Abstract
Context: Salvia miltiorrhiza Bge. (Labiatae) has been widely used for treating diabetes for centuries. Salvianolic acid B (SalB) is the main bioactive component in Salvia miltiorrhiza; however, its antidiabetic activity and possible mechanism are not yet clear.
Objective: To investigate the effects of SalB on glycometabolism, lipid metabolism, insulin resistance, oxidative stress, and glycogen synthesis in type 2 diabetic rat model.
Materials and methods: High-fat diet (HFD) and streptozotocin-induced diabetic rats were randomly divided into model group, SalB subgroups (50, 100, and 200 mg/kg), and rosiglitazone group.
Results: Compared with the model group, SalB (100 and 200 mg/kg) significantly decreased blood glucose (by 23.8 and 21.7%; p < 0.05 and p < 0.01) and insulin (by 31.3 and 26.6%; p < 0.05), and increased insulin sensitivity index (by 10.9 and 9.3%; p < 0.05). They also significantly decreased total cholesterol (by 24.9 and 27.9%; p < 0.01), low-density lipoprotein cholesterol (by 56.2 and 64.6%; p < 0.01), non-esterified fatty acids (by 32.1 and 37.9%; p < 0.01), hepatic glycogen (by 41.3 and 60.5%; p < 0.01), and muscle glycogen (by 33.2 and 38.6%; p < 0.05), and increased high-density lipoprotein cholesterol (by 50.0 and 61.4%; p < 0.05 and p < 0.01), which were originally altered by HFD and streptozotocin. In addition, SalB (200 mg/kg) markedly decreased triglyceride and malondialdehyde (by 31.5 and 29.0%; p < 0.05 and p < 0.01), and increased superoxide dismutase (by 56.6%; p < 0.01), which were originally altered by HFD and streptozotocin.
Discussion and conclusion: The results indicate that SalB can inhibit symptoms of diabetes mellitus in rats and these effects may partially be correlated with its insulin sensitivity, glycogen synthesis and antioxidant activities.
Introduction
Diabetes is currently one of the most prevalent and serious diseases worldwide. The global incidence of diabetes among adults is 6.4% (Shaw et al., Citation2010), while the incidence of total diabetes in China is 9.7% (Yang et al., Citation2010); more than 90% of diagnosed diabetics have been categorized as type 2 diabetics and present with hyperglycemia, dyslipidemia, and insulin resistance (Matthews et al., Citation1985). Many kinds of antidiabetic drugs have been used in the treatment of diabetes, such as dipeptidyl peptidase-IV inhibitors, sulfonylureas, biguanides, thiazolidinedione, α-glycosidase inhibitors, and insulin. However, these medications also have considerable side effects and high rates of secondary failure (Tahrani et al., Citation2010). Apart from these currently available therapeutic options, many herbal medicines have been recommended for the treatment of diabetes. A large number of ingredients present in medicinal plants are thought to act on a variety of targets by various modes and mechanisms, and they have the potential to impart therapeutic effects in complicated disorders like diabetes and its complications (Prabhakar & Doble, Citation2008). Therefore, searching natural plant-derived antidiabetic drugs possessing fewer side effects receives considerable attention.
Traditional Chinese medicines (TCMs), which have played a key role in maintaining the health of Chinese people for thousands of years, were found to be useful to successfully manage diabetes and diabetic complications (Li et al., Citation2004). Salvia miltiorrhiza Bge. (Labiatae) (known as Danshen in China), a well-known traditional Chinese herbal medicine, has been widely used in both Asian and Western countries for the treatment of cerebrovascular diseases, coronary artery diseases, diabetes, and diabetic complications (Lei & Chiou, Citation1986; Xie & Du, Citation2011; Zhou et al., Citation2005). As the most abundant and bioactive component, salvianolic acid B (SalB) has received a considerable attention and been assigned as the marker compound of Salvia miltiorrhiza in the Chinese Pharmacopoeia (Commission, Citation2010). Our previous report demonstrated that total polyphenolic acid fraction of Salvia miltiorrhiza, which containing 53.6% SalB, significantly decreased blood glucose, total cholesterol, and triglyceride levels, and increased insulin sensitivity in type 2 diabetic rats (Huang et al., Citation2012; Zhou et al., Citation2012). However, which compound from this extract is responsible for the antidiabetic effects is still unclear. In addition, a recent in vitro study (Cheng et al., Citation2013) has shown that SalB possessed the capacity to inhibit the formation of human islet armyloid polypeptide (hIAPP) amyloid and disaggregate hIAPP fibrils, and protected hIAPP-induced cytotoxicity on pancreatic INS-1 cells, which suggested a potential use of SalB for the treatment of diabetes. A systematic study, therefore, was required to verify the antidiabetic effects of SalB and to reveal its possible mechanism. In this study, the effects of SalB on blood glycemic control, lipid metabolism, insulin resistance, oxidative stress, and glycogen synthesis in the type 2 diabetic rat model were investigated and the known antidiabetic agent rosiglitazone was used as a positive control.
Materials and methods
Animals
Specific pathogen-free male Sprague–Dawley (SD) rats (160–200 g) were procured from the Center of Laboratory Animal Science of Guangdong Province (Foshan, China). All animals were examined for clinical signs of ill health upon receipt and observed within 5 d of arrival. The animals were housed as 2 in 1 medium poly-carbonated cage under standard environmental conditions (23 ± 2 °C, 40–60% relative humidity, and 12 h light/12 h dark cycle) and allowed free access to tap water and food. The rats were fed with a standard rodent pellet diet obtained from the Center of Laboratory Animal Science of Guangdong Province (Foshan, China). The experimental protocols for this study were approved by the Institutional Animal Care and Use Committee and the animals were kept according to the institutional ethical guideline of Fujian University of Traditional Chinese Medicine.
Drugs and reagents
SalB () with more than 98% purity was purchased from Shanghai Tauto Biotech Co., (Shanghai, China). Rosiglitazone was purchased from Hengrui Pharmaceutical Co., Ltd (Chengdu, China). STZ was purchased from Sigma (St. Louis, MO). Assay kits of total cholesterol (TC), triglyceride (TG), high-density lipoprotein cholesterol (HDL-C), low-density lipoprotein cholesterol (LDL-C), non-esterified fatty acids (NEFA), superoxide dismutase (SOD), malondialdehyde (MDA), creatinine (CREA), blood urea nitrogen (BUN), and glycogen were obtained from Nanjing Jiancheng Bio-Engineering Research Institute (Nanjing, China). Radioimmunoassay kit for insulin was obtained from Linco Research Co., Ltd (St. Louis, MO). All other chemicals were of the highest grade available.
Induction of type 2 diabetes in rats
The induction of diabetes in rats was done according to our previous study (Huang et al., Citation2012). Briefly, 10 healthy rats were continually feed with standard rodent pellet diet and 90 healthy rats were feed with high-fat diet (HFD) (consisting of 5% lard, 10% sucrose, 10% egg yolk, 0.2% cholesterol, 0.5% cholate, and 74.3% standard laboratory chow) after 1 week accommodation. After 1 month of dietary manipulation, the rats fed by HFD were fasted for 12 h (free access to water), and injected intraperitonially with 30 mg/kg STZ which was dissolved freshly in 0.1 M acetate buffer (pH 4.5). Ten control rats fed by standard rodent pellet diet were injected with same volume of buffer solution. 3 d after injection, all animals were fasted for 12 h, then blood samples were taken from their tail veins and fasting blood glucose (FBG) level was measured by using a one-touch glucose autoanalyzer (Roche, Mannheim, Germany). The animals with FBG between 11.1 and 33.3 mmol/L were considered diabetic (n = 53) and 50 within them were selected for next studies.
Drug administration
Diabetic rats were randomly divided into five groups of 10 rats in each as follows: model control group, low-, middle-, and high-dose SalB groups, and positive control group. These five groups were continued on their HFD for the duration of the study. The aforementioned 10 control rats without STZ induction were selected as normal control group. Low-, middle-, and high-dose SalB were administered with SalB 50, 100, and 200 mg/kg/d, respectively, the positive control group was treated with rosiglitazone 1.33 mg/kg/d, the normal control group, and the model control group were treated with distilled water. In our previous study (Huang et al., Citation2012), 187 mg/kg/d of total polyphenolic acids fraction (containing 53.6% SalB) isolated from Salvia miltiorrhiza was found to be useful at improving blood glycometabolism, lipid metabolism, and insulin resistance in diabetic rats. Based on the content of SalB (53.6%) in this extract, the actual dose of SalB was calculated to be 100 mg/kg/d. Therefore, 100 mg/kg/d SalB was selected as the middle level, and 50 and 200 mg/kg/d were chosen as the low and highest levels in this experiment. In addition, the dose of rosiglitazone was set at 1.33 mg/kg/d according to its clinical dose. The gavage volume was 1 mL/100 g body weight, and the administration continued for 6 weeks. The necessary amount of drugs adjusted according to the weekly weight of the rats.
Biochemical analysis
After fasting for 12 h (free access to water), blood samples were taken from the tail vein at the 2th, 4th, and 6th week, and blood glucose concentrations was measured using one-touch glucose autoanalyzer. At the end of the experimental period, all rats were anesthetized and sacrificed by cervical dislocation after fasting for 12 h (free access to water). Whole blood was collected and serum was isolated for biochemical analysis. Serum TC, TG, HDL-C, LDL-C, CREA, and BUN were measured with a fully automated biochemical analyzer (ECHO, Rome, Italy). Serum NEFA, SOD, and MDA were determined using a UV–Vis 752 spectrophotometer (Lengguang Optical Instrument, Shanghai, China). Serum insulin was assessed by the SN-6100 γ-radiation-immunity counter (Beijing Chemclin Biotech, Beijing, China), and the insulin sensitivity index (ISI) was calculated according to the following formula: ISI = ln [1/(FBG × FINS)] (Li et al., Citation1993). All the measurements were carried out in accordance with the instructions from manufactures.
Measurement of glycogen in liver and muscle
At the end of the experiment, after sacrificing the rats, the right lateral lobe of liver and the right soleus muscle were quickly excised, washed with ice-cold saline, and stored at −80°C for glycogen assay. On the day of analysis, the frozen sample was thawed at room temperature and used for glycogen extraction. Each frozen tissue (about 50 mg) was extracted by KOH solution, precipitated with ethanol and redissolved in distilled water. Thereafter, the contents of hepatic glycogen and muscle glycogen (expressed as mg/g wet tissue) were determined with a spectrophotometer at 620 nm with a commercial kit (Keller et al., Citation2011).
Statistical analysis
The Statistical Package for Social Science (SPSS Inc., Chicago, IL), version 12.0, was used for statistical analysis. The values were presented as means ± standard error (SEM). Before statistical analysis, all variables were checked for homogeneity and normality of variance by using Bartlett’s test and the Kolmogorov–Smirnoff test, respectively. The data obtained were tested by ANOVA followed by Tukey’s post hoc multiple comparison test. A value of p < 0.05 was considered as statistically significant.
Results
Effect of SalB on body weight
As shown in , there was no significant variation on the initial body weight of each group. However, the body weight of normal rats gradually increased in this experiment period, while it decreased remarkably in diabetic rats from weeks 2 to 6 (p < 0.01). Moreover, low-, middle-, and high-dose SalB groups and the positive control group had slightly higher weight gain than the model control group from weeks 2 to 6, but these differences were not statistically significant (p > 0.05). And there was no significant difference between these groups (p > 0.05).
Figure 2. Effects of SalB on body weight in diabetic rats. The values are expressed as means ± SEM (n = 10). *p < 0.01 as compared with the NC group. NC, normal control; MC, model control; ROS, diabetic rats treated with rosiglitazonen (1.33 mg/kg, bw); HSB, diabetic rats treated with high dose of SalB (200 mg/kg, bw); MSB, diabetic rats treated with middle dose of SalB (100 mg/kg, bw); LSB, diabetic rats treated with low dose of SalB (50 mg/kg, bw).
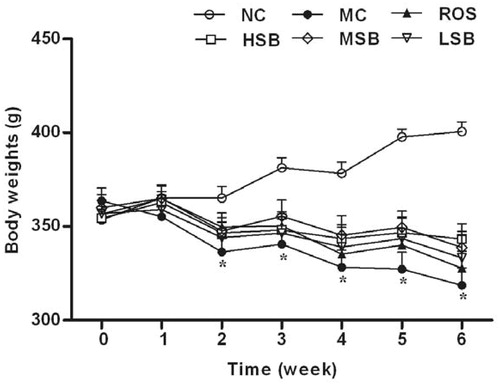
Effect of SalB on FBG, fasting blood insulin, and ISI
The changes in FBG, fasting blood insulin, and ISI are presented in . The FBG concentrations in diabetic rats were significantly higher than normal rats after the induction of STZ, and at the following 6-week period of drug administration, the FBG concentrations in the model control group increased with time (20.7–24.4 mmol/L). At 4 and 6 weeks after drug administration, the FBG concentrations in middle- and high-dose SalB groups and the positive control group were significantly lower than the model control group (by 19.5 and 21.7%, 21.0 and 23.8%, and 18.8 and 17.2%, respectively) (), but there was no significant difference between these groups (p > 0.05). Besides, diabetic rats showed higher fasting blood insulin and lower ISI levels than normal rats at the end of this experiment; however, after 6 weeks of treatment, middle- and high-doses of SalB and rosiglitazone significantly reduced blood insulin (by 26.6, 31.3, and 45.1%, respectively) and markedly increased ISI (by 9.3, 10.9, and 13.7%, respectively) (), and there was no significant difference between these groups (p > 0.05). In sum, there is a dose-dependent response of SalB, and those effects of middle- and high-dose SalB were similar to that of rosiglitazone.
Figure 3. Effects of SalB on serum FBG, insulin, and ISI in diabetic rats for 6 weeks. The values are expressed as means ± SEM (n = 10). *p < 0.01 as compared with the NC group. #p < 0.05 as compared with the MC group, ##p < 0.01 as compared with the MC group. NC, normal control; MC, model control; ROS, diabetic rats treated with rosiglitazonen (1.33 mg/kg, bw); HSB, diabetic rats treated with high dose of SalB (200 mg/kg, bw); MSB, diabetic rats treated with middle dose of SalB (100 mg/kg, bw); LSB, diabetic rats treated with low dose of SalB (50 mg/kg, bw).
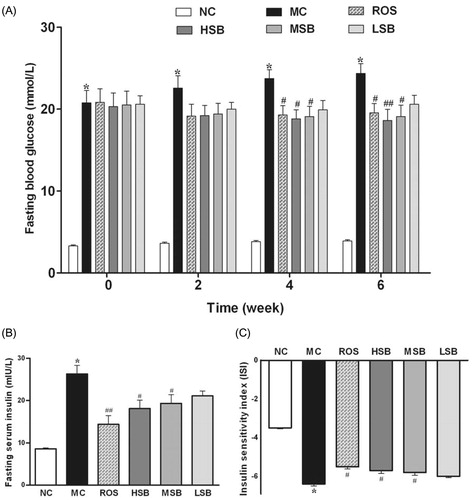
Effect of SalB on serum lipid profile, CREA, and BUN
depicts the values of fasting serum TC, TG, LDL-C, HDL-C, NEFA, CREA, and BUN in control and experimental rats. There were significant elevations in the levels of TC, TG, LDL-C, and NEFA with significant decrease in the level of HDL-C in diabetic rats compared with normal rats. After 6 weeks of drug treatment, the TC, LDL-C, and NEFA levels in all SalB treated groups were significantly lower than the model control group (p < 0.01). In addition, compared with the model control group, the HDL-C level in middle- and high-dose SalB groups were significantly increased (p < 0.05 and p < 0.01), and the TG level in high-dose SalB group was significantly decreased (p < 0.05). Administration of rosiglitazone was as effective as high-dose SalB without affecting TG and HDL-C significantly (p > 0.05). In addition, there was no obvious difference in CREA and BUN levels between all groups (p > 0.05).
Figure 4. Effects of SalB on TC, TG, LDL-C, HDL-C, and NEFA in diabetic rats for 6 weeks. The values are expressed as means ± SEM (n = 10). *p < 0.01 as compared with the NC group. #p < 0.05 as compared with the MC group, ##p < 0.01 as compared with the MC group. △p < 0.05 as compared with the ROS group. NC, normal control; MC, model control; ROS, diabetic rats treated with rosiglitazonen (1.33 mg/kg, bw); HSB, diabetic rats treated with high dose of SalB (200 mg/kg, bw); MSB, diabetic rats treated with middle dose of SalB (100 mg/kg, bw); LSB, diabetic rats treated with low dose of SalB (50 mg/kg, bw).
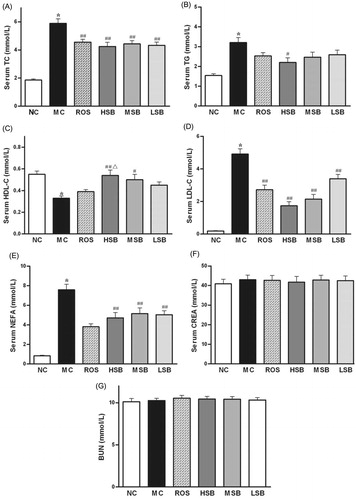
Effect of SalB on serum SOD activity and MDA level
As shown in , serum SOD activity was significantly inhibited and MDA level was significantly elevated in diabetic rats compared with normal rats. Administration of SalB (50 and 200 mg/kg) to diabetic rats resulted in significant increase of SOD activity (by 22.7 and 29.0%) and reduction of MDA level (by 45.7 and 56.6%) in serum when compared with untreated diabetic rats. However, no significant change was found in the values of SOD and MDA when administered with middle-dose SalB or rosiglitazone (p > 0.05). And there was no significant difference between all treated groups (p > 0.05).
Figure 5. Effects of SalB on serum SOD activity and MDA level in diabetic rats for 6 weeks. The values are expressed as means ± SEM (n = 10). *p < 0.01 as compared with the NC group. #p < 0.05 as compared with the MC group, ##p < 0.01 as compared with the MC group. NC, normal control; MC, model control; ROS, diabetic rats treated with rosiglitazonen (1.33 mg/kg, bw); HSB, diabetic rats treated with high dose of SalB (200 mg/kg, bw); MSB, diabetic rats treated with middle dose of SalB (100 mg/kg, bw); LSB, diabetic rats treated with low dose of SalB (50 mg/kg, bw).
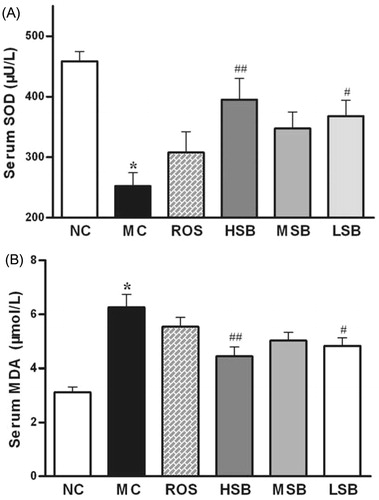
Effect of SalB on liver glycogen and muscle glycogen
The data for liver and muscle glycogen changes are shown in . Compared with normal rats, a significant decline in the liver glycogen level as well as in the muscle glycogen level was observed in diabetic rats. Treatment with SalB (100 and 200 mg/kg) and rosiglitazone increased the levels of liver glycogen (by 41.3, 60.5, and 55.5%) and muscle glycogen (by 33.2, 38.6, and 41.7%) when compared with untreated diabetic rats. In summary, there is a dose-dependent response of SalB, and those effects of middle- and high-dose SalB were similar to that of rosiglitazone.
Figure 6. Liver and muscle glycogen contents in diabetic rats treated with SalB for 6 weeks. The values are expressed as means ± SEM (n = 10). *p < 0.01 as compared with the NC group. #p < 0.05 as compared with the MC group, ##p < 0.01 as compared with the MC group. NC, normal control; MC, model control; ROS, diabetic rats treated with rosiglitazonen (1.33 mg/kg, bw); HSB, diabetic rats treated with high dose of SalB (200 mg/kg, bw); MSB, diabetic rats treated with middle dose of SalB (100 mg/kg, bw); LSB, diabetic rats treated with low dose of SalB (50 mg/kg, bw).
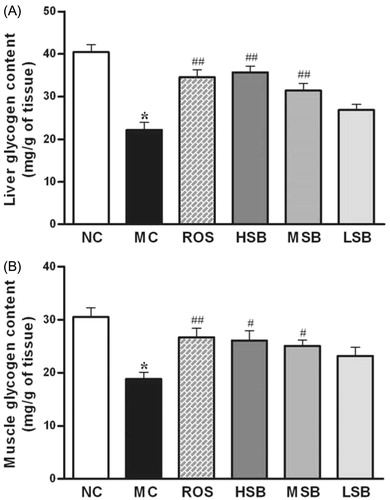
Discussion and conclusion
High-fat diet feeding and low-dose STZ-induced diabetes in rodents are considered to mimic closely the natural history of human type 2 diabetes and are widely used to valuate anti-diabetic compounds and extracts (Islam & Choi, Citation2008; Srinivasan et al., Citation2005). This animal model exhibits typical symptoms of human type 2 diabetes, such as hyperglycemia, hyperlipidemia, and hyperinsulinemia. Results of our study have been consistent with these observations that the model showed significant increases in FBG (by 531.0%), TC (by 216.5%), TG (by 108.9%), and insulin (by 206.8%) as well as ISI (an index of insulin sensitivity) decline (by 84.0%) when compared with normal rats. However, the above disturbances were inhibited or brought back to near normal mediated by SalB treatment and those results were similar to that of rosiglitazone, a well-known hypoglycemic drug.
Hyperglycemia is a macroscopic manifestation of diabetes symptom, and persistence hyperglycemia will affect cells, tissues, and organs all over the body, because it is the main factor to cause diabetes chronic complications. Achieving and maintaining near-normoglycemia are imperative to prevent or delay the onset and progression of long-term complications of the disease (Li et al., Citation2012). In the present study, the FBG level of diabetic rats was significantly decreased at 4th and 6th week after SalB treatment (100 and 200 mg/kg), providing direct evidence of the antihyperglycemic effect of SalB. Moreover, the insulin level was decreased and ISI level was increased in diabetic rats after SalB treatment similar to positive drug (rosiglitazone), which has been proved to exert its antidiabetic effects through improving insulin sensitivity (Ezhumalai et al., Citation2014). Therefore, from these results of the present study, it seems likely that an increase in sensitivity to insulin contributes to the antidiabetic effects of SalB.
It has long been known that in addition to hyperglycemia, the type 2 diabetic individual almost invariably manifests a serious breakdown in lipid dynamics, often reflected by elevated levels of TG, TC, LDL-C, and NEFA, which are often associated with an increased risk of type 2 diabetes and diabetes-associated microvascular and macrovascular diseases (McLachlan & Alford, Citation2005; Rutledge et al., Citation2010). In our study, treatment with SalB (100 and 200 mg/kg) for 6 weeks dramatically improved lipid metabolites including serum TC, TG, LDL-C, HDL-C, and NEFA levels in diabetic rats as reported earlier (Lv et al., Citation2006; Zheng et al., Citation2011), indicating that this compound might regulate the lipids metabolism, which will be beneficial to preventing the development of type 2 diabetes and reducing the risk of microvascular or macrovascular diseases.
Liver plays a vital role in maintaining blood glucose homeostasis by glycogenesis and glycogen breakdown or gluconeogenic precursors, and its normal capacity to synthesize glycogen appear to be defective in patients with type 2 diabetes (Roden & Bernroider, Citation2003). In addition to liver, muscle is another important metabolic organ for glucose metabolism (Schalin-Jantti et al., Citation1992). Decrease in both hepatic and muscle glycogen of diabetic rats was observed in this study. Treatment with SalB (100 and 200 mg/kg) obviously increased liver and muscle glycogen indicating that the impaired glycogenesis and/or glycogen storage of the diabetic state was partially restored by this compound.
Decrease in SOD activity and increase in MDA level may also accelerate the pathogenesis progress of diabetes and its complications (Chan et al., Citation2009). The hypoglycemic mechanisms of many polyphenolic compounds in medical plants such as green tea catechins, pomegranate polyphenols, and coffee polyphenols are closely related to their antioxidant activities (Basu et al., Citation2013; Crespy & Williamson, Citation2004; Mubarak et al., Citation2013). Some previous studies have shown that SalB has a strong antioxidant property and can scavenge reactive oxygen species and improve the activity of antioxidant enzymes both in vitro and in vivo (Chen et al., Citation2000; Gao et al., Citation2012; Liu et al., Citation2007). In the current study, diabetic rats treated with SalB (50 and 200 mg/kg) showed notably increased serum SOD (a scavenger of free radicals) ability and decreased MDA (a by-product of lipid peroxidation) level, indicating its potential antioxidative ability in vivo. Therefore, the antioxidant activity of SalB might have played an important role in the development of diabetes.
In conclusion, the present study clearly demonstrated that SalB has a certain beneficial effect on ameliorating hyperglycemia, hyperlipidemia, and insulin resistance in diabetic rats, which may partly relate to several mechanisms such as insulin sensitizing, glycogen synthesizing, and antioxidant activities. Although the precise mechanisms for the observed effects of SalB remains to be determined, its effective antidiabetic activity with multiple therapeutic effects suggests that SalB is a potential component for further drug development on diabetes and its complications.
Declaration of interest
The authors have declared that there is no conflict of interest. This work was supported by the National Natural Science Foundation of China (81373940), the Specialized Research Fund for the Doctoral Program of Higher Education (20133519120001), and the Provincial Natural Science Foundation of Fujian (2012J05152 and 2012Y4005). The authors are grateful to Collaborative Innovation Center for Rehabilitation Technology and TCM Rehabilitation Research Center of SATCM for providing part financial support.
References
- Basu A, Newman ED, Bryant AL, et al. (2013). Pomegranate polyphenols lower lipid peroxidation in adults with type 2 diabetes but have no effects in healthy volunteers: A pilot study. J Nutr Metab 2013:Article ID 708381, 7 pages
- Chan JY, Lam FC, Leung PC, et al. (2009). Antihyperglycemic and antioxidative effects of a herbal formulation of Radix astragali, Radix codonopsis and Cortex lycii in a mouse model of type 2 diabetes mellitus. Phytother Res 23:658–65
- Chen YH, Du GH, Zhang JT. (2000). Salvianolic acid B protects brain against injuries caused by ischemia-reperfusion in rats. Acta Pharmacol Sin 21:463–6
- Cheng B, Gong H, Li X, et al. (2013). Salvianolic acid B inhibits the amyloid formation of human islet amyloid polypeptideand protects pancreatic beta-cells against cytotoxicity. Proteins 81:613–21
- Commission CP. (2010). Pharmacopoeia of the People's Republic of China. Beijing, China: Chinese Medical Science and Technology Press
- Crespy V, Williamson G. (2004). A review of the health effects of green tea catechins in in vivo animal models. J Nutr 134:3431S–40
- Ezhumalai M, Radhiga T, Pugalendi KV. (2014). Antihyperglycemic effect of carvacrol in combination with rosiglitazone in high-fat diet-induced type 2 diabetic C57BL/6J mice. Mol Cell Biochem 385:23–31
- Gao HY, Li GY, Lou MM, et al. (2012). Hepatoprotective effect of matrine salvianolic acid B salt on carbon tetrachloride-induced hepatic fibrosis. J Inflamm (Lond) 9:16–21
- Huang MQ, Xie YL, Chen LD, et al. (2012). Antidiabetic effect of the total polyphenolic acids fraction from Salvia miltiorrhiza Bunge in diabetic rats. Phytother Res 26:944–8
- Islam MS, Choi H. (2008). Dietary red chilli (Capsicum frutescens L.) is insulinotropic rather than hypoglycemic in type 2 diabetes model of rats. Phytother Res 22:1025–9
- Keller AC, Ma J, Kavalier A, et al. (2011). Saponins from the traditional medicinal plant Momordica charantia stimulate insulin secretion in vitro. Phytomedicine 19:32–7
- Lei XL, Chiou GC. (1986). Studies on cardiovascular actions of Salvia miltiorrhiza. Am J Chin Med 14:26–32
- Li GW, Fan XR, Lillioja S, Bennett PH. (1993). A new index to evaluate insulin sensitivity. Chin J Intern Med 32:656–60
- Li WL, Zheng HC, Bukuru J, De KN. (2004). Natural medicines used in the traditional Chinese medical system for therapy of diabetes mellitus. J Ethnopharmacol 92:1–21
- Li Y, Chen M, Xuan H, Hu F. (2012). Effects of encapsulated propolis on blood glycemic control, lipid metabolism, and insulin resistance in type 2 diabetes mellitus rats. Evid Based Complement Alternat Med 2012:Article ID 981896, 8 pages
- Liu CS, Chen NH, Zhang JT. (2007). Protection of PC12 cells from hydrogen peroxide-induced cytotoxicity by salvianolic acid B, a new compound isolated from Radix salviae miltiorrhizae. Phytomedicine 14:492–7
- Lv BQ, Fan YC, Sun LS. (2006). The influence of salvianolic acid B and tanshinone IIA on the nitric oxide and triglyceride in sera of atherosclerosis rabbits. J Tianjin Univ Tradit Chin Med 25:32–4
- Matthews DR, Hosker JP, Rudenski AS, et al. (1985). Homeostasis model assessment: Insulin resistance and beta-cell function from fasting plasma glucose and insulin concentrations in man. Diabetologia 28:412–19
- McLachlan KA, Alford FP. (2005). The impact of acute elevation of non-esterified fatty acids on insulin sensitivity and secretion in women with former gestational diabetes. Clin Endocrinol (Oxf) 62:79–84
- Mubarak A, Hodgson JM, Considine MJ, et al. (2013). Supplementation of a high-fat diet with chlorogenic acid is associated with insulin resistance and hepatic lipid accumulation in mice. J Agric Food Chem 61:4371–8
- Prabhakar PK, Doble M. (2008). A target based therapeutic approach towards diabetes mellitus using medicinal plants. Curr Diabetes Rev 4:291–308
- Roden M, Bernroider E. (2003). Hepatic glucose metabolism in humans – Its role in health and disease. Best Pract Res Clin Endocrinol Metab 17:365–83
- Rutledge JC, Ng KF, Aung HH, Wilson DW. (2010). Role of triglyceride-rich lipoproteins in diabetic nephropathy. Nat Rev Nephrol 6:361–70
- Schalin-Jantti C, Harkonen M, Groop LC. (1992). Impaired activation of glycogen synthase in people at increased risk for developing NIDDM. Diabetes 41:598–604
- Shaw JE, Sicree RA, Zimmet PZ. (2010). Global estimates of the prevalence of diabetes for 2010 and 2030. Diabetes Res Clin Pract 87:4–14
- Srinivasan K, Viswanad B, Asrat L, et al. (2005). Combination of high-fat diet-fed and low-dose streptozotocin-treated rat: A model for type 2 diabetes and pharmacological screening. Pharmacol Res 52:313–20
- Tahrani AA, Piya MK, Kennedy A, Barnett AH. (2010). Glycaemic control in type 2 diabetes: Targets and new therapies. Pharmacol Ther 125:328–61
- Xie W, Du L. (2011). Diabetes is an inflammatory disease: Evidence from traditional Chinese medicines. Diabetes Obes Metab 13:289–301
- Yang W, Lu J, Weng J, et al. (2010). Prevalence of diabetes among men and women in China. N Engl J Med 362:1090–101
- Zheng F, Guo MJ, Gu LY, Fan YC. (2011). Effects of salvianolic acid B on stability of atherosclerotic plaque in apolipoprotein E gene knock-out mice treated with STZ and high fat diet. Chin J Arterioscler 11:89–93
- Zhou CJ, Huang MQ, Chen CQ, et al. (2012). Salvianolic acids improves glucose and lipid metabolism and insulin resistance in rat models with type 2 diabetes. Chin J Exp Tradit Med Formulae 18:233–7
- Zhou L, Zuo Z, Chow MS. (2005). Danshen: An overview of its chemistry, pharmacology, pharmacokinetics, and clinical use. J Clin Pharmacol 45:1345–59