of AUC0–t and any residual area, which was computed as the concentration at the last time point divided the terminal rate constant. Volume of distribution at steady state (Vss) as (AUMC0–∞/AUC0–∞) × CL and total body clearance (CL) as dose/AUC0–∞. The theophylline apparent elimination rate constant (λz) was estimated by linear regression analysis of the terminal portion of the log concentration–time data. Theophylline apparent elimination half-life (T1/2λ) was calculated as ln2/λ. Pharmacokinetic analysis was performed using WinNonlin software (version 4.1, Pharsight Corporation, Palo Alto, CA).
Statistical analysis
Data are presented as the mean ± SEM Differences in pharmacokinetic parameters (e.g., CL/F, Vss, and AUC0–∞) of theophylline before and after treatment with the tested herbs were assessed by a paired t-test on log-transformed data. Statistical significance were assumed when p ≤ 0.05. All calculations were performed using GraphPad Prism version 3.00 for Windows (San Diego, CA).
Results
The analytical method was developed and validated in our laboratory. The peak area ratio of theophylline to I.S. in dog plasma was linear with respect to the analyte concentration over the range 0.1–25.0 μg/mL. The mean coefficient of determination (r2) for the standard curves was >0.99. The intra- and inter-day precision values for all QC samples were less than 5.0% and accuracies of 95–105%.
The estimated model-independent pharmacokinetic parameters of theophylline before and after fenugreek treatment are listed in and . The calculated Cmax in dogs was 16.45 ± 0.72 μg/mL and 11.92 ± 1.08 μg/mL (p = 0.0029), and AUC0–t was 186.03 ± 5.71 μg h/mL and 144.38 ± 7.79 μg h/mL (p = 0.0035), respectively. A trend of reduction was observed in the calculated oral clearance (CL/F) and apparent oral volume of distribution at steady state (Vss), which, however, did not reach statistical significance (; p > 0.05). These parameters have resulted in T1/2λ of theophylline of 8.13 h and 8.05 h.
Figure 1. Plasma concentration–time curves of theophylline following oral administration of 200 mg dose to male beagle dogs (n = 5) alone (control) and after fenugreek, garden cress, and black seed treatment.
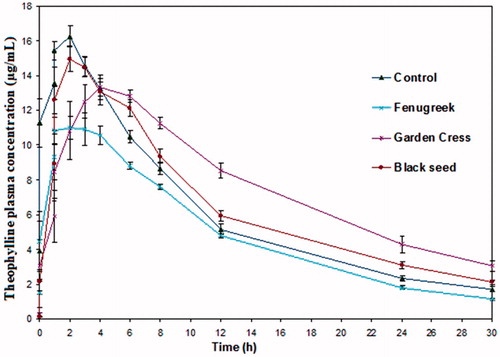
Table 1. Non-compartmental pharmacokinetic parameters of theophylline following an oral administration in beagle dogs before and after fenugreek treatment.
Plasma concentration–time curve and pharmacokinetic parameters of theophylline in dogs before and after garden cress are given in and . Theophylline Cmax and Tmax under garden cress treatment were 14.64 μg/mL and 3.40 h, respectively (p > 0.05). However, AUC0–∞ was significantly increased with herbal treatment (279.16 μg h/mL compared with 203.12 μg h/mL; p < 0.05). Similarly, the relative bioavailability of theophylline was enhanced by ∼37.44%.
Table 2. Non-compartmental pharmacokinetic parameters of theophylline following an oral administration in beagle dogs before and after garden cress treatment .
The comparison of plasma concentration–time profile and pharmacokinetic parameters of the theophylline after black seed treatment is shown in and . In this case, non-significant (p > 0.05) changes in Cmax and Tmax of theophylline were observed. T1/2λ of theophylline in the black seed treated group and controls were similar (). The extent of theophylline absorption (measured by AUC0–∞) was non-significantly (p > 0.05) increased when the drug was taken with black seed. In addition, there were no significant differences in the rests of the pharmacokinetic parameters including CL/F and Vss, relative to those of the control group.
Table 3. Non-compartmental pharmacokinetic parameters of theophylline following an oral administration in beagle dogs before and after black seed treatment.
Discussion
Traditional herbal medicines can act through a variety of mechanisms to alter the pharmacokinetic profile of the co-administered drug (Fugh-Berman, Citation2000). It is important to be aware of the interaction potency of commonly used herbal medicines in order to avoid a reduction in efficacy or an increase in the side-effects of concomitantly administered drugs (Tang et al., Citation2009). Further, the clinical consequence of interactions may be unforeseen side effects and non-compliance, and it is, therefore, of major importance for patient outcome (Landmark & Patsalos, Citation2008).
To best of our knowledge, this is the first report which explored the effect of some Asian traditional herbs on the disposition of theophylline after oral administration using an animal model. Our study has shown that all tested herbs interfere with the theophylline pharmacokinetic to varying degrees. Fenugreek treatment for 7 d caused a decrease in Cmax and AUC0-t. The T1/2λ whoever did not change significantly following fenugreek treatment and this can be explained by a similar simultaneous reduction in CL and Vss following the herb administration in beagle dogs. It is possible that one or more of fenugreek constituents interfere with theophylline tissue distribution, resulting in smaller Vss. In a recent study (Alkharfy et al., Citation2013a) fenugreek caused a significant reduction in the calculated Cmax and AUC0–t (∼ 50%) as compared with controls.
In our study, garden cress produced no significant effect on Cmax but caused an increase in Tmax. Theophylline AUC0–∞ also increased significantly following garden cress administration in beagle dogs, and the relative bioavailability of theophylline was 37.44% more when it was concurrently administered with the herbal product. Our results are in accordance with recently published data, where garden cress increased AUC0–t and AUC0–∞ of cyclosporine by about 40% (Al-Jenoobi et al., Citation2013). In contrast, black seed did not produce any remarkable effect on the disposition of theophylline in beagle dogs at the doses used in this study.
CYP1A2 is an important CYP enzyme subfamily, accounting for 13% of the total CYP enzyme in humans (Chien et al., Citation2010). In the current study, theophylline was a probe drug for CYP1A2, and showed some evidence of potential herbal interactions. Hence, many CYP1A2-metabolized drugs such as caffeine, theophylline, clozapine, and tacrine (Faber et al., Citation2005) could interact with fenugreek and garden cress. It would be interesting to identify herbs' specific constituent(s) responsible for these effects.
Conclusion
These findings have shown that fenugreek and garden cress have the potential to interfere with theophylline disposition. Therefore, it would be important to consider such types of interactions when dealing with individuals on theophylline therapy. In addition, these interactions should be further evaluated to assess their significance and clinical consequence in humans.
Declaration of interest
The authors report no declarations of interest. This study was supported by the Deanship of Scientific Research, King Saud University, Riyadh, Saudi Arabia (Grant number: DSR-AR-2-20).
References
- Ajayi IA, Oderinde RA, Kajogbola DO, Uponi JI. (2006). Oil content and fatty acid composition of some underutilized legumes from Nigeria. Food Chem 99:115–20
- Al-Faris EA. (2000). The pattern of alternative medicine use among patients attending health centres in a military community in Riyadh. J Fam Commun Med 7:17–25
- Al-Jenoobi FI, Al-Suwayeh SA, Muzaffar I, et al. (2013). Effects of Nigella sativa and Lepidium sativum on cyclosporine pharmacokinetics. Biomed Res Int 2013: Article ID 953520, 6 pages, doi:10.1155/2013/953520
- Alkharfy KM, Al-Jenoobi FI, Alam MA, et al. (2013b). Lepidium sativum but not Nigella sativa affects carbamazepine disposition in an animal model. Drug Metab Lett 7:47–51
- Alkharfy KM, Al-Jenoobi FI, Al-Mohizea AM, et al. (2013a). Effects of Lepidium sativum, Nigella sativa and Trigonella foenum-graceum on phenytoin pharmacokinetics in beagle dogs. Phytother Res 27:1800–4
- Basch E, Ulbricht C, Kuo G, et al. (2003). Therapeutic applications of fenugreek. Altern Med Rev 8:20–7
- Blanc PD, Trupin L, Earnest G, et al. (2001). Alternative therapies among adults with a reported diagnosis of asthma or rhinosinusitis: Data from a population-based survey. Chest 120:1461–7
- Chien CF, Wu YT, Lee WC, et al. (2010). Herb–drug interaction of Andrographis paniculata extract and andrographolide on the pharmacokinetics of theophylline in rats. Chem Biol Interact 30:184, 458–65
- Desoky E, Meinshausen J, Buhl K, et al. (1993). Generation of pharmacokinetic data during routine therapeutic drug monitoring: Bayesian approach vs pharmacokinetic studies. Ther Drug Monit 15:281–8
- Faber MS, Jetter A, Fuhr U. (2005). Assessment of CYP1A2 activity in clinical practice: Why, how, and when? Basic Clin Pharmacol Toxicol 97:125–34
- Fugh-Berman A. (2000). Herb–drug interaction. Lancet 355:134–8
- Gali-Muhtasib H, Roessner A, Schneider-Stock R. (2006). Thymoquinone: A promising anti-cancer drug from natural sources. Int J Biochem Cell Biol 38:1249–53
- Gilani AH, Rehman NU, Mehmood MH, Alkharfy KM. (2013). Species differences in the antidiarrheal and antispasmodic activities of Lepidium sativum and insight into underlying mechanisms. Phytother Res 27:1086–94
- Jan W, Lin L, Chen C, Tsai T. (2005). Herb–drug interaction of Evodia rutaecarpa extract on the pharmacokinetics of theophylline in rats. J Ethnopharmacol 102:440–5
- Khader M, Bresgen N, Eckl PM. (2009). In vitro toxicological properties of thymoquinone. Food Chem Toxicol 47:129–33
- Landmark CJ, Patsalos PN. (2008). Interactions between antiepileptic drugs and herbal medicines. Bol Latinoam Caribe Plant Med Aromaticas 7:108–18
- Mukherjee PK, Wahile A. (2006). Integrated approaches towards drug development from ayurveda and other Indian system of medicines. J Ethnopharmacol 103:25–35
- Ohnishi A. (2000). A review of clinical use of theophylline in acute asthma: Factors influencing kinetic disposition and drug interactions. Methods Find Exp Clin Pharmacol 22:253–8
- Page CP, Cotter T, Kilfeather S, et al. (1998). Effect of chronic theophylline treatment on the methacholine dose–response curve in allergic asthmatic subjects. Eur Respir J 12:24–9
- Qiu F, Wang G, ZhaoY, et al. (2007). Effect of danshen extract on pharmacokinetics of theophylline in healthy volunteers. Br J Clin Pharmacol 65:270–4
- Sarkar MA, Hunt C, Guzelian PS, Karnes HT. (1992). Characterization of human liver cytochromes P-450 involved in theophylline metabolism. Drug Metab Dispos 20:3l–7
- Shi S, Klotz U. (2012). Drug interactions with herbal medicines. Clin Pharmacokinet 51:77–104
- Sowmya P, Rajyalakshmi P. (1999). Hypocholesterolemic effect of germinated fenugreek seed in human subjects. Plant Food Hum Nutr 53:359–65
- Steels E, Rao A, Vitetta L. (2011). Physiological aspects of male libido enhanced by standardized Trigonella foenum-graecum extract and mineral formulation. Phytother Res 25:1294–300
- Sullivan P, Bekir S, Jaffear Z, et al. (1994). Anti-inflammatory effects of low-dose oral theophylline in atopic asthma. Lancet 343:1006–8
- Tang J, Song X, Zhu M, Zhang J. (2009). Study on the pharmacokinetics drug-drug interaction potential of Glycyrrhiza uralensis, a traditional Chinese medicine, with lidocaine in rats. Phytother Res 23:603–7
- Tarirai C, Viljoen AM, Hamman JH. (2010). Herb–drug pharmacokinetic interactions reviewed. Exp Opin Drug Metab Toxicol 6:1515–38
- WHO. (2003). Traditional Medicine. Fifty-Sixth World Health Assembly A56/18 March 31: 1–4. Available from: http://apps.who.int/gb/archive/pdf_files/WHA56/ea5618.pdf [last accessed 10 Feb 2014]