Abstract
Context: Nardostachys chinensis Batalin (Valerianaceae) has been used in Korean traditional medicine to elicit stomachic and sedative effects. However, the anti-leukemic activities of N. chinensis have not been well examined.
Objective: To investigate the effect of N. chinensis on differentiation and proliferation in the human promyelocytic leukemic HL-60 cells.
Materials and methods: The dried roots and stems of N. chiensis are extracted using hot water and then freeze-dried. The yield of extract was 12.82% (w/w). The HL-60 cells were treated with 25–200 μg/ml of N. chinensis for 72 h or 100 μg/ml of N. chinensis for 24–72 h.
Results: Nardostachys chinensis significantly inhibited cell viability dose dependently with an IC50 of 100 μg/ml in HL-60 cells. Nardostachys chinensis induced differentiation of the cells as measured by reduction activity of NBT and expression of CD11b but not of CD14 as analyzed by flow cytometry, which indicates a differentiation toward the granulocytic lineage. Nardostachys chinensis also induced growth inhibition through G0/G1 phase arrest in the cell cycle of HL-60 cells. Among the G0/G1 phase in the cell cycle-related protein, the expression of cyclin-dependent kinase (CDK) inhibitor p27Kip1 was increased in N. chinensis-treated HL-60 cells, whereas the expression levels of CDK2, CDK4, CDK6, cyclin D1, cyclin D3, cyclin E, and cyclin A were decreased. Interestingly, N. chinensis markedly enhanced the binding of p27Kip1 with CDK2 and CDK6.
Discussion and conclusion: This study demonstrated that N. chinensis is capable of inducing cellular differentiation and growth inhibition through p27Kip1 protein-related G0/G1 phase arrest in HL-60 cells.
Introduction
A variety of chemical and biological agents can induce cellular differentiation of leukemic cells, and thus have an anti-leukemic activity (Breitman et al., Citation1980; Collins et al., Citation1978; Huberman & Callaham, Citation1979; Rovera et al., Citation1979). The human promyelocytic leukemic HL-60 cells, when treated with anti-leukemic agents, can be differentiated into the two types of cells; monocyte/macrophage-like cells and granulocyte-like cells in myeloid lineage (Breitman et al., Citation1980; Collins et al., Citation1978; Huberman & Callaham, Citation1979; Rovera et al., Citation1979). 12-O-Tetradecanoyl-phorbol-13-acetate and all-trans-retinoic acid (ATRA) are well-known inducers that can stimulate the differentiation of HL-60 cells into monocyte/macrophage-like and granulocyte-like phenotypes, respectively (Breitman et al., Citation1980; Collins et al., Citation1978; Huberman & Callaham, Citation1979). After exposure to these agents, HL-60 cells tend to stop cellular proliferation and also to express the phenotypical and physiological functions to be specific to mature cells. It has been shown that cell differentiation is an important approach to cancer therapy (Lo Coco et al., Citation2002; Spira & Carducci, Citation2003; Wang et al., Citation1999), because cancer cells, generally, are not fully differentiated. Although this differentiation therapy is well known for hematological malignancies, particularly leukemia (Aksentijevich & Flinm et al., Citation2003; Parmar & Tallman, Citation2003), the differentiation-based treatment can be also applicable to prostate (Myers, Citation1999; Sciarra et al, Citation1998; Walczak et al., Citation2001), breast (Baj et al., Citation2000; Yang et al., Citation2002), and other (Patnaik et al., Citation2002) cancers as well.
Cell differentiation is usually associated with the exodus of cells from cell cycle. Cell-cycle progression in mammalian cells is regulated by a family of enzymes known as cyclin-dependent kinases (CDKs) whose activity is dependent on binding to specific regulatory subunits called cyclins (Covacci et al., Citation1998). The activity of cyclin/CDK complexes is negatively regulated by specific CDK inhibitors (CDKIs) (Sherr & Roberts, Citation1995). These CDKIs play a key role in controlling cell-cycle progression by inhibiting CDK activity at the appropriate time in the cell cycle (Brugarolas et al., Citation1995; Elledge & Harper, Citation1994; Peter & Herskowitz, Citation1994; Sherr & Roberts, Citation1995). There are two classes of mammalian CDKIs known to date. One group is CIP/KIP family, including p21WAF/Cip1, p27Kip1 and p57, with broad specificity (Brugarolas et al., Citation1995; Sherr & Roberts, Citation1995), and the other is INK4 family, including p15INK4B, p16INK4A, p18INK4C, and p19INK4D, which target CDK4 and CDK6 (Sherr & Roberts, Citation1995). The mechanisms for regulation of cell cycle have a fundamental role in the control of cell proliferation and may also be involved in differentiation.
Nardostachys chinensis Batalin (Valerianaceae) has been used in Korean traditional medicine to elicit stomachic, anti-arrhythmic, and sedative effects (Seo et al., Citation2008). The plant is known to be rich in sesquiterpenoids (Xiao, Citation2002), which have been found to exhibit antimalarial, antinociceptive, and cytototic activities (Itokawa et al., Citation1993; Takaya et al., Citation2000), as well as to enhance nerve growth factors (Li et al., Citation2003). However, the anticancer and anti-leukemic activities of N. chinensis have not been well examined. This study investigated the anti-leukemic effects of N. chinensis on HL-60 cells.
Materials and methods
Regents and antibodies
3-(4,5-Dimethyl-2-thiazolyl)-2,5-diphenyl-2H-tetrazolium bromide (MTT), nitroblue tetrazolium (NBT), phorbol 12-myristate 13-acetate (PMA), propidium iodide (PI), ribonuclease A (RNase A), and protease inhibitor cocktail were purchased from Sigma-Aldrich Chemical (St. Louis, MO). RPE-conjugated anti-CD11b and FITC-conjugated anti-CD14 antibodies were purchased from DAKO (Glostrup, Denmark). RPMI 1640, fetal bovine serum (FBS), and antibiotic-antimycotic solution were purchased from GIBCO (Grand Island, NY). Protein A/G PLUS-Agarose, anti-p27Kip1, anti-CDK2, anti-CDK4, anti-CDK-6, anti-cyclin D1, anti-cyclin D3, anti-cyclin A, and anti-cyclin E antibodies were purchased from Santa Cruz Biotechnology Inc. (Santa Cruz, CA). Anti-p27Kip1 monoclonal antibody was purchased from BD biosciences (San Jose, CA). HRP-conjugated goat anti-rabbit IgG and rabbit anti-mouse antibodies were purchased from Invitrogen (Burlington, ON, Canada).
Preparation of N. chinensis extract
The dried roots and stems of N. chiensis were purchased from Nonglim-Saengyak (Seoul, Korea) in March 2013. Nardostachys chinensis was identified by Profs. Jeon and Lee, two of the authors. A voucher specimen (DP-2012-NC) has been deposited in the Department of Pathology, College of Korean Medicine, Wonkwang University. An aqueous extract was prepared by boiling 100 g of N. chinensis with 1 L of distilled water for 2 h, and then centrifuged at 2000 rpm for 15 min to remove the insoluble ingredients. The supernatant was filtered through Whatman No. 4 filter paper in a Buchner funnel under vacuum and stored at −20 °C for overnight. Frozen extract was freeze-dried in a vacuum chamber. The yield of extract was 12.82% (w/w). Freeze-dried powder was dissolved in PBS (pH 7.4) at a concentration 40 mg/ml and stored at −20 °C and diluted in cell culture medium before use.
HPLC analysis of N. chinensis extract
Aqueous extract of N. chinensis was analyzed by SmartLC (LC800, GL Sciences, Kyoto, Japan) equipped with an Inertsil ODS-4 column (2.1 × 50 mm, 2 μm; GL Sciences, Kyoto, Japan) at 35 °C. The mobile phase consisted of water (A) and acetonitrile (B). A gradient program of mobile phase was used as follows: 10% (B) maintained for 1 min, 10–90% (B) over 1–9 min. The final concentration of nardosinone dissolved in methanol was 0.1 mg/ml and that of the water extract dissolved in water was 25 mg/ml. The flow rate was set at 0.4 ml/min and the injection volume was 1 μl. A detection wavelength of nardosinone was set at 280 nm. The acquired data were processed using EZChrom Elite software (Ver. 3.3.2 SP1, Agilent Technologies Inc., Santa Clara, CA).
Cell culture
HL-60 cells were obtained from the American Type Culture Collection (ATCC, Rockville, MD) and routinely cultured in RPMI 1640 medium supplemented with 10% FBS in 1:100 dilution of an antibiotic–antimycotic solution at 37 °C in a 5% CO2 incubator.
Cell viability assay
Exponentially growing cells were seeded into a 24-well plate at 1 × 105 cells/well in duplicate. The cells were treated with N. chinensis or ATRA for 72 h. After the indicated time periods, 100 μl of 5 mg/ml MTT solution was added to each wells and incubated for 4 h. Water-insoluble MTT-formazan crystals were solubilized by adding equal volume of solubilization solution (10% SDS/0.01 N HCl) and incubating the plate overnight in humidified atmosphere of 5% CO2 at 37 °C. The amount of formazan was determined at 570 nm using SpectraMAX 250 microplate spectrophotometer (Molecular Devices, Sunnyvale, CA). Percentage of the cell viability was calculated using the equation: (mean OD of treated cells/mean OD of untreated cells) × 100.
Differentiation assay
HL-60 cells (1 × 106/60 mm dish) were cultured with N. chinensis or ATRA in complete medium for 72 h, and then the cells were harvested, washed twice with ice-cold PBS (pH 7.4). NBT reducing activity was determined by the method of Sakashita et al. (Citation1991), with a slight modification. In brief, the cells were suspended in 200 μl of 2 mg/ml NBT solution. After the addition of 2 μl of 100 μg/ml PMA solution, the cell suspension was incubated at 37 °C for 20 min, 200 μl of 1 N HCl was added at 4 °C to terminate the reaction. After centrifugation, 600 μl of DMSO was added to the cell pellets to solubilize the formazan deposits. The amount of formazan was determined at 560 nm using a SpectraMAX 250 microplate spectrophotometer (Molecular Devices, Silicon Valley, CA). Differentiation markers were determined by flow cytometry using RPE or FITC-labeled antibodies. The cells were suspended in 100 μl of PBS (pH 7.4) containing 0.25% BSA. After the addition of 10 μl of RPE-labeled anti-CD 11b or FITC-labeled anti-CD 14 antibodies, the cells were incubated in the dark at 4 °C for 30 min, washed twice with PBS (pH 7.4) containing 0.25% BSA, fixed in 500 μl of PBS (pH 7.4) containing 1% formaldehyde, and then the level of antibody binding to the cells were quantified using fluorescence-activated cell sorting (FACS) Calibur (BD Biosciences, San Jose, CA).
Cell-cycle analysis
Cell-cycle progression was monitored by quantitating cellular DNA content after staining with PI. Untreated or N. chinensis-treated HL-60 cells were harvested and washed with PBS (pH 7.4). The cells were fixed with ice-cold 100% ethanol for 1 h, washed with PBS (pH 7.4), and then treated with 10 μg/ml PI/100 μg/ml RNase A solution at 37 °C for 1 h in the dark condition, and analyzed on a FACS Calibur. Cell-cycle analysis was determined by DNA content from fixed cells stained with PI.
Western blot analysis
Cells were washed with ice-cold PBS (pH 7.4), gently resuspended in ice-cold lysis buffer (50 mM Tris-HCl, pH 7.4, 150 mM NaCl, 1% NP-40, 0.5% sodium deoxycholate, 0.1% SDS, 1% protease inhibitor cocktail), and incubated on ice for 30 min. Cell lysates were centrifuged at 14 000 rpm for 10 min at 4 °C, and the protein concentration was determined using a Bradford assay. Samples containing 40 μg of total protein were resolved by 10–15% SDS-PAGE gel, and transferred onto a nitorcellulose membrane for 3 h at 40 V. The membranes were blocked with Tri-buffered saline with Tween-20 (TBST; 20 mM Tris-HCl, pH 7.6, 150 mM NaCl, 0.1% Tween 20) containing 5% non-fat dry milk for 1 h at room temperature and probed with different primary antibodies (diluted 1:1000 in 3% non-fat dry milk in TBST) overnight at 4 °C with gentle shaking. Blots were washed three times for 10 min in TBST and then incubated with anti-rabbit or anti-mouse peroxidase-conjugated secondary immunoglobulin G antibodies (diluted 1:2000 in 3% non-fat dry milk in TBST) for 1 h at room temperature. The blots were visualized by chemiluminescence using SuperSignal West Pico Chemiluminescent Substrate Kit (Thermo Fisher Scientific, Waltham, MA) and Molecular Imager ChemiDoc XRS System (Bio-Rad, Hercules, CA). The density of bands was quantitated using Quantity One 4.6.6 software (Bio-Rad, Hercules, CA).
Immunoprecipitation
Cells were lysed with immunoprecipitation lysis buffer (50 mM Tris-HCl, pH 7.4, 150 mM NaCl, 1% NP-40, 0.5% sodium deoxycholate, 1% protease inhibitor cocktail) for 15 min on ice. Cell lysates were centrifuged at 11 000 rpm for 15 min at 4 °C, and then pre-cleared using Protein A/G PLUS-Agarose. Pre-cleared lysates were quantitated using a Bradford assay. About 200 μg of the total protein were incubated with 10 μl of the anti-CDK2, anti-CDK4, and anti-CDK6 antibodies for 6 h at 4 °C, followed by incubation with 20 μl of the Protein A/G PLUS-Agarose for 2 h. The immunoprecipitates were washed three times with immunoprecipitation wash buffer (50 mM Tris-HCl, pH 7.4, 150 mM NaCl, 0.5% NP-40), and samples were boiled in 2 × SDS sample buffer (125 mM Tris-HCl, pH 6.8, 4% SDS, 10% β-mercaptoethanol, 2% glycerol, and 0.02% bromophenolblue) for 5 min followed by centrifugation; the reaction mixture was then resolved by SDS-PAGE gel, transferred onto a nitrocellulose membrane by electroblotting and probed with the anti-p27Kip1 monoclonal antibody.
Statistical analysis
Statistical analysis was performed using Microsoft Office Excel 2010 (Microsoft, Redmond, WA). The data were expressed as means ± standard deviation (SD). The statistically significant differences between two groups were calculated by Student's t-test. p < 0.05 was considered to be significant.
Results
Identification of nardosinone and cytotoxicity testing of aqueous extract of N. chinensis
Nardosinone is the main active ingredient obtained from the dried roots of N. chinensis. The peak of nardosinone was detected in the retention time of 5.051 min and it was also observed in the extract of N. chinensis with same retention time ().
Figure 1. Identification of nardosinone in water extract of N. chinensis. (A) The peak of nardosinone was detected in the retention time 5.051 min and (B) it was also observed in the extract of N. chinensis with same retention time.
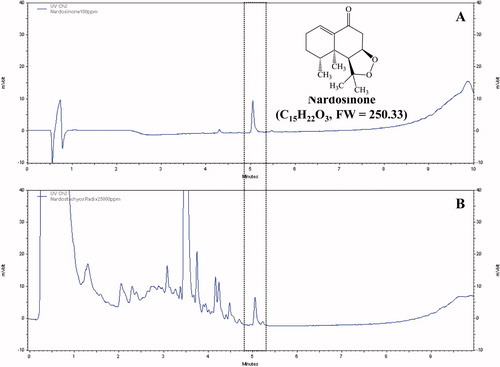
The cytotoxic effect of N. Chinensis was evaluated by the MTT assay using HL-60 cells. The cells were treated with different concentrations of N. chinensis for 72 h. As shown in , N. Chinensis reduced the viability of cells in a dose-dependent manner. The concentration required to inhibit growth of HL-60 cells by 50% (IC50) was approximately 100 μg/ml.
Figure 2. The effect of N. chinensis on cytotoxicity in HL-60 cells. The cells were treated with various concentrations of N. chinensis for 72 h and the cells were tested for viability by MTT assay. Values are means ± SD, N = 3. The statistically significant differences compared with two groups were calculated by Student's t-test. *p < 0.05 versus the untreated control group.
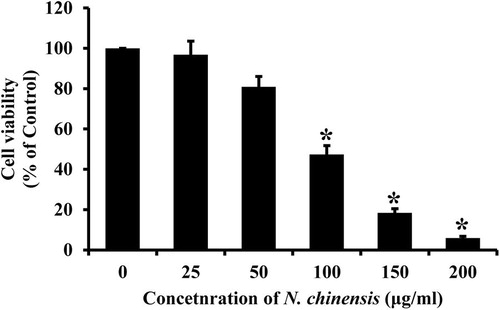
Nardostachys chinensis induces differentiation of HL-60 cells
Initially, we examined whether N. chinensis could induce a cellular differentiation in HL-60 cells. The cells were treated with N. chinensis or ATRA (served as a positive control of differentiation) for 72 h, and then the numbers of differentiated cells were measured using NBT-reducing activity assay. As shown in , when HL-60 cells were treated with N. chinensis at concentrations of 50 and 100 μg/ml, NBT-reducing activity was significantly increased; being approximately 1.5-fold at 50 μg/ml and 15.0-fold at 100 μg/ml. Moreover, the differentiation-inducing activity of N. chinensis was comparable with that of ATRA (). Next, the induction of HL-60 cell differentiation was also assessed by examining the expression of cell surface antigens (e.g., CD11b and CD14). The CD11b (FITC-labeled) expression was used as a marker of granulocytic differentiation, while CD14 (RPE-labeled) expression was only found in monocytic differentiation. After HL-60 cells were incubated with or without 100 μg/ml N. chinensis for 72 h, cell surface markers were immunolabeled and measured by a flow cytometry. As shown in , in comparison with the untreated cells, the amount of CD11b-positive cells in N. chinensis-treated cells was increased significantly (60.87%). However, the expression of monocytic CD14 antigen was not significantly increased. These results suggest that N. chinensis has the ability to induce granulocytic differentiation of HL-60 cells.
Figure 3. The differentiation-inducing effect of N. chinensis in HL-60 cells. The cells were treated with or without 100 μg/ml of N. chinensis for 72 h. Differentiation was assessed by evaluating the extent of CD11b and CD14 expression using a flow cytometry.
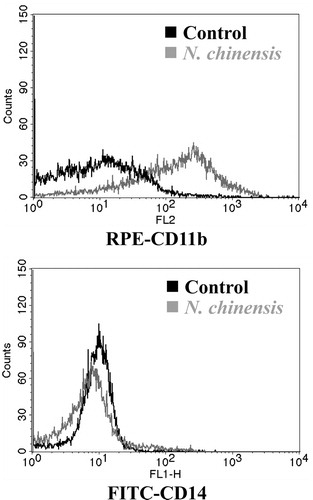
Table 1. The effect of N. chinensis on proliferation and differentiation of HL-60 cells.
Nardostachys chinensis induces cell-cycle arrest in HL-60 cells
The effect of N. chinensis on the cell-cycle progression in HL-60 cells was determined by flow cytometry. ATRA, a differentiating agent, inhibits cell proliferation and also arrests leukemic cells in G0/G1 phase (Gezer et al., Citation1988; Hui & Yung, Citation1993). The maintenance of G0/G1 phase might be necessary for granulocytic differentiation of HL-60 cells (Gezer et al., Citation1988; Hui & Yung, Citation1993). HL-60 cells were incubated with or without 100 μg/ml N. chinensis for 72 h, and analyzed by a flow cytometry after PI staining. As shown in , the cell population in G0/G1 phase of N. chinensis-treated cells was increased from 32.71% to 42.26%, with a significant decrease in cell population in G2/M and S phases. These results indicate that N. chinensis is capable of inducing a G0/G1 phase arrest of cell cycle in HL-60 cells.
Figure 4. The effect of N. chinensis on the DNA content and expression of p27Kip1 protein in HL-60 cells. (A) The cells were exposed to 100 μg/ml of N. chinensis for 72 h, washed, and then harvested. Cells were fixed and stained with PI, and the DNA content was analyzed by a flow cytometry. (B) The cells were harvested at the indicated times after incubation with 100 μg/ml of N. chinensis. Cells were lysed, and the supernatants were subjected to Western blot analysis using anti-p27Kip1 antibody. The density of band was quantitated by densitometry using Bio-Rad Quantity One software (Bio-Rad, Hercules, CA). Values are means ± SD, N = 3. *p < 0.05 versus the untreated control group.
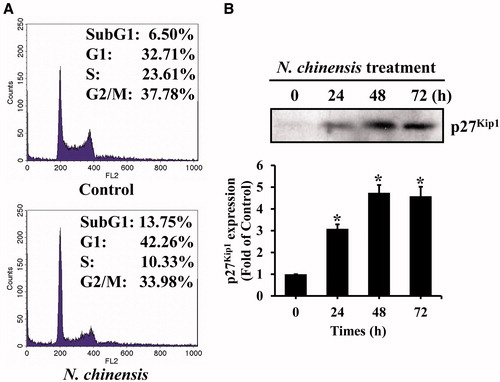
Because N. chinensis induced a G0/G1 arrest in HL-60 cells, we subsequently examined the intracellular change in the p21WAF1/Cip1 and p27Kip1 proteins, which are the CDKIs related with the G1 phase arrest (el-Deiry et al., Citation1994; Harvat et al., Citation1997; Sheaff et al., Citation1997; Xiong et al., Citation1993). The p21WAF1/Cip1 protein acts as a downstream mediator of the tumor suppressor protein p53 that functions as the G1 phase checkpoint, resulting in G1 arrest (el-Deiry et al., Citation1994; Xiong et al., Citation1993). The p27Kip1, a member of a family of proteins that can include p21WAF1/Cip1 and p57Kip2, is a universal CDK inhibitor that negatively regulates G1 CDKs (Harvat et al., Citation1997; Sheaff et al., Citation1997). After N. chinensis treatment of HL-60 cells, the p27Kip1 protein level was increased in a time-dependent manner, whereas no detectable change was observed in the level of the p21WAF1/Cip1 (). The level of p27Kip1 began to be increased slightly after 24 h incubation with N. chinensis and had been clearly increased at 48 h. These results suggested that induction of p27Kip1 protein, but not p21WAF1/Cip1, was involved in G0/G1 phase arrest of N. chinensis-treated HL-60 cells.
We next examined time-dependent changes in the levels of G1 cell-cycle regulatory proteins in N. chinensis-treated HL-60. The CDK4/6-cyclin D and CDK2-cyclin E complexes play important regulatory roles in G1 phase cell-cycle progression (Seo et al., Citation2004). The CDK4/6-cyclin D complexes are involved in early G1 phase, and CDK2-cyclin E complex is necessary for the G1/S phase transition (Seo et al., Citation2004). HL-60 cells were treated with 100 μg/ml N. chinensis for 24 h, 48 h, and 72 h, and then harvested for Western blotting analysis. The protein level of CDK2 was slightly decreased, whereas the levels of CDK4, CDK6, cyclin D1, cyclin D2, cyclin E, and cyclin A were markedly decreased (). Interestingly, the steady-state levels of cyclin D2 was unaffected (data not shown). These results imply that the decreased expression of CDK2, CDK4, CDK6, cyclin D1, cyclin D3, cyclin E, and cyclin A proteins may be responsible for the N. chinensis-induced G0/G1 phase arrest in HL-60 cells.
Figure 5. The effect of N. chinensis on the expression of the cell cycle-related proteins in HL-60 cells. The cells were harvested at the indicated times after incubation with 100 μg/ml of N. chinensis. Cells were lysed, and the supernatants were subjected to Western blot analysis using anti-CDK2, anti-CDK4, anti-CDK6, anti-cyclin D1, anti-cyclin D3, anti-cyclin E, and anti-cyclin A antibodies. The density of band was quantitated by densitometry using Bio-Rad Quantity One software (Bio-Rad, Hercules, CA). Values are means ± SD, N = 3. *p < 0.05 versus the untreated control group.
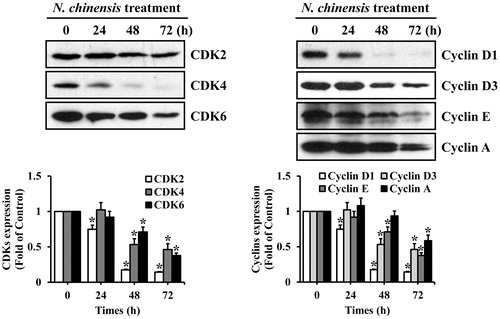
The p27Kip1 protein associates with G1-specific cyclin–CDK complexes in N. chinensis-treated HL-60 cells
The increased p27Kip1 protein inhibits the kinase activity through the formation of cyclin–CDK complexes, which is necessary for cell-cycle progression (Harvat et al., Citation1997; Sheaff et al., Citation1997). Thus, we examined the amount of p27Kip1 associated with cyclin–CDK complexes. The CDK2, CDK4, and CDK6 complexes were immunoprecipitated from the HL-60 cells, which were treated with or without N. chinensis, and the co-immunoprecipitated p27Kip1 level in the immune complex was determined by Western blot analysis using anti-p27Kip1 monoclonal antibody. As shown in , p27Kip1 level in the CDK2 immune complex of the N. chinensis-treated cells was distinctively higher than in that of the untreated cells, whereas p27Kip1 level in the CDK6 immune complex was slightly detected. In contrast, the CDK4 immune complex exhibited no significant difference in p27Kip1 level between N. chinensis-treated and -untreated cells. Taken together, these results suggested that p27Kip1 protein might play a key role in G1 phase arrest, presumably through its increased binding to CDK2 and CDK6 in N. chinensis-treated HL-60 cells.
Figure 6. Association of p27Kip1 with CDKs in HL-60 cells. The cells were treated with (+) or without (−) N. chinensis at a dose 100 μg/ml for 48 h. The total lysates were immunoprecipitated using anti-CDK2, anti-CDK4, and anti-CDK6 antibodies. The density of band was quantitated by densitometry using Bio-Rad Quantity One software (Bio-Rad, Hercules, CA). The relative density of p27Kip1 to CDKs was compared. Values are means ± SD, N = 3. *p < 0.05 versus the untreated control group.
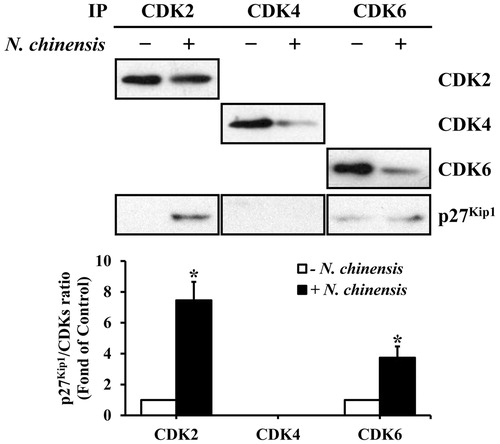
Discussion
This study demonstrates that N. chinensis induces the cell-cycle arrest in the HL-60 cells, thereby leading to cell growth inhibition and differentiation induction. The effect of N. chinensis on differentiation-inducing activity in HL-60 cells was confirmed with functional NBT reduction activity as well as expression of cell surface antigens. According to our results, treatment of HL-60 cells with N. chinensis resulted in significant increase in NBT reducing activity and expression of cell surface antigen CD11b, suggesting that N. chinensis may have its ability to induce granulocytic differentiation.
Generally, cell differentiation is regulated in a cell cycle-dependent manner. For example, the differentiation of hematopoietic cells is strongly associated with a significant loss of cell-cycling capacity, and the cells, as such a result, become arrested in the G0/G1 phase of the cell cycle (Furukawa et al., Citation1992). In this study, the cell-cycle analysis revealed that N. chinensis could markedly induce a G0/G1 phase arrest in HL-60 cells, along with a significant reduction in G2/M and S phases. It has been demonstrated that inhibition of the G1/S transition can induce growth arrest and granulocytic differentiation of HL-60 cells, which is mediated through blockage of cell-cycle progression at the G0/G1 phase (Cooper, Citation2001; Sakashita et al., Citation1991). The p21WAF1/Cip1 and p27Kip1 proteins are cyclin-dependent kinase inhibitors that are involved in regulation of cell-cycle progression in the G1/S transition (Wang et al., Citation1996). Here, we have demonstrated that treatment of HL-60 cells with N. chinensis resulted in increased expression of p27Kip1 protein, with no significant expression of p21WAF1/Cip1 protein. Thus, it is most likely that the expression of p27Kip1 protein by N. chinensis is important for the G0/G1 phase arrest and granulocytic differentiation in HL-60 cells.
Among the CDKs that regulate the cell cycle, CDK2, CDK4, and CDK6 are activated in association with the D-type cyclins or cyclin E during the G1 progression and the G1–S transition (Furukawa et al., Citation1992). This study also revealed that the CDK2, CDK4, CDK6, cyclin D1, cyclin D3, cyclin E, and cyclin A were significantly reduced in the N. chinensis-treated HL-60 cells. Moreover, the accumulation of the p27Kip1 protein in association with the G1 arrest was detected in the complexes with CDK2 and CDK6. Because the interaction between the CDK2/CDK6 and the p27Kip1 was associated with suppressed cyclin/CDK activity, the decreased protein levels of CDK2, CDK4, CDK6, cyclin D1, cyclin D3, cyclin E, and cyclin A, and the reduced kinase activities of CDK2 and CDK6 might be responsible for G0/G1 phase cell-cycle arrest in N. chinensis-treated HL-60 cells. It has been demonstrated that ATRA-induced terminal differentiation of leukemia cells involves the sequential regulation of cell-cycle regulatory genes, coordinating the process of differentiation with arrest in the G0/G1 phase of the cell cycle (Dimberg & Oberg, Citation2003); namely G0/G1 phase arrest of the cell cycle is a hallmark of terminally differentiation in leukemia cells.
Conclusions
The medicinal plant N. chinensis is capable of inducing granulocytic differentiation and growth inhibition through p27Kip1 protein-related G0/G1 phase arrest in HL-60 cells. Based on these results, N. chinensis may be a new potent inducer for differentiation of the HL-60 human leukemia cells into granulocyte. Further studies are necessary to fractionate the active components from N. chinensis and to elucidate the exact mechanism(s) for N. chinensis-induced differentiation of HL-60 cells.
Declaration of interest
The authors declare that there is no conflict of interests regarding the publication of this article.
Acknowledgements
This paper was supported by Wonkwang University in 2013.
References
- Aksentijevich I, Flinm IW. (2003). Chronic lymphocytic leukemia: Advances in biology and therapeutics. Curr Opin Oncol 15:16–22
- Baj G, Arnulfo A, Deaglio S, et al. (2000). Retinoids in breast cancer prevention and treatment. A review of the literature. Eur J Gynaecol Oncol 21:411–15
- Breitman TR, Selonick SE, Collins SJ. (1980). Induction of differentiation of the human promyelocytic leukemia cell line (HL-60) by retinoic acid. Proc Natl Acad Sci USA 77:2936–40
- Brugarolas J, Chandrasekaran C, Gordon JI, et al. (1995). Radiation-induced cell cycle arrest compromised by p21 deficiency. Nature 377:552–7
- Collins SJ, Ruscetti FW, Gallagher RE, Gallo RC. (1978). Terminal differentiation of human promyelocytic leukemia cells induced by dimethyl sulfoxide and other polar compounds. Proc Natl Acad Sci USA 75:2458–62
- Cooper S. (2001). Revisiting the relationship of the mammalian G1 phase to cell differentiation. J Theor Biol 208:399–402
- Covacci V, Bruzzese N, Sgambato A, et al. (1998). Magnesium restriction induces granulocytic differentiation and expression of p27Kip1 in human leukemic HL-60 cells. J Cell Biochem 70:313–22
- Dimberg A, Oberg F. (2003). Retinoic acid-induced cell cycle arrest of human myeloid cell lines. Leuk Lymphoma 44:1641–50
- el-Deiry WS, Harper JW, O'Connor PM, et al. (1994). WAF1/CIP1 is induced in p53-mediated G1 arrest and apoptosis. Cancer Res 54:1169–74
- Elledge SJ, Harper JW. (1994). Cdk inhibitors: On the threshold of checkpoints and development. Curr Opin Cell Biol 6:847–52
- Furukawa Y, Uenoyama S, Ohta M, et al. (1992). Transforming growth factor-beta inhibits phosphorylation of the retinoblastoma susceptibility gene product in human monocytic leukemia cell line JOSK-I. J Biol Chem 267:17121–7
- Gezer S, Yasin Z, Imren S, et al. (1988). Changes observed in the growth fraction, labeling index, duration of S phase, and total cell cycle times of HL-60 cells as they undergo differentiation in response to retinoic acid. Cancer Res 48:5989–94
- Harvat BL, Seth P, Jetten AM. (1997). The role of p27Kip1 in gamma interferon-mediated growth arrest of mammary epithelial cells and related defects in mammary carcinoma cells. Oncogene 14:2111–22
- Huberman E, Callaham MF. (1979). Induction of terminal differentiation in human promyelocytic leukemia cells by tumor-promoting agents. Proc Natl Acad Sci USA 76:1293–7
- Hui EK, Yung BY. (1993). Cell cycle phase-dependent effect of retinoic acid on the induction of granulocytic differentiation in HL-60 promyelocytic leukemia cells. Evidence for sphinganine potentiation of retinoic acid-induced differentiation. FEBS Lett 318:193–9
- Itokawa H, Masuyama K, Morita H, Takeya K. (1993). Cytotoxic sesquiterpenes from Nardostachys chinensis. Chem Pharm Bull (Tokyo) 41:1183–4
- Li P, Matsunaga K, Yamakuni T, Ohizumi Y. (2003). Nardosinone, the first enhancer of neurite outgrowth-promoting activity of staurosporine and dibutyryl cyclic AMP in PC12D cells. Brain Res Dev Brain Res 145:177–83
- Lo Coco F, Zelent A, Kimchi A, et al. (2002). Progress in differentiation induction as a treatment for acute promyelocytic leukemia and beyond. Cancer Res 62:5618–21
- Myers CE. (1999). Differentiating agents and nontoxic therapies. Urol Clin North Am 26:341–51
- Parmar S, Tallman MS. (2003). Acute promyelocytic leukemia: A review. Exp Opin Pharmacother 4:1379–92
- Patnaik A, Rowinsky EK, Villalona MA, et al. (2002). A phase I study of pivaloyloxymethylbutyrate, a prodrug of the differentiating agent butyric acid, in patients with advanced solid malignancies. Clin Cancer Res 8:2142–8
- Peter M, Herskowitz I. (1994). Joining the complex: Cyclin-dependent kinase inhibitory proteins and the cell cycle. Cell 79:181–4
- Rovera G, Santoli D, Damsky C. (1979). Human promyelocytic leukemia cells in culture differentiate into macrophage-like cells when treated with a phorbol diester. Proc Natl Acad Sci USA 76:2779–83
- Sakashita A, Nakamaki T, Tsuruoka N, et al. (1991). Granulocyte colony-stimulating factor, not granulocyte-macrophage colony-stimulating factor, co-operates with retinoic acid on the induction of functional N-formyl-methionyl-phenylalanine receptors in HL-60 cells. Leukemia 5:26–31
- Sciarra A, Casale P, Di Chirio C, et al. (1998). New aspects on prostate cancer: Hereditary form, developmental estrogenization and differentiation therapy. Minerva Urol Nefrol 50:185–90
- Seo BI, Lee JH, Choi HY, et al. (2008). Korean Medicine Herbology. Seoul: Younglim Press, 496–7
- Seo BR, Lee KW, Ha J, et al. (2004). Saucernetin-7 isolated from Saururus chinensis inhibits proliferation of human promyelocytic HL-60 leukemia cells via G0/G1 phase arrest and induction of differentiation. Carcinogenesis 25:1387–94
- Sheaff RJ, Groudine M, Gordon M, et al. (1997). Cyclin E-CDK2 is a regulator of p27Kip1. Genes Dev 11:1464–78
- Sherr CJ, Roberts JM. (1995). Inhibitors of mammalian G1 cyclin-dependent kinases. Genes Dev 9:1149–63
- Spira AI, Carducci MA. (2003). Differentiation therapy. Curr Opin Pharmacol 3:338–43
- Takaya Y, Takeuji Y, Akasaka M, et al. (2000). Novel guaiane endoperoxides, nardoguaianone A–D, from roots and their antinociceptive and antimalarial activities. Tetrahedron 56:7673–8
- Walczak J, Wood H, Wilding G, et al. (2001). Prostate cancer prevention strategies using antiproliferative or differentiating agents. Urology 57:81–5
- Wang QM, Jones JB, Studzinski GP. (1996). Cyclin-dependent kinase inhibitor p27 as a mediator of the G1-S phase block induced by 1,25-dihydroxyvitamin D3 in HL60 cells. Cancer Res 56:264–7
- Wang Z, Sun G, Shen Z, et al. (1999). Differentiation therapy for acute promyelocytic leukemia with all-trans retinoic acid: 10-year experience of its clinical application. Chin Med J (Engl) 112:963–7
- Xiao PG. (2002). Modern Chinese Materia Medica. Beijing: Chemical Industry Press, 252–3
- Xiong Y, Hannon GJ, Zhang H, et al. (1993). p21 is a universal inhibitor of cyclin kinases. Nature 366:701–4
- Yang Q, Sakurai T, Kakudo K. (2002). Retinoid, retinoic acid receptor beta and breast cancer. Breast Cancer Res Treat 76:167–73