Abstract
Context: Careya arborea Roxb. (Lecythidaceae) has multiple applications in traditional medicine; it exhibits analgesic, antibacterial, anti-inflammatory, antiulcer, and protective effects. However, the effect of C. arborea on biochemical and immmunological inflammatory mediators has not been explored.
Objective: The present study investigates the anti-inflammatory potential of the methanol extract of C. arborea stem bark and further assesses its possible mechanism on the modulation of inflammatory biomarkers.
Materials and methods: Anti-inflammatory activity of C. arborea methanol extract (CAME) was evaluated (100 and 200 mg/kg, p.o) using indomethacin (10 mg/kg, p.o) as the standard drug in Wistar albino rats. Inflammation was induced by injecting 0.1 ml carrageenan (1% w/v) into the left hind paw. The anti-inflammatory mechanism was studied by measuring malondialdehyde (MDA), C-reactive protein (CRP), nitric oxide (NO), myeloperoxidase (MPO), TNF-α, and IL-1β levels in both control and treated groups. A protocol has also been established to quantify quercetin and betulinic acid content in CAME using HPTLC fingerprint.
Results: Careya arborea significantly (p < 0.001) decreased carrageenan-induced paw edema, showed a reduction of 48.87 and 65.53% at doses of 100 and 200 mg/kg, respectively. Moreover, CAME significantly decreased the MDA, CRP, NO, and MPO levels, elevated by carrageenan induced inflammation. CAME also markedly down-regulated serum TNF-α and IL-1β levels. These findings were further supported by the histological study. The content of quercetin and betulinic acid in CAME was found to be 0.177 and 3.14%, respectively.
Conclusion: Several mechanisms, including the inhibition of pro-inflammatory cytokines, enzymes and mediators release, appear to account for the anti-inflammatory potential of C. arborea.
Introduction
Acute inflammation is rapid, short-lived, and characterized by accumulation of fluid, plasma proteins, and leukocytes. At the site of inflammation, the injured vascular endothelial cells and the emigrated leukocytes release a large number of soluble mediators which modulate and maintain the inflammation (Rosenberg & Gallin, Citation1999). During inflammation, inflammatory cells, including neutrophils and macrophages, are activated. Activated macrophages stimulate the expression of a series of genes involved in host defense, which results in the release of different inflammatory mediators, pro-inflammatory cytokines, nitric oxide, cycloxygenase-2, etc. (Yoon et al., Citation2009).
However, when inflammation is uncontrolled, it leads to chronic inflammatory and autoimmune diseases (Rao et al., Citation2007). Extensive investigations within the last two decades revealed that most chronic illnesses including cancer, neurological, autoimmune, diabetes, and cardiovascular diseases are mediated through inflammation. Thus, suppressing inflammation has the potential to delay, prevent, and treat these diseases (Shah et al., Citation2006). Therefore, inhibition of inflammatory biomarkers could be a target to treat inflammatory disorders. Plants which have been used traditionally for inflammation have a potential to serve as sources of future drugs for treatment of various inflammatory diseases.
Careya arborea Roxb. (Lecythidaceae) is a large tree found throughout India in deciduous forests and grasslands (Kirtikar & Basu, Citation1975). Careya arborea is traditionally used in tumors, inflammation, anthelmintic, bronchitis, epileptic fits, astringents, antidote to snake-venom, skin disease, diarrhea, dysentery with bloody stools, dyspepsia, ulcer, tooth ache, and ear pain (Kirtikar & Basu, Citation1975; Sambathkumar et al., Citation2005). The plant has been extensively investigated and a number of chemical constituents from the stem bark, leaves, and seeds of the plant have previously been reported which includes triterpenoides (Das & Mahato, Citation1982; Mahati et al., Citation1973; Ramchandra & Sashtry, Citation1976), flavonoides (Basak et al., Citation1976; Gupta et al., Citation1975), sterols (Mahato & Datta, Citation1972), coumarin (Basak et al., Citation1976), saponins (Gedeon & Kinel, Citation1956), and tannins (Kulakkattolickal, Citation1987). The stem bark of C. arborea contains bioactive constituents including betulinic acid, betulin, and lupeol that have been reported as potential anti-inflammatory agents (Bentham & Hooker, Citation1985; Saldanha & Nicolson, Citation1976; Senthilkumar et al., Citation2008). The underlying mechanism of the anti-inflammatory activity of C. arborea is yet to be established. Further, no studies have yet investigated the anti-inflammatory potential of C. arborea on biochemical and inmmunological inflammatory mediators. The present study was focused on investigating the anti-inflammatory effect of C. arborea in carrageenan-induced acute inflammatory model and evaluation of inflammatory biomarkers.
Materials and methods
Plant material
The stem bark of C. arborea was collected from Kanyakumari district, Tamil Nadu, India, during the month of April 2012. The plant was identified by Mr. V. Chellandurai, Research officer (Botanist), FMR, AYUSH, and the specimen voucher (JH/PHCOG/20/01/2012) was deposited in the Department of Pharmacognosy and Phytochemistry, Faculty of Pharmacy, Jamia Hamdard, New Delhi 110062, India.
Drugs and chemicals
All HPLC grade solvents were purchased from Merck (Darmstadt, Germany). Quercetin, betulinic acid, γ-carrageenan, indomethacin, thiobarbituric acid, trichloroacetic acid, sulfanilamide, N-1-napthylethylenediaminedihydrochloride, hexadecyltrimethylammonium bromide, o-dianisidine hydrochloride, and other chemicals and reagents were purchased from Sigma-Aldrich (St. Louis, MO). Rat CRP ELISA assay kit was purchased from Immunology Consultants Laboratory, Inc. (Portland, OR). Rat TNF-α and IL-1β ELISA assay kits were purchased from RayBiotech Inc. (Norcross, GA).
Animals
All the experiments were carried out using 8–10 weeks old, Wistar rats weighing about 180–220 g, procured from the Central Animal House, Hamdard University, New Delhi, India. The animals had free access to standard laboratory food and water ad libitum, and they were housed in a natural light–dark cycle (12 h each). The animals were acclimatized to the laboratory conditions for at least 7 d before anti-inflammatory experiments. The experimental protocol was approved by the Institutional Animal Ethical Committee (754/CPCSEA, 2011) and the care of laboratory animal was taken as per the guidelines of the Committee for the Purpose of Control and Supervision of Experiments on Animals (CPCSEA).
Preparation of plant extract
The fresh stem bark of C. arborea was shade dried and powdered using the electric homogenizer. The powdered material was extracted with 1.5 l methanol for 8 h at 40 °C by using the Soxhlet apparatus. The solvent was removed at the reduced pressure with the help of the rotary vacuum evaporator to yield dark brown residue (65 g, 21.67%). The solid C. arborea methanol extract (CAME) was stored in a refrigerator and reconstituted later for various studies.
Phytochemical screening of major metabolites
CAME was subjected to preliminary phytochemical analysis with reference to the standard protocol for the detection of various constituents (Kokate et al., Citation2005).
Quantification of quercetin and betulinic acid by HPTLC
Preparation of sample and standards
Sample solution was prepared by dissolving 30 mg of CAME in methanol and making up the volume to 10 ml to get the concentration of 3 mg/ml. Stock solutions of quercetin and betulinic acid were prepared separately by dissolving 10 mg of accurately weighed in methanol and making the volume up to 10 ml with methanol to get the final concentration of 1 mg/ml. Sample and standards were filtered using a 0.22-μ membrane filter. To quantify quercetin and betulinic acid, 5 μl/spot of sample solution was applied on separate TLC plates.
Chromatographic conditions
HPTLC study was carried out following the methods of Harborne (Citation1998) and Wagner et al. (Citation1996). For quercetin and betulinic acid, toluene:ethyl acetate:formic acid:methanol (6:3:0.2:0.4) and toluene:acetone:formic acid (2.5:0.5:0.02) mobile phases were used, respectively.
For the present study, CAMAG HPTLC system equipped with Linomat V applicator, TLC scanner 3, controlled by WinCATS-4 software was used. The standards and sample were spotted in the form of bands of width 5 mm with a CAMAG microliter syringe on pre-coated silica gel plate 60F-254 [20 cm × 10 cm with 0.2 mm thickness (Merck KGaA, Darmstadt, Germany)] using a CAMAG Linomat IV (Muttenz, Switzerland). The plates were pre-washed by methanol and activated at 60 °C for 5 min prior to chromatography. The standard and sample-loaded plate was kept in the TLC twin trough developing chamber (after saturating with solvent vapor) with respective mobile phase up to 80 mm. The optimized chamber saturation time for the mobile phase was 30 min at room temperature (25 ± 2 °C). The developed plate was dried by hot air to evaporate solvents from the plate. Finally the quercetin-developed plate was scanned at 366 nm and post-chromatographic derivatization of betulinic acid was carried out in anisaldehyde and sulfuric acid followed by heating at 110 °C for 3 min and scanning at 550 nm. Densitometric scanning was performed on CAMAG TLC scanner and operated by WinCATS software.
Calibration curves for standards
The stock solutions of standards were diluted with methanol to obtain a concentration of 10–120 ng/spot for quercetin and 100–600 ng/spot for betulinic acid. Standards were applied on a separate TLC plate using the Linomat V applicator for preparing the calibration curve of peak area versus concentration. The regression equation for quercetin was y = 830.960 + 55.933x (co-relation coefficient, r2 = 0.99108) and for betulinic acid was y = −341.1 + 4.359x (co-relation coefficient, r2 = 0.97353).
Carrageenan induced paw edema
To evaluate the effect of the CAME on carrageenan-induced inflammation model in rats, edema was induced by subplantar injection of 0.1 ml of freshly prepared 1% w/v carrageenan into the left hind paw. The animals were divided into five groups, each consisting of six animals. CAME and indomethacin were suspended in normal saline and administered orally to animals. Group I rats received only normal saline, 1 ml/kg, which served as normal control. Group II rats (1 ml/kg normal saline + 0.1 ml of 1% w/v carrageenan) were served as carrageenan control. Group III rats (10 mg/kg indomethacin + 0.1 ml of 1% w/v carrageenan) were administered indomethacin and served as standard control to compare the efficacy of CAME. Group IV (100 mg/kg CAME + 0.1 ml of 1% w/v carrageenan) and group V rats (200 mg/kg CAME + 0.1 ml of 1% w/v carrageenan) were treated with CAME. The test drugs/vehicle were administered 1 h before injecting carrageenan. Paw volume was measured prior to injection of carrageenan (0 h) and then at predetermined intervals (1, 2, 3, 4, and 5 h after injecting carrageenan) by using plethysmometer, Ugo Basile, Comerio VA, Italy (Winter et al., Citation1962). The percentage changes in paw volume were calculated using the formula:
where Vc and Vt represent the mean increase in paw volume in carrageenan control and treated groups, respectively. After 5 h of carrageenan challenge, rats were sacrificed by ether overdose. Blood samples and tissues of hind paw for homogenate preparation were collected and stored at −80 °C until biochemical analysis.
Preparation of paw tissues for histopathology
After the last determination of paw edema (5 h after the carrageenan injection), animals were sacrificed and paw samples were collected for histopathological observation. The paws were decalcified in 5% formic acid, processed for paraffin embedding, sectioned at 5 μm thicknesses, and subsequently strained with hematoxylin and eosin (H & E) for examination under a light microscope. The micrographs were evaluated histologically by an independent observer.
Biochemical parameters
Malondialdehyde (MDA) level in plasma was measured by the thiobarbituric acid reacting substances (TBARS) method in heparinized blood plasma (Brown & Kelly, Citation1996). The amount of 1.5 ml of 0.67% thiobarbituric acid, 1.5 ml of 20% trichloroacetic acid, 700 μl of phosphate buffer, and pH 7.4 were added to 300 μl of plasma, then mixed and incubated in a water bath at 90 °C for 30 min. The reaction was stopped by dipping the test tubes into ice for 10 min. Samples were centrifuged at 3000 rpm. The supernatant was removed and the absorbance was measured spectrophotometrically at 535 nm in a 1 cm cuvette. The concentration of MDA was calculated based on the absorbance coefficient of TBA-MDA complex (ε = 1.56 × 105 M−1 cm−1) and it was expressed as nmol/ml. Plasma concentration of C-reactive protein was determined by Rat CRP ELISA assay (Eckersall, Citation2000). Evaluation was performed according to the manufacturer's protocols.
NO level in rat paw tissue was measured by means of the Griess method (Wang et al., Citation2000). Briefly 10% paw tissue was homogenized in ice-cold potassium phosphate buffer (pH 7.4) and centrifuged at 3000 rpm for 10 min at 4 °C. The resulting supernatant was used to estimate NO. Fifty microliters of each experimental sample was added to a 96-well plate in duplicate. Similarly 50 µl of standard sodium nitrite (50, 25, 12.5, 6.25, 3.125, and 1.56 μM/ml) was placed in different wells of the 96-well plate, in duplicate. After this, 50 µl of the sulfanilamide solution (1% sulfanilamide in 5% phosphoric acid) was dispensed to all the wells containing experimental samples and nitrite standards. The plate was incubated for 5–10 min at room temperature, protected from light. Following this 50 µl of the NED, 0.1% N-1-napthylethylenediamine dihydrochloride in distilled water solution was dispensed in all wells. The plate was incubated again for 5–10 min at room temperature, protected from light. A purple/magenta color begins to from immediately. Absorbance was measured within 30 min in an ELISA plate reader with a filter of 520 nm and was expressed as micromoles/ml. NO concentration was calculated by using the standard curve equation.
Tissue neutrophil infiltration was quantified by measuring the MPO activity using a spectrophotometric method as proposed by Bradley et al. (Citation1982). Briefly, 10% paw tissue was homogenized in homogenizing solution containing 50 mM potassium phosphate buffer (pH 6.0) with 0.5% hexadecyltrimethylammonium bromide and 5 mM EDTA. The homogenate was sonicated and centrifuged at 15 000 g for 15 min at 4 °C. The supernatant was mixed in a ratio of 1:15 with assay buffer comprising 100 mM potassium phosphate buffer (pH 6.0), 0.167 mg o-dianisidine hydrochloride per ml, and 0.0005% hydrogen peroxide. MPO activity was assayed by measuring the change at 460 nm from 0 min to 4 min over intervals of 30 s. MPO activity was calculated by using an absorption coefficient of o-dianisidine 11.3 mM−1 cm−1 at 460 nm and was reported as micromoles/ml.
Immunological parameters
The concentrations of TNF-α and IL-1β were measured by rat enzyme-linked immunosorbent assay (ELISA) according to the manufacturer's instructions (Blankenstein et al., Citation1991; Brouckaert et al., Citation1993).
Statistical analysis
All the results were expressed as mean ± standard error mean (SEM). Data were analyzed using one-way ANOVA followed by the Tukey post test (GraphPad InStat 3 software, GraphPad Software, San Diego, CA). Values of p < 0.05 were considered as statistically significant.
Results
Preliminary phytochemical screening
Phytochemical investigation of CAME showed the presence of alkaloids, phenolics, flavonoids, coumarin, terpinoids, and saponin glycosides.
Quantification of quercetin and betulinic acid by HPTLC
The Rf value of standard quercetin was found to be 0.37 and peak area 7347.46 at 120 ng/spot (). The methanol extract of C. arborea showed 13 peaks, the Rf value (0.38) of sixth peak was coinciding with quercetin Rf value (0.38) and its peak area was 2318.60 (), the amount of quercetin was found to be 0.177%. The Rf value of standard betulinic acid was found to be 0.53 and peak area 2443.66 at 600 ng/spot (). The methanol extract of C. arborea showed 11 peaks, the Rf value (0.53) of sixth peak was coinciding with betulinic acid Rf value (0.53) and its peak area was 1709.33 (), the amount of betulinic acid was found to be 3.14%.
Carrageenan induced paw edema
The anti-inflammatory activity of CAME (100 and 200 mg/kg) measured against acute paw edema induced by carrageenan is presented in .
Figure 3. Effect of CAME on paw volume in carrageenan-induced inflammed rats. Each value is mean ± SEM (n = 6). ***p < 0.001 when compared with untreated carrageenan control.
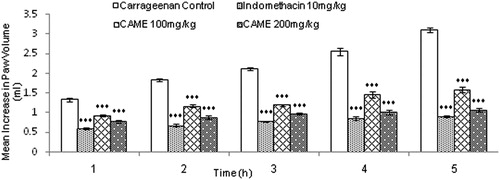
CAME (100 and 200 mg/kg) decreased the edema significantly (p < 0.001) at 1, 2, 3, 4, and 5 h after carrageenan injection when compared with the carrageenan control group. The higher dose of CAME (200 mg/kg) exhibited inhibition of inflammation, close to the inhibitory effect of indomethacin (10 mg/kg). Administration of CAME inhibited paw edema 48.87% (100 mg/kg) and 65.53% (200 mg/kg), whereas indomethacin (10 mg/kg) showed 70.63% inhibition when compared with carrageenan control.
Histopathology of paw tissues
Representative histopathology of paw tissue of the experimental groups is shown in . Histopathology of paw tissue in the carrageenan group showed evidence of pronounced inflammatory cell infiltration () whereas the normal control group showed the absence of inflammatory cells (). Rats treated with CAME (100 and 200 mg/kg) revealed only a few scattered inflammatory cells (, respectively). The indomethacin (10 mg/kg) treated group showed only minimal inflammatory cells ().
Figure 4. Photomicrograph of left hind paw tissue section stained by H & E. (A) Photomicrograph of paw tissue section from normal control rat. (B) Photomicrograph of paw tissue section from carrageenan control rat. (C) Photomicrograph of paw tissue section from indomethacin (10 mg/kg) treated rat. (D) Photomicrograph of paw tissue section from CAME (100 mg/kg) treated rat. (E) Photomicrograph of paw tissue section from CAME (200 mg/kg) treated rat (magnification: 400×; scale bar: 20 µm).
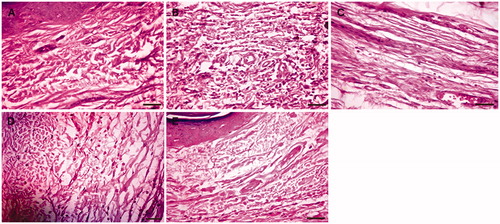
Effect of CAME on biochemical parameters
In carrageenan-induced acute inflammation, the changes in inflammatory biomarkers like MDA, CRP, NO, and MPO were remarkably increased due to inflammation.
The MDA level was significantly (p < 0.001) increased in the carrageenan control group while compared with normal control (). Administration of CAME (100 and 200 mg/kg) resulted in significant (p < 0.01 and p < 0.001, respectively) decreases in MDA level while compared with carrageenan control. As shown in the results (), the plasma level of CRP was significantly (p < 0.001) elevated in carrageenan control and treated animals as compared with the normal control group. While treating with CAME (100 and 200 mg/kg) and indomethacin (10 mg/kg), there was a significant (p < 0.05, p < 0.001, and p < 0.001, respectively) reduction in CRP levels as compared with the diseased control group. NO level increased dramatically in the carrageenan control whereas it was non-significant in CAME higher dose and indomethacin-treated groups () as compared with normal control. However, CAME (100 and 200 mg/kg) caused a significant (p < 0.05 and p < 0.01, respectively) decrease in NO levels while compared with the carrageenan control group. Similarly, while comparing the indomethacin (10 mg/kg), treatment group dramatically decreased the NO level (p < 0.001). Paw tissue MPO level of the carrageenan control group was increased significantly (p < 0.001) due to carrageenan injection (). Administration of CAME (100 and 200 mg/kg) and indomethacin (10 mg/kg) significantly decreased the MPO level (p < 0.05, p < 0.01, and p < 0.01, respectively) at the end of the study period.
Figure 5. Effect of CAME on plasma malondialdehyde and C-reactive protein levels of inflamed rats. (A) Malondialdehyde (MDA) level in the plasma. (B) C-reactive protein (CRP) level in the plasma. Each value is mean ± SEM (n = 6) §p < 0.05, §§p < 0.01, and §§§p < 0.001, a = non-significant when compared with normal control. *p < 0.05, **p < 0.01, and ***p < 0.001, b=non-significant when compared with untreated carrageenan control.
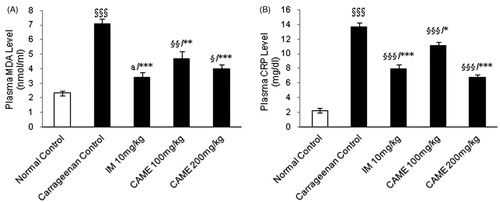
Figure 6. Effect of CAME (100 and 200 mg/kg) on nitric oxide and myeloperoxidase levels of inflamed rats paw tissue. (A) Nitric oxide (NO) level in the paw tissue homogenate. (B) Myeloperoxidase (MPO) level in the paw tissue homogenate. Each value is mean ± SEM (n = 6). §p < 0.05, §§p < 0.01, and §§§p < 0.001, a = non-significant when compared with normal control. *p < 0.05, **p < 0.01, and ***p < 0.001 when compared with untreated carrageenan control.
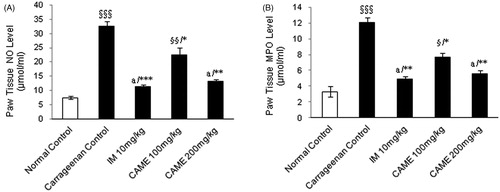
Effect of CAME on immunological parameters
To further elucidate the mechanism of action of CAME in inflammation, pro-inflammatory cytokines such as TNF-α and IL-1β were determined using ELISA technique. The serum level of TNF-α in the carrageenan-induced inflammation group was significantly (p < 0.001) increased compared with the normal control group, but pre-treatment with CAME (100 and 200 mg/kg) reduced its serum level significantly (p < 0.05 and p < 0.01, respectively) which is comparable with the indomethacin treated group (10 mg/kg) (). As shown in , the carrageenan-induced inflammation group showed significant (p < 0.001) increased serum level of IL-1β, whereas pre-treatment with indomethacin (10 mg/kg) and CAME (200 mg/kg) showed significant (p < 0.05) inhibition in serum IL-1β level when compared with carrageenan control. No significant difference was found at the lower dose of CAME.
Figure 7. Effect of CAME (100 and 200 mg/kg) on serum pro-inflammatory cytokines (TNF-α and IL-1β) of inflamed rats. (A) TNF-α level in the serum. (B) IL-1β level in the serum. Each value is mean ± SEM (n = 6). §p < 0.05, §§p < 0.01, and §§§p < 0.001, a = non-significant when compared with normal control. *p < 0.05 and **p < 0.01, b = non-significant when compared with untreated carrageenan control.
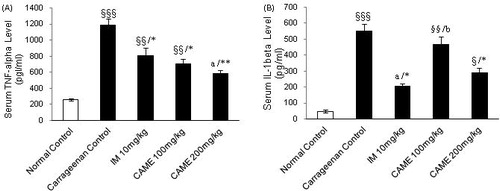
Discussion
Conventional NSAIDs are the most commonly prescribed agents used for the management of inflammation and pain; however, toxic manifestation associated with these agents is a matter of concern. As a result, several new approaches are now considered for designing and development of superior anti-inflammatory compounds, showing fewer side effects (Mani et al., Citation2008). On phytochemical screening, CAME showed the presence of numerous amounts of phenols, triterpinoids, steroids, and flavonoids as major constituents. HPTLC of CAME confirmed the presence of significant amount of the quercetin. Quercetin, a dietary-derived flavonoid, is ubiquitous in fruits and vegetables and plays important roles in human health by virtue of its antioxidant activity (Mariee et al., Citation2012). Both in vitro and in vivo studies suggest that quercetin ameliorates inflammation by inhibition of either the secretion of inflammatory mediators such as nitrogen reactive species produced after induction of iNOS expression or the expression and subsequent release of cytokines such as TNF-α and IL-1β (Comalada et al., Citation2005). In this present study, CAME showed the presence of abundant amount of the betulinic acid. Betulinic acid affected the levels of COX-2, NO, TNF-α, and IL-1β in the lambda-carrageenan-induced paw edema (Tsai et al., Citation2011). Thus the presence of quercetin and betulinic acid in CAME might be responsible for anti-inflammatory activity of C. arborea.
Carrageenan-induced paw edema animal model is usually used to assess the contribution of natural products in resisting the biochemical changes associated with acute inflammation. Carrageenan can induce acute inflammation beginning with the infiltration of phagocytes, the production of free radicals as well as the release of inflammatory mediators. Edema formation due to carrageenan in rat is a biphasic event. The initial phase of edema is due to the release of histamine and serotonin and the second phase of edema is due to release of prostaglandins, protease, and lysosomal enzymes (Vinegar et al., Citation1969). Further, it has been demonstrated that the second phase is sensitive to the most clinically effective anti-inflammatory drugs (Brooks & Day, Citation1991). In the present experiments, CAME inhibited the carrageenan edema in a dose-dependent manner at the end of the study period. The progression of inflammation in the second phase was less. Hence, CAME might elicit its anti-inflammatory activity by inhibiting synthesis and release of prostaglandins, proteases, and lysosomal enzymes like non-steroidal anti-inflammatory drugs.
Lipid oxidation not only serves as a marker of cellular damage in vivo but also has been recognized to be the inducer of inflammatory processes. Some researches demonstrate that inflammatory effect induced by carrageenan is associated with free radicals. Free radicals, prostaglandin, and NO will be released when administrating with carrageenan for 1–6 h. MDA production is due to free radicals attack in plasma membrane (Huang et al., Citation2011a,Citationb). In this study, we have noticed that elevated plasma MDA level in carrageenan induced inflamed rats. Increased level of MDA is due to the damage of plasma membrane. Pre-treatment with CAME significantly reduced MDA as compared with the carrageenan control group.
To understand the mechanism of anti-inflammatory activity of methanol extracts of stem bark of C. arborea better, the effect of CAME on C-reactive protein level was further studied. Since CRP level in the blood rises more quickly when the inflammatory or infective process begins, due to a rise in the plasma concentration of IL-6, which is produced predominantly by macrophages (Pepys & Hirschfield, Citation2003). The present study revealed that the CRP level in plasma of carrageenan control was approximately six times higher than in the normal control while treating with CAME (100 and 200 mg/kg) lowered the CRP level significantly and dose dependently as compared with the carrageenan control group. This indicates that test drugs have potential inhibitory effects on acute phase protein of inflammation.
NO is a major product and its production is controlled by the nitric oxide synthases (NOS), which include iNOS, eNOS, and nNOS. Most importantly, iNOS is highly expressed in macrophages; its activation leads to organ destruction in inflammatory and autoimmune diseases. Therefore, inhibition of NO production by iNOS may have potential therapeutic value related to inflammation. Furthermore, under inflammatory conditions, macrophages can greatly increase (Yoon et al., Citation2009). In the present study, the NO level was found to be significantly elevated in the carrageenan control compared with normal control whereas the NO level was inhibited significantly and dose dependently by treating with CAME. This indicates that the anti-inflammatory mechanism of C. arborea might be through the l-arginine–NO pathway.
The present study reflects the increased level of MPO activity in the hind paw tissue homogenate of carrageenan control while compared with the normal group. However, a significant protective effect against this increase was observed by the treatment with CAME and standard drug. This finding has significant importance as MPO is the most abundant enzyme in neutrophils. MPO enhances the binding of leukocytes, including monocytes and neutrophils, to the endothelium (Johansson et al., Citation1997).
In the experiment, CAME and indomethacin almost equally lowered IL-1β. There is evidence that pro-inflammatory cytokines such as TNF-α and IL-1 help to propagate the extension of a local or systemic inflammatory process (Mizgerd et al., Citation2001). TNF-α and IL-1β are very important pro-inflammatory substances that are related to the release of other pro-inflammatory mediators, including bradykinin and substance P (Kuo et al., Citation2000; Santos et al., Citation2003). CAME was more effective in suppressing the serum level of TNF-α than indomethacin. TNF-α plays several important roles in inflammation based on its appearance at the inflammatory site and ability to induce certain mechanisms including activation and chemotaxis of leukocytes, expression of adhesion molecules on neutrophils and endothelial cells, and regulation of the secretion of other pro-inflammatory cytokines (Cha et al., Citation2011; Collins et al., Citation2001; Park et al., Citation2002).
In conclusion, the inhibitory effect of C. arborea upon leukocyte influx to the inflammation induced by carrageenan in the rat paw demonstrated here in can be attributed to the suppression of the release of inflammatory mediators such as MDA, CRP, NO, MPO, and pro-inflammatory cytokines (TNF-α and IL-1β). Higher dose of extract (200 mg/kg) has shown better inhibitory effect on various inflammatory biomarkers than the lower dose (100 mg/kg). Finally, we may conclude that these results support the traditional use of C. arborea in some inflammatory and painful conditions and confirm the presence of active chemical compounds related to these activities. Further study to isolate, identify, and characterize the active constituents responsible for these effects is progressing.
Acknowledgements
The authors thank Dr. Mukherjee (Pathologist, All India Institute of Medical Science, New Delhi, India) for his technical support in histological staining and analysis.
Declaration of interest
Authors have declared no conflicts of interest. The authors thank ICCR for sponsoring the work.
References
- Basak A, Banerjee S, Bose L, Basu K. (1976). Chemical examination of the leaves of Careya arborea. J Indian Chem Soc 53:639–40
- Bentham G, Hooker JD. (1985). Genera Plantarum. London: Reeve & Co. Ltd., 721
- Blankenstein T, Qin ZH, Uberia K, et al. (1991). Tumor suppression after tumor cell-targeted tumor necrosis factor alpha gene transfer. J Exp Med 173:1047–52
- Bradley PP, Priebat DA, Christensen RD, Rothstein G. (1982). Measurement of cutaneous inflammation: Estimation of neutrophils content with an enzyme marker. J Invest Dermatol 78:206–9
- Brooks PM, Day RO. (1991). Non-steroidal anti-inflammatory drugs: Differences and similarities. N Engl J Med 324:1716–25
- Brouckaert P, Libert C, Everaerdt B, et al. (1993). Tumor necrosis factor, its receptors and the connection with interleukin 1 and interleukin 6. Immunobiology 187:317–29
- Brown RK, Kelly FJ. (1996). Peroxides and other products. In: Punchard NA, Kelly FJ, eds. Free Radicals, A Practical Approach. Oxford: Oxford University Press, 119–31
- Cha DS, Eun JS, Jeon H. (2011). Anti-inflammatory and antinociceptive properties of the leaves of Eriobotrya japonica. J Ethnopharmacol 134:305–12
- Collins RA, Grounds MD. (2001). The role of tumor necrosis factor-alpha (TNF-α) in skeletal muscle regeneration: Studies in TNF-α(−/−) and TNF-α(−/−)/LT-α(−/−) mice. J Histochem Cytochem 49:989–1001
- Comalada M, Camuesco D, Sierra S, et al. (2005). In vivo quercetin anti-inflammatory effect involves release of quercetin, which inhibits inflammation through down-regulation of the NF-κB pathway. Eur J Immunol 35:584–92
- Das MC, Mahato SB. (1982). Triterpenoid sapogenols from leaves of Careya arborea structure of careyagenolide. Phytochemistry 21:2069–73
- Eckersall PD. (2000). Recent advances and future prospects for the use of acute phase proteins and markers of disease in animals. Rev Med Vet 151:577–84
- Gedeon J, Kinel FA. (1956). Saponins and sapogenins. 2. Arch Pharm (Weinheim) 289:162–5
- Gupta RK, Chakraborty NK, Dutta TR. (1975). Crystalline constituents from Careya arborea Roxb. Indian J Pharm 37:161–2
- Harborne JB. (1998). Phytochemcial Methods, 3rd ed. London: Chapman and Hall Ltd
- Huang MH, Huang SS, Wang BS, et al. (2011a). Antioxidant and anti-inflammatory properties of Cardiospermum halicacabum and its reference compounds ex vivo and in vivo. J Ethnopharmacol 133:743–50
- Huang SS, Chiu CS, Chen HJ, et al. (2011b) Antinociceptive activities and the mechanisms of anti-inflammation of Asiatic acid in mice. Evid Based Compl Alt Med 2011:1–10
- Johansson MW, Patarroyo M, Oberg F, et al. (1997). Myeloperoxidase mediates cell adhesion via the alpha M beta 2 integrin (Mac-1, CD11b/CD18). J Cell Sci 110:1133–9
- Kirtikar KR, Basu BD. (1975). Indian Medicinal Plants 2, 2nd ed. Dehradun, India: Bishen Singh Mahendra Pal Singh International Book Distributors and Publishers, 894–5
- Kokate CK, Purohit AP, Gokhale SB. (2005). Pharmacognosy. Pune: Nirali Prakashan
- Kulakkattolickal AT. (1987). Piscicidal plants of Nepal, preliminary toxicity screening using grass carp (Ctenopharyngodon idella) Fingerlings. J Ethnopharmacol 21:1–9
- Kuo HP, Lin HC, Hwang KH, et al. (2000). Lipopolysaccharide enhances substance P-mediated neutrophil adherence to epithelial cells and cytokine release. AM J Resp Crit Care 162:1891–7
- Mahati SB, Dutta NL, Chakravarti RN. (1973) Trierpenes from Careya arborea: Structure of careyageno D. J Indian Chem Soc 50:254–9
- Mahato SB, Datta NL. (1972). Sterols from Careya arborea. Phytochemistry 11:2116–17
- Mani Senthil Kumar KT, Gorain B, Roy DK, et al. (2008). Anti-inflammatory activity of Acanthus ilicifolius. J Ethnopharmacol 120:7–12
- Mariee AD, Abd-Allah GM, El-Beshbishy HA. (2012). Protective effect of dietary flavonoid quercetin against lipemic-oxidative hepatic injury in hypercholesterolemic rats. Pharm Biol 50:1019–25
- Mizgerd JP, Spieker MR, Doerschuk CK. (2001). Early response cytokines and innate immunity: Essential roles for TNF receptor 1 and type 1 IL-1 receptor during Escherichia coli pneumonia in mice. J Immunol 166:4042–8
- Park SJ, Lee SC, Hong SH, Kim HM. (2002). Degradation of IkappaBalpha in activated RAW264.7 cells in blocked by the phosphatidylinositol 3-kinase inhibitor LY294002. Cell Biol Toxicol 18:121–30
- Pepys MB, Hirschfield GM. (2003). C-reactive protein: A critical update. J Clin Invest 111:1805–12
- Ramchandra RL, Sashtry PCS. (1976). Chemical examination of Careya arborea Roxb. Indian J Chem 2:510–14
- Rao YK, Fang SH, Tzeng YM. (2007). Evaluation of the anti-inflammatory and antiproliferation tumoral cells activities of Antrodia camphorata, Cordyceps sinensis and Cinnamomum osmophloeum bark extracts. J Ethnopharmacol 114:78–85
- Rosenberg HF, Gallin JI. (1999). Inflammation. In: Paul WE, ed. Fundamental Immunology, 4th ed. Philadelphia, New York: Lippincott-Raven, 1051–66
- Saldanha CJ, Nicolson DH. (1976). Flora of Hassan District, Karnataka, India. New Delhi: Amerind Publishing House, 157
- Sambathkumar R, Sivakumar T, Shanmuga SR, et al. (2005). Anti-inflammatory and analgesic effects of Careya arborea stem bark in experimental animal models. Nigeria J Nat Prod Med 9:38–43
- Santos DR, Calixto JB, Souza GE. (2003). Effect of a kinin B2 receptor antagonist on LPS- and cytokine-induced neutrophil migration in rats. Brit J Pharmacol 139:271–8
- Senthilkumar N, Badami S, Dongre SH, Bhojraj S. (2008). Antioxidant and hepatoprotective activity of the methanol extract Careya arborea bark in Ehrlich ascites carcinoma bearing mice. J Nat Med Tokyo 62:336–9
- Shah BS, Nayak BS, Seth AK, et al. (2006). Search for medicinal plants as a source of anti-inflammatory and anti-arthritic agents – A review. Pharmacogn Mag 2:77–86
- Tsai JC, Peng WH, Chiu TH, et al. (2011). Anti-inflammatory effects of Scoparia dulcis L. and betulinic acid. Am J Chin Med 39:943–56
- Vinegar R, Schreiber W, Hugo R. (1969). Biphasic development of carrageenan edema in rats. J Pharmacol Exp Ther 166:96–103
- Wagner H, Baldt S, Zgainski EM. (1996). Plant Drug Analysis. Berlin: Springer
- Wang CC, Lai JE, Chen LG, et al. (2000). Inducible nitric oxide synthase inhibitor of Chinese herbs part II: Naturally occurring furanocoumarins. Bioorg Med Chem 8:2701–7
- Winter CA, Risley E, Nuss G. (1962). Carrageenan-induced edema in hind paws of the rat as an assay for anti-inflammatory drugs. Proc Soc Exp Biol Med 111:544–7
- Yoon WJ, Ham YM, Kim SS, et al. (2009) Suppression of pro-inflammatory cytokines, iNOS and COX-2 expression by brown algae Sargassum micracanthum in RAW 264.7 macrophages. Eur Asia J BioSci 3:130–43