Abstract
Context: Melatonin, a pineal hormone and a potent antioxidant, has important roles in metabolic regulation.
Objective: This study investigated serum asymmetric dimethylarginine (ADMA), homocysteine (Hcy), nitric oxide (NO) levels, known to be reliable markers of cardiovascular diseases, and determined possible protective effects of melatonin in fructose-fed rats.
Materials and methods: Sprague–Dawley rats were divided into four groups: control, fructose, melatonin, and fructose plus melatonin. Metabolic syndrome was induced in rats by 20% (w/v) fructose solution in tap water, and melatonin was administered at the dose of 20 mg/kg bw per day by oral gavage. After 8 weeks, serum lipids, glucose, insulin, ADMA, Hcy, and NOx (the stable end products of NO) levels were quantified.
Results: Fructose administration caused a statistically significant increase in systolic blood pressure (SBP), serum insulin, triglycerides, and very low-density lipoprotein (VLDL)–cholesterol levels compared with the control group and the metabolic syndrome model was successfully demonstrated. In comparison with the control group, fructose caused a significant increase in serum ADMA, Hcy, and NOx levels. Melatonin counteracted the changes in SBP, serum ADMA, and Hcy levels found in rats both alone and administered with fructose.
Discussion and conclusion: These results show that high fructose consumption leads to elevated SBP, atherogenic lipid profile, increased serum ADMA, and Hcy levels and melatonin treatment has beneficial effects on these biochemical parameters in rats. Melatonin might be beneficial for the prevention and/or treatment of the cardiovascular complications of metabolic syndrome not only by reducing the well-known risk factors of the disease but also by diminishing blood ADMA and Hcy levels.
Introduction
Metabolic syndrome diagnosis relies on the presence of at least three metabolic alterations among hypertension, insulin resistance, hyperinsulinemia, visceral obesity, dyslipidemia, and endothelial dysfunction. These multiple risk factors accelerate the development of cardiovascular diseases in a cooperative way (de Moura et al., Citation2009). There have been clinical and epidemiological evidence suggesting a progressive association of metabolic syndrome development and high-fructose consumption. Laboratory animals fed on fructose-rich diets show glucose intolerance, insulin resistance, hyperinsulinemia, and dyslipidemia (Delbosc et al., Citation2005). Insulin resistance and hyperinsulinemia impair endothelial function, thereby contributing to the elevation in the blood pressure in animal models. Vascular dysfunction due to fructose-rich diet has been reported in rats. In addition, impaired endothelium-dependent relaxation has been found to occur after fructose intake in mice (Delbosc et al., Citation2005; Glushakova et al., Citation2008). There is now evidence that insulin modulates the expression of endothelial nitric oxide synthase (eNOS) and stimulates nitric oxide (NO) production, causing vasodilation. On one hand, in the state of insulin resistance, the effects of insulin on eNOS and NO become blunted, resulting endothelial dysfunction (Assumpçao et al., Citation2008; Johnson et al., Citation2007; Marchesi et al., Citation2009). On the other hand, blood homocysteine (Hcy) and asymmetric dimethylarginine (ADMA) levels are markers of endothelial damage and subsequent cardiovascular disorders, and these parameters are linked to other metabolic syndrome symptoms (Bestermann, Citation2011). Evidence of a metabolic link between Hcy and ADMA has also been obtained from studies on animals, humans, and cell cultures. A potential interaction between Hcy and ADMA is suggested to be the metabolic relationship between ADMA and methyl transfer reactions. It has been hypothesized that increased protein methylation may result in increased production of both ADMA and Hcy (Stühlinger et al., Citation2001). Hyperhomocysteinemia has been reported to be a risk factor for cardiovascular diseases and accumulation of ADMA in endothelial cells may inhibit eNOS leading to decreased production of NO and subsequently endothelial dysfunction. The endothelium is the primary target of destruction arising from chemical and mechanical factors. Endothelial dysfunction, either as a cause or a consequence, is now known to be associated with a spectrum of cardiovascular diseases (Bestermann, Citation2011; Dayal & Lentz, Citation2005; Wilcken et al., Citation2006).
Melatonin (N-acetyl-5-methoxytryptainine) is a hormone-secreted chemical, mainly by the pineal gland, and it is involved in many physiological events in humans and animals, having antiexcitatory, antioxidant, antiinflammatory, immunomodulatory, and vasomotor effects. Melatonin is reported to have positive effects on visceral fat accumulation, dyslipidemia, and high blood pressure (Kitagava et al., Citation2012; Kozirog et al., Citation2011; Rios-Lugo et al., Citation2010). Moreover, it is known that melatonin improves glucose homeostasis and endothelial vascular function in high-fat diet-fed insulin-resistant mice (Robeva et al., Citation2008; Sartori et al., Citation2009). In recent years, many studies have examined melatonin administration with metabolic syndrome patients and metabolic syndrome models, reporting melatonin had beneficial effects on blood pressure, blood lipids, glucose, and insulin levels (Cardinali et al., Citation2013; Kozirog et al., Citation2011; Leibowitz et al., Citation2008). However, no data have yet been published regarding the effects of melatonin on blood ADMA–Hcy–NOx parameters of fructose-induced metabolic syndrome model in rats. The objective of this study was to investigate the effects of melatonin, first on metabolic syndrome criteria, and second on serum NOx, ADMA and Hcy levels in fructose-fed rats.
Materials and methods
Chemicals
Melatonin (≥98%) and d-fructose (≥99%) were purchased from Sigma-Aldrich (St. Louis, MO). All other chemicals used were of the highest analytical grade, and purchased from Merck (Darmstadt, Germany) or Sigma-Aldrich (St. Gallen, Switzerland).
Animals and experimental design
This study was carried out in accordance with the regulations of Animal Experimentation Ethics Committee of Gazi University. Thirty-two adult male Sprague–Dawley rats weighing 225 ± 10 g were obtained from Laboratory Animal Husbandry and Experimental Research Center at Gazi University. The animals were housed at 20–24 °C with a 12-h light/12-h dark cycle (the lights were off daily from 20:00 to 08:00 h), and they were provided with standard rat chow and tap water freely available.
Thirty-two rats were randomly divided into four groups (n = 8) as follows:
Control: Rats in this group received standard rodent diet and tap water.
Fructose: Rats in this group received standard rodent diet and tap water supplemented with 20% fructose (Sanchez-Lozada et al., Citation2007).
Melatonin: Rats in this group received standard rodent diet and tap water, and melatonin administered at the dose of 20 mg/kg body weight in 0.1% ethanol solution per day by oral gavage (Leibowitz et al., Citation2008). Melatonin solution was prepared freshly every day.
Fructose plus melatonin: Rats in this group received standard rodent diet and tap water supplemented with 20% fructose, and melatonin administered at the dose of 20 mg/kg body weight in 0.1% ethanol solution per day by oral gavage.
Since ethanol was used as a melatonin vehicle, control and fructose groups received 0.1% ethanol solution proportionately with body weight. The experiment was carried out for 8 weeks and at the end of the 8th week, the animals were sacrificed under ketamine (30 mg/kg bw) and xylazine (6 mg/kg bw) anesthesia. Blood samples for laboratory assays were drawn intracardiacally and sera were separated by centrifugation and stored at −80 °C until analysis.
Systolic blood pressure (SBP), body weight, food, and fluid intake
SBP were measured by the tail-cuff method at the beginning of the study, at the end of the 4th week and at the end of the 8th week. All animals were preconditioned for blood pressure measurements 1 week before each experiment. At least seven determinations were made in every session and the mean of the lowest three values within 5 mmHg was taken as the SBP value. All SBP measurements were made between 10:00 and 13:00 h. Body weights were recorded weekly, food and fluid intake of all above groups were measured daily. Caloric intake for fructose-fed rats was calculated as sum of calories ingested as food on the basis of 2.9 kcal per gram of chow consumed and on that each ingested gram of fructose corresponds to 4.0 kcal.
Biochemical assays
Serum glucose, triglyceride, total-, high-density lipoprotein (HDL), and very low-density lipoprotein (VLDL)-cholesterol levels were measured by enzymatic methods using autoanalyzers. Serum insulin level was estimated by using commercially available ELISA kit (Millipore, Billerica, MA). Insulin resistance was evaluated by the Homeostasis Model Assessment index (HOMA-IR) using the formula: [insulin (mU/L) × glucose (mmol/L)]/22.5.
Serum NOx (nitrite plus nitrate), which are known to be the end products of NO, was measured by using commercially available colorimetric kit (Cayman Chemical, Ann Arbor, MI). ADMA and Hcy levels were measured with HPLC-flourescent detection (Araki & Sako, Citation1987; Chen et al., Citation1997).
Statistical analysis
All data were expressed as mean ± standard deviation. The statistical analyses of the results were performed using a computerized statistical package (SPSS 16.0 for windows, SPSS Inc., Chicago, IL). Each mean value was compared by one-way analysis of variance (ANOVA) and Tukey for multiple comparisons. All statistical tests were two-tailed, and p < 0.05 was considered statistically significant.
Results
SBP, body weight, food, and fluid intake
Water and food consumptions, body weights, and SBP are shown in . The fructose group body weights were higher compared with the control group; however, this was not statistically significant (). Water consumptions of fructose and fructose plus melatonin groups were approximately 10 ml more than control and melatonin groups, which was a significant difference (). Solid food intake in fructose-treated groups was significantly lower compare with control and melatonin groups. In addition, total caloric intake in fructose-treated groups was significantly higher compare with control and melatonin groups. Fructose administration significantly elevated SBP, compared with the control group. SBP showed a rise by the values of 30 mmHg and 7 mmHg at the end of 4th week and at the end of 8th week, respectively, which was found to be statistically significant (p < 0.05). In comparison with the fructose group, melatonin administration significantly reduced SBP to control levels in the fructose plus melatonin group (p < 0.05, ).
Figure 2. Weekly water consumption (ap < 0.05, compared with the control group; bp < 0.05, compared with the fructose group; cp < 0.05, compared with the melatonin group).
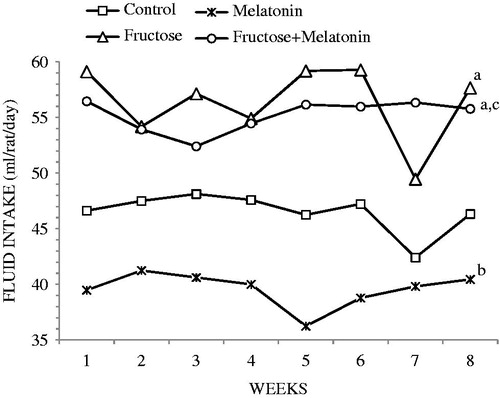
Table 1. Body weight, fluid, food, and calorie intake.
Table 2. Systolic blood pressures.
Serum lipids
shows the serum lipid profile. Compared with the control group, the fructose group showed a significant increase in serum triglyceride and VLDL-cholesterol levels (p < 0.05) without changes in total and HDL-cholesterol levels. Serum triglyceride and VLDL-cholesterol levels of the fructose plus melatonin group were lower than the fructose group; however, these moderations did not reach statistical significance.
Table 3. Serum lipid profile.
Serum glucose, insulin levels, and insulin resistance
As shown in , a significant difference between glucose levels of control and fructose groups could not be found. Serum glucose levels were not affected by melatonin. Serum insulin levels and HOMA-IR indexes for insulin resistance of the fructose group were significantly higher compared with control (p < 0.05). Compared with the fructose group, serum insulin levels and insulin resistance were significantly retreated in the fructose plus melatonin group; however, they did not reach control levels.
Table 4. Serum glucose, insulin, and HOMA-IR levels.
Serum NOx, ADMA, and Hcy levels
Fructose administration significantly enhanced serum NOx, ADMA, and Hcy levels (p < 0.05). In the fructose plus melatonin group, melatonin treatment significantly attenuated ADMA and Hcy levels, while the decrease in NOx was not statistically significant ().
Table 5. Serum NOx, ADMA, and Hcy levels.
Discussion
Metabolic syndrome, also called the syndrome X or insulin resistance syndrome, is a cluster of pathologies comprising insulin resistance, hyperinsulinemia, hypertriglyceridemia, and hypertension. Nowadays, it is known that one of the main reasons of increasing incidence of metabolic syndrome is high-fructose consumption in diet (Hanson et al., Citation2002). While fructose is also a simple sugar, like glucose, there are emerging data from many animal and human studies showing that fructose is highly lipogenic and plays important roles in the development of metabolic syndrome and related complications (Miller & Adeli, Citation2008). Previous studies showed that fructose-fed rat models develop insulin resistance syndrome with a very similar metabolic profile to humans (Johnson et al., Citation2007). Thus, high-fructose feeding is widely used to induce the alterations which take place in metabolic syndrome and to investigate the development mechanisms of related pathologies and search for possible treatment methods (Dai & McNeill, Citation1995).
In the present study, we used male Sprague–Dawley rats and fructose administration was accomplished by giving daily prepared 20% d-fructose solution in tap water for 8 weeks. At the end of the study, fructose induced hypertension, hyperinsulinemia, insulin resistance, and hypertriglyceridemia in which metabolic syndrome criteria were observed compared with the control group. However, serum glucose, total, and HDL-cholesterol levels did not change. Our study and other experimental animal models demonstrated an association between fructose consumption and hypertension, insulin resistance, and high plasma triglycerides levels (Castro et al., Citation2013; Giani et al., Citation2009; Miatello et al., Citation2005; Reddy et al., Citation2009). Numerous mechanisms have been suggested to explain this phenomenon. Fructose metabolism is known to be an insulin-independent mechanism, considering fructose uptake is not insulin dependent, unlike glucose, and fructose bypasses one of the rate limiting steps of glycolytic pathway which is catalyzed by phosphofructokinase. Thereby, fructose entrance to liver cells does not prevent further glucose uptake. Fructose and glucose can enter the glycolytic pathway and as their intermediates accumulate, the intermediates can be converted to glycerol 3-phosphate, which is the source of glycerol in triglyceride molecule. Furthermore, as a result of pyruvate conversion to acetyl CoA, long-chain fatty acids that can be esterified to form triglycerides are produced, and subsequently hypertriglyceridemia arises (Elliot et al., Citation2002; Tran et al., Citation2009). An increased triglyceride level leads to increased free fatty acid level. Free fatty acid oxidation impairment, possibly as a result of the defects in insulin receptor activity and/or changes in the insulin effect at post-receptor signalling, leads to insulin resistance (Bezerra et al., Citation2000). Hyperinsulinemia inhibits glucose oxidation and glycogen synthesis in skeletal muscle and, therefore, glucose intolerance arises. In addition, high-fructose consumption also promotes insulin resistance by altering functions of the enzymes that regulate hepatic carbohydrate metabolism. These alterations include decreased glucokinase and increased glucose 6-phosphatase activities (Delbosc et al., Citation2005; Kim et al., Citation2010). Insulin resistance/hyperinsulinemia and hypertension is also associated with continuous activation of the sympathetic nervous system, resulting in increased production and activity of vasoconstrictors such as endothelin-I, angiotensin-II, thromboxane A2, and, therefore, impaired endothelium-dependent relaxation. Each of the proposed mechanisms contributes to elevated vascular tone and blood pressure (Kamata & Yamashita, Citation1999; Scherrer et al., Citation1994; Verma et al., Citation1997).
In our study, in addition to high-fructose feeding, we treated rats with 20 mg/kg/d melatonin for 8 weeks and aimed to investigate the possible protective effects of melatonin. From our results, it is seen that melatonin significantly prevented SBP rise, hyperinsulinemia, and insulin resistance which were caused by high-fructose consumption. However, melatonin treatment did not have a positive effect on serum triglyceride and VLDL–cholesterol levels. Previous studies proposed several mechanisms to explain the blood pressure lowering effect of melatonin, such as sympathetic nervous system depression, anti-adrenergic effects, and effects on NO bioavailability (Leibowitz et al., Citation2008; Nduhirabandi et al., Citation2012). Melatonin is a hormone which is closely related to the regulation of insulin secretion and glucose/lipid metabolism. Melatonin showed positive impacts on metabolic syndrome due to its anti-hyperlipidemic effect and it is reported to reduce adiposity and body weight in animal studies (Chan & Tang, Citation1995; Srinivasan et al., Citation2013; Zanuto et al., Citation2013). Moreover, melatonin was showed to increase insulin sensitivity and glucose tolerance in rats fed with high fat or sucrose diets (Srinivasan et al., Citation2013). On a cellular level, insulin resistance is associated with abnormal or deranged intracellular insulin signalling cascade. The role of melatonin on insulin resistance can be understood better in pinealectomised animal studies as insulin resistance and glucose intolerance is induced in these animals. Melatonin therapy was reported to induce glucose transport in skeletal muscle via phosphorilation and activation of the molecules involved in the signalling cascade. In addition, it was demonstrated to improve glucose homeostasis by restoring the vascular effect of insulin (Nduhirabandi et al., Citation2012). Melatonin also carries out its regulatory effect on insulin levels by inhibiting insulin release via effecting MT1 and MT2 melatonin receptors found in pancreatic β-cells (Muhlbauer et al., Citation2012).
Results of a study similar to ours, conducted by Cardinali et al. (Citation2013), are consistent with our findings. They have found that treatment with 25 µg/ml melatonin in drinking water significantly lowered SBP of Wistar rats which were given 5% or 10% fructose in drinking water and the researchers suggested that melatonin might be a strong therapeutic agent for metabolic syndrome treatment. With the intend of investigating the effect of melatonin on hypertension pathogenesis, Leibowitz et al. (Citation2008) supplemented 30 mg/kg melatonin via drinking water to the rats which have fructose-induced metabolic syndrome and reported that melatonin supplementation significantly reduced SBP. There is also clinical trial evidence supporting our findings, such as the Kozirog et al. (Citation2011) study. They claimed that 5 mg/d melatonin supplementation to 30 patients for 2 months not only lowered SBP but also fixed the blood lipid profile and they concluded that melatonin supplementation might be beneficial for especially the prevention of arterial hypertension in metabolic syndrome patients.
In the present study, serum NOx, Hcy, and ADMA levels were also increased in fructose-fed rats. Melatonin treatment decreased serum ADMA and Hcy levels in both melatonin and fructose plus melatonin groups compared with the fructose group. Moreover, ADMA levels were even lower than that of the control group. Hcy levels of the fructose plus melatonin group was the same as the control group. On one hand, ADMA is a competitive endogenous inhibitor of all NOS isoforms and, at physiological concentrations, it can be considered as an endogenous regulator of arterial tone by eNOS inhibition. On the other hand, increased plasma concentrations of ADMA were found to cause an increase in superoxide () production via the activation of the renin–angiotensin system and NADPH oxidase, and thereby lead to oxidative stress in smooth muscle cells and cause vasoconstriction by the impairing NO bioavailability. Hence, elevated plasma concentrations of ADMA represent a novel risk factor for the development of endothelial dysfunction and a predictor for all-cause and cardiovascular mortality (Ersahin et al., Citation2009; Sydow & Münzel, Citation2003).
Hcy is a non-protein, sulfur-containing amino acid and is a product of methionine metabolism, and likewise, increased levels of Hcy are a major risk factor for vascular diseases. In Hcy-induced endothelial dysfunction, the principle mechanism is related to auto-oxidation and free radical production. Hcy can easily be auto-oxidized and homocystine, Hcy-mixed disulfides, and Hcy thiolactone are produced. Hydrogen peroxide and are produced during the oxidation of sulfydryl groups and these oxygen-derived molecules are believed to play a role in endothelial cytotoxicity of Hcy. Hcy is shown to reduce endothelium derived NO bioavailability without changing eNOS activity in in vivo and in vitro studies. This process was mediated by the reaction between
which was formed as a result of its auto-oxidation, and NO. By means of this reaction, Hcy inhibits NO via peroxynitrite (ONOO−) formation and thus weakens vascular relaxation (Murawska-Cialowicz et al., Citation2008; Okatani et al., Citation2000). Neither the driving factor for ADMA and Hcy increase in metabolic syndrome nor the effect of fructose on these parameters is fully clear. However, it is shown that high levels of plasma ADMA were correlated with insulin resistance and hypertriglyceridemia in metabolic syndrome (Bestermann, Citation2011). In addition, the association between plasma ADMA and Hcy levels is observed in many animal and clinical studies. High Hcy concentrations might elevate ADMA by inhibiting dimethylarginine dimethylaminohydrolase (DDAH), the ADMA metabolizing enzyme, activity (Dayal & Lentz, Citation2005; Stühlinger et al., Citation2001; Wilcken et al., Citation2006).
In previous studies, NO production and blood flow were reported to show a tendency to decrease in insulin resistance and in high levels of ADMA (Delbosc et al., Citation2005); however, in our study, serum NOx levels were found to be elevated together with ADMA and Hcy. On one hand, our results seem to be in conflict with some other results, while on the other hand, some previous observations support us regarding the NOx amount. In various studies, it is claimed that Hcy and/or ADMA causes uncoupling of eNOS and causes the enzyme to produce rather than NO. The production of
may contribute to the oxidative inactivation of endothelium-derived NO, by converting it to ONOO−. ONOO− and notably its conjugated peroxynitrous acid are highly unstable in aqueous phase, and they isomerize and decompose to nitrate and nitrite (Assumpçao et al., Citation2008; Bestermann, Citation2011; Dayal & Lentz, Citation2005; Dayal et al., Citation2004). With regard to these data, endothelium-dependent vasodilatation might be decreased due to decreased bioavailability of NO and levels of NO metabolites might be raised due to oxidative stress.
In our study, serum Hcy concentrations were also decreased compared with the fructose group after melatonin administration when used alone or together with fructose. Melatonin administration also significantly diminished the increased ADMA levels caused by fructose. Melatonin is an effective scavenger of oxygen and nitrogen-derived free radicals, such as hydroxyl radical (OH), singlet oxygen, peroxyl radical, and ONOO−. In addition, it has stimulatory effects on antioxidant enzymes, such as glutathione peroxidase and superoxide dismutase. By means of these features, it hinders Hcy-induced endothelial damage and vasospastic effects via scavenging the free radicals, especially. OH, without inhibiting Hcy auto-oxidation (Okatani et al., Citation2000, Citation2001). Melatonin’s diminishing effects on increased Hcy levels might be related to the regulatory roles in its metabolism or limiting its synthesis (Murawska-Cialowicz et al., Citation2008). In various experimental models, it is shown that melatonin reduced plasma ADMA levels by preventing oxidative stress-induced reduction of DDAH activity (Tain et al., Citation2010a,Citationb,Citationc). It is reported that melatonin administration to hypertensive rats refined increased ADMA levels and decreased plasma NO levels, reduced blood pressure by probably either directly inhibiting ADMA production or restoring NO pathway, thus provided endothelial tissue homeostasis (Ersahin et al., Citation2009; Tain et al., Citation2010a).
In conclusion, we implemented three important criteria of metabolic syndrome, which are hypertension, hypertriglyceridemia, and hyperinsulinemia/insulin resistance by 20% fructose administration in drinking water for 8 weeks. In addition to this, serum ADMA and Hcy levels, which are also important risk factors for cardiovascular diseases, were found to be significantly elevated. Administration of 20 mg/kg/d melatonin was effective for prevention of fructose-induced hypertension and insulin resistance and the treatment also decreased serum ADMA and Hcy levels. Melatonin can be useful for the prevention/treatment of the cardiovascular complications of metabolic syndrome by its beneficial effects not only on the well-known criteria of the disease but also on the reduction of plasma ADMA and Hcy levels.
Declaration of interest
The authors declare that they have no conflict of interest. This study was supported by Gazi University, Department of Scientific Research Projects Unit (Project no. 01/2010–17). The authors thank TUBITAK (The Scientific and Technological Research Council of Turkey) for the fellowship to F. S. B.
References
- Araki A, Sako Y. (1987). Determination of free and total homocysteine in human plasma by high-performance liquid chromatography with fluorescence detection. J Chromatogr 422:43–52
- Assumpçao CR, Brunini TMC, Matsuura C, Mendes-Riberio AC. (2008). Impact of the l-arginine-nitric oxide pathway and oxidative stress on the pathogenesis of the metabolic syndrome. Open Biochem J 2:108–15
- Bestermann WH. (2011). The ADMA–metformin hypothesis: Linking the cardiovascular consequences of the metabolic syndrome and type II diabetes. Cardiorenal Med 1:211–19
- Bezerra RM, Ueno M, Silva MS, et al. (2000). A high fructose diet affects the early steps of insulin action in muscle and liver of rats. J Nutr 130:1531–5
- Cardinali DP, Bernasconi PA, Reynoso R, et al. (2013). Melatonin may curtail the metabolic syndrome: Studies on initial and fully established fructose-induced metabolic syndrome in rats. Int J Mol Sci 14:2502–14
- Castro MC, Massa ML, Schinella G, et al. (2013). Lipoic acid prevents liver metabolic changes induced by administration of a fructose-rich diet. Biochim Biophys Acta 1830:2226–32
- Chan TY, Tang PL. (1995). Effect of melatonin on the maintenance of cholesterol homeostasis in the rat. Endocr Res 21:681–96
- Chen BM, Xia LW, Zhao RQ. (1997). Determination of N(G),N(G)-dimethylarginine in human plasma by high-performance liquid chromatography. J Chromatogr B Biomed Sci Appl 692:467–71
- Dai S, McNeill JH. (1995). Fructose-induced hypertension in rats is concentration and duration dependent. J Pharmacol Toxicol Methods 33:101–7
- Dayal S, Lentz SR. (2005). ADMA and hyperhomocysteinemia. Vasc Med 10:S27–33
- Dayal S, Arning E, Bottiglieri T, et al. (2004). Cerebral vascular dysfunction mediated by superoxide in hyperhomocysteinemic mice. Stroke 35:1957–62
- de Moura RF, Ribeiro C, de Oliveira JA, et al. (2009). Metabolic syndrome signs in Wistar rats submitted to different high fructose ingestion protocols. Br J Nutr 101:1178–84
- Delbosc S, Paizanis E, Magous R, et al. (2005). Involvement of oxidative stress and NADPH oxidase activation in the development of cardiovascular complications in a model of insulin resistance, the fructose-fed rat. Atherosclerosis 179:43–9
- Elliott SS, Keim NL, Stern JS, et al. (2002). Fructose, weight gain, and the insulin resistance syndrome. Am J Clin Nutr 76:911–22
- Ersahin M, Sehirli O, Toklu HZ, et al. (2009). Melatonin improves cardiovascular function and ameliorates renal, cardiac and cerebral damage in rats with renovascular hypertension. J Pineal Res 47:97–106
- Giani JF, Mayer MA, Munoz MC, et al. (2009). Chronic infusion of angiotensin-(1–7) improves insulin resistance and hypertension induced by a high-fructose diet in rats. Am J Physiol Endocrinol Metab 296:E262–71
- Glushakova O, Kosugi T, Roncal C, et al. (2008). Fructose induces the inflammatory molecule ICAM-1 in endothelial cells. J Am Soc Nephrol 19:1712–20
- Hanson RL, Imperatore G, Bennett PH, Knowler WC. (2002). Components of the metabolic syndrome and incidence of type 2 diabetes. Diabetes 51:3120–7
- Johnson RJ, Segal MS, Sautin Y, et al. (2007). Potential role of sugar (fructose) in the epidemic of hypertension, obesity and the metabolic syndrome, diabetes, kidney disease and cardiovascular disease. Am J Clin Nutr 86:899–906
- Kamata K, Yamashita K. (1999). Insulin resistance and impaired endothelium-dependent renal vasodilatation in fructose-fed hypertensive rats. Res Commun Mol Pathol Pharmacol 103:195–210
- Kim EH, Koh EH, Park JY, Lee KU. (2010). Adenine nucleotide translocator as a regulator of mitochondrial function: Implication in the pathogenesis of metabolic syndrome. Korean Diabetes J 34:146–53
- Kitagava A, Ohta Y, Ohashi K. (2012). Melatonin improves metabolic syndrome induced by high fructose intake in rats. J Pineal Res 52:403–13
- Kozirog M, Poliwczak AR, Duchnowicz P, et al. (2011). Melatonin treatment improves blood pressure, lipid profile, and parameters of oxidative stress in patients with metabolic syndrome. J Pineal Res 50:261–6
- Leibowitz A, Peleg E, Sharabi Y, et al. (2008). The role of melatonin in the pathogenesis of hypertension in rats with metabolic syndrome. Am J Hypertens 21:348–51
- Marchesi C, Ebrahimian T, Angulo O, et al. (2009). Endothelial nitric oxide synthase uncoupling and perivascular adipose oxidative stress and inflammation contribute to vascular dysfunction in a rodent model of metabolic syndrome. Hypertension 54:1384–92
- Miatello R, Vazguez M, Renna N, et al. (2005). Chronic administration of resveratrol prevent biochemical cardiovascular changes in fructose-fed rats. Am J Hypertens 18:864–70
- Miller A, Adeli K. (2008). Dietary fructose and the metabolic syndrome. Curr Opin Gastroenterol 24:204–9
- Muhlbauer E, Albrecht E, Bazwinsky-Wutschke I, Peschke E. (2012). Melatonin influences insulin secretion primarily via MT1 receptors in rat insulinoma cells (INS-1) and mouse pancreatic islets. J Pineal Res 52:446–59
- Murawska-Cialowicz E, Januszewska L, Zuwala-Jagiello J, et al. (2008). Melatonin decreases homocysteine level in blood of rats. J Physiol Pharmacol 59:717–29
- Nduhirabandi F, du Toit EF, Lochner A. (2012). Melatonin and the metabolic syndrome: A tool for effective therapy in obesity-associated abnormalities? Acta Physiol 205:209–23
- Okatani Y, Wakatsuki A, Reiter RJ. (2000). Protective effect of melatonin against homocysteine-induced vasoconstriction of human umbilical artery. Biochem Biophys Res Commun 277:470–5
- Okatani Y, Wakatsuki A, Reiter RJ. (2001). Melatonin suppresses homocysteine enhancement of serotonin-induced vasoconstriction in the human umbilical artery. J Pineal Res 31:242–7
- Reddy SS, Ramatholisamma P, Karuna R, Saralakumari D. (2009). Preventive effect of Tinospora cordifolia against high-fructose diet-induced insulin resistance and oxidative stress in male Wistar rats. Food Chem Toxicol 47:2224–9
- Rios-Lugo MJ, Cano P, Jimenez-Ortega V, et al. (2010). Melatonin effect on plasma adiponectin, leptin, insulin, glucose, triglycerides and cholesterol in normal and high fat-fed rats. J Pineal Res 49:342–8
- Robeva R, Kirilov G, Tomova A, Kumanov P. (2008). Melatonin-insulin interactions in patients with metabolic syndrome. J Pineal Res 44:52–6
- Sanchez-Lozada LG, Tapia E, Jimenez A, et al. (2007). Fructose-induced metabolic syndrome is associated with glomerular hypertension and renal microvascular damage in rats. Am J Physiol Renal Physiol 292:F423–9
- Sartori C, Dessen P, Mathieu C, et al. (2009). Melatonin improves glucose homeostasis and endothelial vascular function in high-fat diet-fed insulin-resistant mice. Endocrinology 150:5311–17
- Scherrer U, Randin D, Vollenweider P, et al. (1994). Nitric oxide release accounts for insulin's vascular effects in humans. J Clin Invest 94:2511–15
- Srinivasan V, Ohta Y, Espino J, et al. (2013). Metabolic syndrome, its pathophysiology and the role of melatonin. Recent Pat Endocr Metab Immun Drug Discov 7:11–25
- Stühlinger MC, Tsao PS, Her JH, et al. (2001). Homocysteine impairs the nitric oxide synthase pathway: Role of asymmetric dimethylarginine. Circulation 104:2569–75
- Sydow K, Münzel T. (2003). ADMA and oxidative stress. Atherosclerosis Suppl 4:41–51
- Tain YL, Hsieh CS, Chen CC, et al. (2010a). Melatonin prevents increased asymmetric dimethylarginine in young rats with bile duct ligation. J Pineal Res 48:212–21
- Tain YL, Huang LT, Lin IC, et al. (2010b). Melatonin prevents hypertension and increased asymmetric dimethylarginine in young spontaneous hypertensive rats. J Pineal Res 49:390–8
- Tain YL, Kao YH, Hsieh CS, et al. (2010c). Melatonin blocks oxidative stress-induced increased asymmetric dimethylarginine. Free Radic Biol Med 49:1088–98
- Tran LT, Yuen VG, McNeill JH. (2009). The fructose-fed rat: A review on the mechanisms of fructose-induced insulin resistance and hypertension. Mol Cell Biochem 332:145–59
- Verma S, Skarsgard P, Bhanot S. (1997). Reactivity of mesenteric arteries from fructose hypertensive rats to endothelin-1. Am J Hypertens 10:1010–19
- Wilcken DE, Wang J, Sim AS, et al. (2006). Asymmetric dimethylarginine in homocystinuria due to cystathionine beta-synthase deficiency: Relevance of renal function. J Inherit Metab Dis 29:30–7
- Zanuto R, Siqueira-Filho MA, Caperuto LC, et al. (2013). Melatonin improves insulin sensitivity independently of weight loss in old obese rats. J Pineal Res 55:156–65