Abstract
Context: Wild carrot, Daucus carota L. ssp. carota (Apiacae), is widely distributed throughout the world and has various uses in traditional medicine in Lebanon.
Objective: The present study aimed to fractionate and analyze the chemical composition of the Daucus carota oil extract (DCOE) fractions and to evaluate their antioxidant and hepatoprotective properties in vitro and in vivo.
Materials and methods: DCOE was chromatographed on silica gel column to produce four fractions: pentane (F1), 50:50 pentane:diethyl ether (F2), diethyl ether (F3), and 93:7 chloroform: methanol (F4). Qualitative and quantitative analyses of oil fractions were performed by GC-MS and HPLC techniques. The in vitro antioxidant properties were assessed using DPPH, FIC, and ferric-reducing antioxidant power (FRAP) assays. The hepatoprotective property was determined by examining the levels of serum markers (alanine transaminase (ALT) and aspartate transaminase (AST)) and hepatic antioxidant (superoxide dismutase (SOD), catalase (CAT), and glutathione-S-transferase (GST)) enzymes in CCl4-intoxicated mice pretreated with intraperitoenal 50, 100, or 200 mg/kg b.w. of the oil fractions for 5 d.
Results: GCMS analysis of F2 revealed the presence of 2-himachalen-6-ol (61.4%) which is reported for the first time in Daucus carota species. F3 and F4 were rich in phenolics and flavonoids and demonstrated significant DPPH activity (IC50 = 0.29 and 0.38 mg/ml, respectively) and high FRAP values (225.11 and 437.59 µmol FeSO4/g, respectively). The sesquiterpene-rich fraction F1 had the highest FIC ability (IC50 = 0.28 mg/ml). Pretreatment with F1 and F4 reversed the CCl4-induced decrease in SOD, CAT, and GST levels and reduced significantly hepatic damage.
Discussion and conclusion: The current results suggested that wild carrot oil fractions exhibited a unique chemical composition and possessed significant antioxidant activities as well as hepatoprotective effects against CCl4-induced hepatotoxicity.
Introduction
There is growing evidence that many human diseases are caused by oxidative stress, which partially results from an imbalance between the formation and the neutralization of pro-oxidants (Hazra et al., Citation2008). Oxidative stress is induced by free radicals, mainly reactive oxygen species (ROS), which include the superoxide radical (), hydrogen peroxide (H2O2), and hydroxyl radical (
). ROS molecules are highly reactive against macromolecules such as DNA, proteins, and membrane lipids in living cells. These changes are associated with the development of aging, cancer, cardiovascular, and inflammatory diseases (Braca et al., Citation2002; Hemnani & Parihar, Citation1998). The human body has natural defense mechanisms that protect against free radicals and oxidative damage. These mechanisms are both enzymatic, such as the ones catalyzed by superoxide dismutase (SOD), catalase (CAT), and the glutathione system (glutathione, glutathione reductase, peroxidase, and transferase), and non-enzymatic, such as the ones led by low molecular weight antioxidants such as vitamins A, E, and C (Evans et al., Citation2004; Manda et al., Citation2009). Although ROS exist in all aerobic cells, they are normally in equilibrium with antioxidants to maintain lower free radicals levels (Waris & Ahsan, Citation2006). However, if the balance between free radicals and the antioxidant defense system is compromised, the need for antioxidant supplements becomes necessary to combat oxidative damage (Shukla et al., Citation2009). Over the last few decades, the literature has revealed the importance of natural products as a source of potent antioxidants (Mantle et al., Citation2000). Among the most described are phenolic acids, polyphenols, monoterpenes, sesquiterpenes, and phenylpropanoids which have been found to possess antioxidant activity (Medina-Holguín et al., Citation2008; Sibanda et al., Citation2004). These compounds have the capacity to scavenge free radicals and to increase the levels of antioxidant enzymes thus protecting the body against damage from ROS (Tachakittirungrod et al., Citation2007; Terao, Citation2009).
Wild carrot, Daucus carota L. ssp. carota (Apiacae), is a spiny-fruited herb that grows in moderate regions of Europe, Asia, Africa, and North and South America (Mitich, Citation1996). The plant is commonly consumed as a salad in the Mediterranean diet or used as an additive in some recipes (Nehir El & Karakaya, Citation2004). Its flower umbels are edible and usually French fried, and its seed oil, which is commercially available, is used to flavor beverages and food products (Leung, Citation1980). In Lebanon, people eat its young taproot cooked and use the plant in folk medicine for the treatment of cancer, gastric ulcer, diabetes, muscle pain, and to protect against hepatic diseases. Available literature on the therapeutic effects of wild carrot showed that the plant possesses antilithic, diuretic (Thomas et al., Citation2001; Wyk & Wink, Citation2004) antibacterial, and antifungal activities (Maxia et al., Citation2009; Rossi et al., Citation2007; Staniszewska et al., Citation2005). The oil extract of wild carrot has been shown to consist mainly of phenols, flavonoids, monoterpenes, sesquiterpenes, and phenylpropanoids (Gonny et al., Citation2004; Maxia et al., Citation2009; Mockute & Nivinskiene, Citation2004; Shebaby et al., Citation2013; Staniszewska et al., Citation2005). However, the percentage composition of the oil was shown to vary greatly with different geographical origins (Shebaby et al., Citation2013). Lately, we reported that the aqueous and methanolic extracts of wild carrot umbels exhibited anti-inflammatory, anti-ulcer (Wehbe et al., Citation2009), anticancer (Shebaby et al., Citation2013; Zeinab et al., Citation2011), and antioxidant activities (Wehbe et al., Citation2009). In the current study, we aimed at analyzing the chemical composition of the Daucus carota oil extract (DCOE) fractions, evaluating their in vitro and in vivo antioxidant activities as well as hepatoprotective properties.
Materials and methods
Chemicals
Folin–Ciocalteu reagent, 1,1-diphenyl-2-picryl hydrazyl (DPPH), 2,4,6-tri(2-pyridyl)-1,3,5-triazine (TPTZ), quercetin, vanillic acid, chlorogenic acid, syringic acid, caffeic acid, ellagic acid, myricetin, luteolin, kaempferol, apigenin, ferrozine, gallic acid, trolox, pyrogallol, 1-chloro-2,4-dinitrobenzene (CDNB), dimethylsulfoxide (DMSO), and silica gel 40 (35–70) mesh were purchased from Sigma Aldrich, St. Louis, MO. All other chemicals used in the experiments were of analytical grade.
Sample collection and oil extraction
Daucus carota ssp. carota mature umbels were collected at the post-flowering season between August and September (2012) from Amioun, North Lebanon. The plant was identified according to the characteristics described in “Handbook of Medicinal Herbs” (Wyk & Wink, Citation2004) and confirmed by Dr. A. Houri, a Lebanese plant expert, Departmental of Natural Sciences at the Lebanese American University. A voucher specimen of the plant was deposited in the Departmental herbarium for further records. The extraction procedure was carried out according to the method described by Zeinab et al. (Citation2011). Briefly, 1 kg of air-dried umbels were cut into small pieces and soaked in 5 l of methanol/acetone (1:1) for 72 h. The extraction was repeated twice and the extracts were collected, filtered, and evaporated to dryness under reduced pressure. The residue was centrifuged at 500 rpm and the oil was removed and dried over anhydrous sodium sulfate yielding 34.7 g of crude oil extract, which was stored in a closed amber bottle at 4 °C until use.
Oil fractionation
DCOE (30 g) was chromatographed on a silica gel column (35–70 mesh). The first fraction (F1) was eluted with pentane (100%), the second fraction (F2) with pentane:diethyl ether (50:50), the third fraction (F3) with diethyl ether (100%), and the fourth fraction (F4) with chloroform:methanol (93:7). Fractions were analyzed by TLC using hexane:ethyl acetate (70:30) as a mobile phase and plates were stained with 2% anisaldehyde.
Gas chromatography and mass spectrometry analysis
The composition of the oil fractions was analyzed using gas chromatography and mass spectrometry (GC–MS) (Hewlett Packard, HP6890 series, Hewlett-Packard, Palo Alto, CA) fitted with a fused silica HP5-MS 5% phenyl methyl siloxane cap column (30 m × 0.25 mm i.d., film thickness 0.25) and directly coupled to the MS. The carrier gas was helium with splitless injection and the flow rate of 1.2 ml/min was applied. The temperature program was as follows: 2.0 min at 70 °C, from 70 °C to 130 °C at 8 °C/min and hold for 5 min, from 130 °C to 180 °C at 2 °C/min and hold for 10 min, from 180 °C to 220 °C at 15 °C/min and hold for 2 min and then from 220 °C to 280 °C at 15 °C/min and hold for 22 min. Identification of the components was performed by comparing their mass spectra with the literature (NIST11 and W9). Percentage composition was computed from GC peak areas.
HPLC analysis of oil fractions
The analysis of phenolic acids and flavonoids was performed on a Shimadzo HPLC system (Shimadzu Corp., Kyoto, Japan) consisting of LC 10-ADVP pump, SCL 10 A system controller coupled with a photo-diode array detector (SPD-M20A), FCV-10AL low-pressure gradient, rheodyne injector (Model 7125), DGU-20 A online degasser, Shim-pack VP-ODS column, 4.6 mm i.d. × 150 mm), pre-column (10 × 4.6 mm i.d. 5 µm) equipped with LC solution 1.23 SP1 software (Shimadzu, Kyoto, Japan). The column was operated at 25 °C. The mobile phase consisted of 10:2:88 – water:acetic acid:methanol v/v (solvent A) and 90:2:8 – water:acetic acid:methanol v/v (solvent B) at a flow rate of 1.5 ml/min. The gradient elution program was as follows: 0–15 min solvent A, 15–30 min solvent followed by washout period for 10 min, and the wavelength of detection was set at 280 nm. The phenolic acids and flavonoids were identified by matching the retention time and their spectral characteristics with those of the standard compounds (gallic acid, chlorogenic acid, vanillic acid, syringic acid, caffeic acid, ellagic acid, myricetin, quercetin, luteolin, kaempferol, and apigenin).
Total flavonoid content determination
Total flavonoid content of DCOE fractions was determined by the aluminum chloride colorimetric method (Chang et al., Citation2002). Briefly, 0.5 ml of each fraction (ranging from 0.2 to 5 mg/ml) in methanol was separately mixed with 1.5 ml of methanol, 0.1 ml of 10% aluminum chloride, 0.1 ml of 1 M potassium acetate, and 2.8 ml of distilled water. The absorbance of the reaction mixture was measured at 415 nm. Different concentrations of quercetin (12.5–200 µg/ml) in methanol were used as a standard curve.
Total phenolic content determination
Total phenolic content of DCOE fractions was determined according to the method described by Rossi et al. (Citation2007) using gallic acid as a standard. About 0.3 ml of each fraction in methanol (0.5–5 mg) was mixed with the Folin–Ciocalteu reagent (2.5 ml, 1:10 dilution) for 5 min. Then 2 ml of aqueous Na2CO3 (7.5% w/v) was added and the mixture allowed to stand for 30 min at 25 °C. Absorption at 760 nm was measured spectrophotometerically. The results were expressed as mg gallic acid equivalent (GAE) per gram of oil fraction.
2,2-Diphenyl-1-picrilhydrazyl (DPPH) radical scavenging assay
The DPPH assay was determined according to the method described by Mossa and Nawwar (Citation2011). Samples (200 μl) of oil fractions of (0.025–1 mg/ml in DMSO) were mixed with 1.5 ml of 0.1 mM DPPH in methanol, and the final volume was adjusted to 3.5 ml with DMSO. The mixture was then shaken vigorously and allowed to stand for 30 min in the dark at room temperature. The absorbance was determined at 517 nm. Trolox was used as a reference compound. Absorbance of DPPH in DMSO was used as a control. The percent inhibition of DPPH free radical by the samples was calculated according to the following formula:
where Ac and As are the absorbance of the control and the sample, respectively. The IC50 value represented the concentration of the oil extract that caused 50% inhibition.
Ferrous ion-chelating ability assay
The ferrous ion-chelating (FIC) assay was carried out according to the method described by Dinis et al. (Citation1994) with slight modifications. Aliquots of 1 ml of different concentrations of the oil fractions (0.25–4 mg/ml) were mixed with 1 ml FeCl2·4H2O (0.1 mM) and incubated for 5 min. The reaction was initiated by the addition of 1 ml ferrozine (0.25 mM) and the absorbance of the solution was measured at 562 nm. The percentage of the chelating ability of each fraction was calculated using the following formula:
where A0 is the absorbance of control sample (the control contains FeCl2 and ferrozine) and As is the absorbance of a tested sample. Na2EDTA was used as a positive control.
Ferric reducing antioxidant power assay
The total antioxidant power of the oil fractions was determined according to the method described by Benzie and Strain (Citation1996). Briefly, the ferric reducing antioxidant power (FRAP) reagent was prepared by mixing 300 mM of acetate buffer (pH 3.6), 10 mM TPTZ in 40 mM HCl and 10 mM FeCl3 at a ratio of 10:1:1. Aliquots (200 μl) of oil fractions (0.25–4 mg/ml) in methanol were added to 2.8 ml of the FRAP solution and the absorbance was recorded at 593 nm. Vitamin C was used as a positive control. The antioxidant power of the sample was calculated from the calibration curve of FeSO4 solution and expressed as mM FeSO4 equivalent per gram of sample.
CCl4-induced hepatotoxicity model
BALB/c mice, weighing 27–30 g (Lebanese American University stock) were housed under stable conditions of temperature (20 ± 2 °C) and humidity (50 ± 5%), and an alternating cycle of light and dark. The animals were supplied with standard laboratory chow diet and water. All experimental protocols were approved by the Animal Ethical Committee of the Lebanese American University, which complies with the Guide for the Care and Use of Laboratory Animals (Committee for the Update of the Guide for the Care and Use of Laboratory Animals, 2010).
Liver damage was induced by intraperitoneal injection of CCl4 in olive oil (1:1, v/v) at a dose of 2 ml/kg body weight (Coballase-Urrutia et al., Citation2011). The mice were divided randomly into 15 groups of six animals each. Group I served as a normal control and was given DMSO (2 ml/kg) for 5 d and olive oil on days 2 and 3. Group II served as a CCl4 control and received DMSO (2 ml/kg) for 5 d and CCl4/olive oil on days 2 and 3. Group III, positive control, was given vitamin E (100 mg/kg body weight) in DMSO (2 ml/kg) for 5 d and CCl4/olive oil on days 2 and 3. Groups IV, V, and VI served as treated groups and were given 50, 100, and 200 mg/kg body weight of F1 fraction in DMSO (2 ml/kg), respectively, for 5 d and CCl4/Olive oil on days 2 and 3. Similarly, Groups VII, VIII, and IX were given the F2 fraction, Groups X, XI, and XII were given the F3 fraction, and Groups XIII, XIV, and XV were given the F4 fraction. At the end of the experiment (day 6), blood was collected from overnight-fasted mice under mild ether anesthesia and the serum was analyzed for marker enzymes aspartate transaminase (AST) and alanine transaminase (ALT) using commercially available kits (SPINREACT) according to the manufacturer’s instructions. The animals were later sacrificed by cervical dislocation under mild ether anesthesia and the liver was excised, washed, and homogenized in ice-cold phosphate buffer (0.1 M; pH = 7) and centrifuged at 19 000g for 15 min at 4 °C. The obtained supernatant was used to determine the total protein content (Bio-Rad Protein Assay, Bio-Rad Laboratories, Inc., Berkeley, CA) and evaluate CAT, SOD, and glutathione-S-transferase (GST) activities.
CAT assay
CAT activity was assayed on the basis of H2O2 disappearance at 240 nm (Pedraza-Chaverri et al., Citation2005). A 5 µl aliquot of the 1:40 diluted supernatant was added to 720 µl of 30 mM H2O2 in 10 mM potassium phosphate solution. The decomposition of H2O2 by CAT contained in the sample was measured at 240 nm for a period of 15 s. The reaction follows first-order kinetics given by the equation , where k is the first-order reaction rate constant, t is the time over which the decrease of H2O2 was measured, and A0 and A are the optical densities at times 0 and 15 s, respectively. The results were expressed in k/mg protein.
SOD assay
SOD activity was assayed according to the method described by Naskar et al. (Citation2009). Briefly, 50 µl of the homogenized liver supernatant was added to 2.8 ml Tris-EDTA (50 mM Tris, 1.2 mM EDTA; pH = 8.5) and 100 µl of 2 mM pyrogallol. The optical density (OD) of the mixture was read at zero and 3 min at 420 nm against the control which consisted of Tris-EDTA and pyrogallol. One unit of SOD is the amount of enzyme that inhibits the rate of auto-oxidation of pyrogallol by 50%. The results were calculated according to the following equations:
GST assay
GST activity was assayed according to the method described by Al-Saffar et al. (Citation2011). Two solutions were prepared (sample and reference) each of 3 ml from the following: 2.2 ml of 0.1 M potassium phosphate buffer (pH 6.5), 100 µl of 30 mM CDNB (1-chloro-2,4-dinitrobenzene), and 100 µl of 30 mM glutathione reduced (GSH). The solutions were allowed to stand for 1 min at room temperature (25 °C), and 600 µl of the liver supernatant was added to the sample solution and mixed thoroughly. The absorbance was read at 340 nm after 1 min and the activity of GST was expressed in nmol of GSH-CDNB conjugates formed/min/mg protein by using molar extinction coefficient 9.6 mM−1 cm−1.
Statistical analysis
Data were analyzed for statistical significance using one-way analysis of variance (ANOVA). Values of the different tested parameters within each group are presented as mean ± SEM. Significant main effect differences were tested using Tukey–Kramer’s post hoc test for multiple comparisons. All data were analyzed with the statistical package SPSS 18 (SPSS Inc., Chicago, IL), and statistical significance was considered when p < 0.05.
Results
Chemical composition of oil fractions
Column chromatography of DCOE yielded four fractions based on TLC profiles. shows the GC-MS analysis of the resulted fractions with their respective components. On one hand, the F1 fraction was predominantly composed of sesquiterpenes, mainly, α-humelene (28.2%), β-caryophyllene (17.2%), α-longipinene (7.18%), α-selinene (4.26%), γ-selinene (3.53%), β-selinene (2.86%), and β-himachalene (2.58%). The F2 fraction was markedly dominated by the sesquiterpene 2-himachalen-6-ol (61%) and noticeable amounts of three phenylpropanoids:elemicin (10.4%), (E)-methyl isoeugenol (11.8%), and methyl eugenol (2.22%). On the other hand, fewer compounds were identified in F3 and F4 fractions with α-longipinene and β-selinene being the major identified components (F3: 3.07% and 1.92%, respectively; F4: 8.18% and 5.33%, respectively).
Table 1. Main identified components (>0.5%) in the wild carrot oil fractions F1, F2, F3, and F4.
On one hand, fractions F3 and F4 were further analyzed by HPLC and their chromatograms were compared with that of the standards presented in . The chromatogram of F3 fraction () showed several peaks, at retention times of 16.45, 31.81, 34.22, and 34.59 min, corresponding to caffeic acid, luteolin, kaempferol, and apigenin, respectively. On the other hand, F4 fraction chromatogram () presented five prominent peaks out of which four were identified at retention times of 30.5, 32.02, 34.35, and 34.89 min corresponding to quercetin, luteolin, kaempferol, and apigenin, respectively. The concentrations of the identified components were calculated by using the obtained calibration curves and are listed in . Luteolin (55.85 µg/mg) and apigenin (50.75 µg/mg) are the major components of F3, while kaempferol (103.00 µg/mg) and apigenin (118.00 µg/mg) are the major components of F4. In addition, the peak at 12.5 min in the chromatogram of F3 was tentatively assigned to p-coumaric acid based on the spectral data and the relative retention time (Rodrıguez-Delgado et al., Citation2001)
Figure 1. (a) HPLC chromatograms of a standard mixture of phenolic acids. Peaks: 1 = gallic acid; 2 = chlorogenic acid; 3 = vanillic acid; 4 = syringic acid; 5 = caffeic acid; 6 = ellagic acid; 7 = myricetin; 8 = quercetin; 9 = luteolin; 10 = kaempferol; 11 = apigenin. (b and c) HPLC chromatograms of F3 and F4 oil fractions of the wild carrot extract. HPLC conditions: mobile phase, water:acetic acid:methanol; 10:2:88 (solvent A) and water:acetic acid:methanol; 90:2:8 (solvent B), flow rate; 1.5 ml/min, detection; UV at 280 nm.
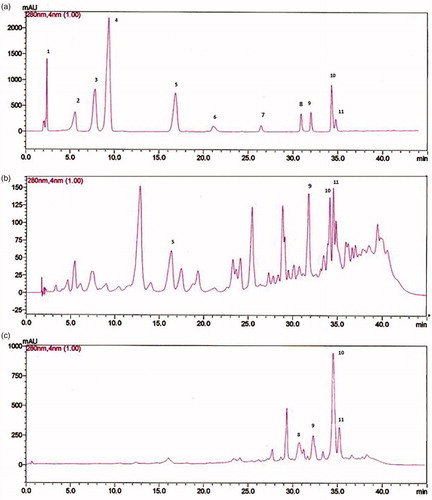
Table 2. Concentrations of phenolic acids and flavonoids in F3 and F4 fractions of the wild carrot extract.
Total phenolic and flavonoid contents
The total phenolic content of the oil fractions was measured using the Folin–Ciocalteu reagent and expressed in terms of GAE (mg GAE/g extract). F4 fraction showed the highest phenolic content, followed by F3, F1, and F2 (). The total flavonoid content was determined according to the AlCl3 colorimetric method and reported in terms of quercetin equivalents (mg QE/g extract). Flavonoid content in the different fractions displayed a similar trend to phenolics ().
Table 3. Phenolic content, flavonoid content, DPPH, FRAP, and FIC assay of the different oil fractions of Daucus carota L. ssp. carota.
In vitro assays
DPPH radical-scavenging activity
The antioxidant activity of the oil fractions was evaluated using the DPPH radical scavenging assay. The scavenging activities of the fractions at various concentrations were determined and compared with that of the standard antioxidant Trolox ( and ). The F3 fraction revealed the strongest scavenging activity among all fractions with an IC50 value of 0.29 ± 0.01 mg/ml while F1 fraction showed the lowest activity with an IC50 value >2.5 ± 0.25 mg/ml.
Figure 2. (a) Percentage DPPH scavenging activity of oil fractions (F1, F2, F3, and F4) and Trolox at different concentrations (25, 50, 100, 500, and 1000 μg/ml) on DPPH radicals. (b) The percentage chelating activity of oil fractions (F1, F2, F3, and F4) and Na2EDTA at different concentrations (0.25, 0.5, 1, 2, and 4 mg/ml) on ferrous ions. Data are presented as mean ± SEM from three experiments.
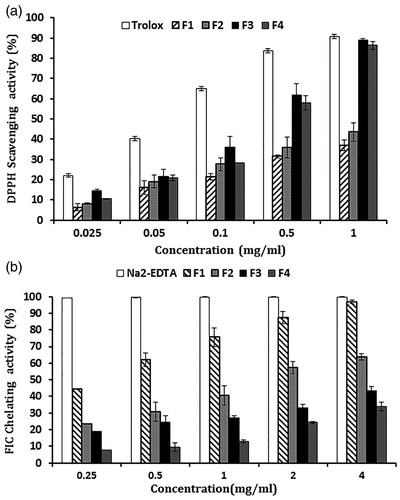
Fe2+-chelating activity
The ferrous ion-chelating (FIC) assay relies on the complex formation between ferrozine and Fe2+ ions. In the presence of other chelating substances, the ferrozine complex formation is disrupted resulting in a decrease in the red color of the complex. As shown in , the F1 fraction demonstrated the highest FIC activity among all fractions with an IC50 value = 0.28 ± 0.03 mg/ml and F4 showed the least FIC effect with an IC50 value >7.25 ± 0.45 mg/ml ().
Ferric-reducing ability
The ferric-reducing antioxidant power (FRAP) measures the ability of a substance to reduce the ferric tripyridltriazine (Fe3+–TPTZ) complex to the ferrous (Fe2+). The FRAP results () showed that the F4 fraction had the highest FRAP value (437.59 ± 6.94 µmol FeSO4/g), whereas the F1 fraction showed the lowest value (19.70 ± 0.92 µmol FeSO4/g).
In vivo assays
In vivo antioxidant activity
The in vivo antioxidant properties of DCOE fractions were assessed by determining the CAT, SOD, and GST activities in the livers of the control and experimental mice (). As expected, CCl4 treatment caused a marked decrease in the enzymatic activity of the three liver enzymes when compared with the normal control group (p < 0.05). Groups pretreated with the four oil fractions had significantly (p < 0.05) increased GST activity when compared with the CCl4-treated control group. On one hand, treatment with F1 (200 mg/kg) and F4 (50, 100, and 200 mg/kg) markedly (p < 0.05) elevated the CAT activity when compared with that of the CCl4-treated control group. Similarly, the level of SOD activity in the liver homogenates was significantly (p < 0.01) improved after treatment with F1 at 200 mg/kg and F4 at all used doses. On the other hand, groups pretreated with the F2 and F3 fractions failed to restore the normal CAT and SOD activities.
Figure 3. Effect of wild carrot oil fractions (F1, F2, F3, and F4) and vitamin E on the activity of SOD, CAT, and GST antioxidant liver enzymes of CCl4-intoxicated mice. Each column represents the mean ± SEM, with n = 6 animals in each group. (a) SOD, superoxide dismutase; (b) CAT, catalase; (c) GST, glutathione-S-transferase. ap < 0.05 compared with the normal control group; bp < 0.05; cp < 0.01; dp < 0.001 compared with the CCl4-control treated group.
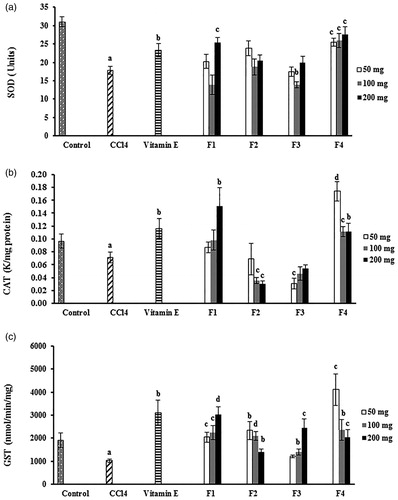
Hepatoprotective activity
The activities of serum ALT and AST for the control and experimental groups are presented in . The results indicated that the levels of ALT and AST were significantly elevated in the CCl4-treated control group compared with the normal control. Pretreated groups with F1 and F4 fractions exhibited significant (p < 0.01) hepatoprotective capacity and restored the liver enzyme activities to normal values. However, F2 and F3 fractions were less effective and showed moderate hepatoprotective activity.
Table 4. Effect of DCOE fractions and vitamin E on AST and ALT in mice after CCl4 application.
Discussion and conclusion
On one hand, phytochemical analysis of the four DCOE fractions showed that sesquiterpenes were the major constituents of F1 and F2 with the latter containing phenylpropanoids as well. On the other hand, several phenolic and flavonoid compounds were detected in F3 and F4. The major sesquiterpene in F2 (61.4%) was identified as 2-himachalen-6-ol by GCMS (NIST Library 2011, 96% match). According to the available literature (Gonny et al., Citation2004; Maxia et al., Citation2009; Mockute & Nivinskiene, Citation2004; Rossi et al., Citation2007; Staniszewska et al., Citation2005), and to the best of our knowledge, this is the first time of reporting the presence of 2-himachalen-6-ol in wild carrot species. This sesquiterpene was first isolated from the heartwood of Juniperus chinensis Linn. var. tsukusiensis Masam in Taiwan (Shiu et al., Citation1999) and this was the only available literature related to 2-himachalen-6-ol. Additionally, the sesquiterpenes β-caryophyllene (17%; F1) and α-humelene (28%; F1) present in the native Lebanese Daucus carota were either detected in trace amounts or not found in DC species worldwide. On the contrary, α-longipinene and β-himachalene appear to be limited to the Lebanese and Italian wild carrot (Gonny et al., Citation2004; Maxia et al., Citation2009; Mockute & Nivinskiene, Citation2004; Rossi et al., Citation2007; Staniszewska et al., Citation2005). These findings stress the importance of the geographical location on the chemical composition of the plant.
Recent work in our lab showed that DCOE possesses important in vitro antioxidant activity (Shebaby et al., Citation2013), and this has prompted us to investigate the in vitro and in vivo antioxidant properties of DCOE fractions. Out of the four fractions, F3 and F4 demonstrated the highest antioxidant activity in DPPH and FRAP assays. Such an effect could be partially attributed to the presence of phenolic compounds such as luteolin, kaempferol, apigenin, caffeic acid, and quercetin, all of which reported to possess antioxidant activity (Boots et al., Citation2008; Gülçin, Citation2006; Park et al., Citation2006; Škerget et al., Citation2005; Yin et al., Citation2008). Phenols, flavonoids, and terpenes are among natural non-enzymatic antioxidants, which play an important role in quenching and neutralizing free radicals and protecting against oxidative stress and tissue damage (Ajith & Janardhanan, Citation2001). The antioxidant capacity of the DCOE fractions evaluated by DPPH assay is strongly correlated with the phenolic (r = 0.93) and flavonoid (r = 0.93) contents. A high-positive correlation was also observed between FRAP antioxidant power and phenolic (r = 0.98) and flavonoid (r = 0.99) contents. Such an observation is in accordance with earlier studies which demonstrate a direct relationship between antioxidant capacity and total phenolic content in certain herbs, vegetables, and fruits (Sun et al., Citation2002; Velioglu et al., Citation1998; Zheng & Wang, Citation2001). The antioxidant activity of these phenolic compounds is believed to be attributed to the presence of hydrogen-donating hydroxyl groups on the aromatic rings (Heijnen et al., Citation2002; Kim & Lee, Citation2004). On the contrary, the ferrous ion-chelating effect of DCOE fractions did not correlate with their phenolic (r = −0.81) or flavonoid contents (r = −0.82). This is consistent with previous work that showed a similar relationship between total phenolic content and metal ion-chelating capacity of plant extracts (Wang et al., Citation2009). One possible explanation could be due to the diversity of the polyphenols’ chemical structures with respect to the number and position of phenolic hydroxyl groups which could influence their Fe2+ chelating ability (Mladěnka et al., Citation2011).
The sesquiterpene-rich fraction F1 showed moderate DPPH-scavenging activity, which could be attributed to the presence of α-humelene and β-caryophyllene, the major constituents of the F1 fraction (Legault & Pichette, Citation2007; Mayachiew & Devahastin, Citation2008; Pérez Gutiérrez et al., Citation2006; Remya et al., Citation2013). Furthermore, the sesquiterpene α-longipinene, present in F1, F3, and F4, was also found in many plants that possess antioxidant activities (Kumar et al., Citation2012; Yang et al., Citation2010). Additionally, F1 and F2 fractions were shown to possess strong Fe2+ chelating activity. Metal ions such as Fe2+ play an essential role in producing hydroxyl radicals through the Fenton reaction which accelerates lipid peroxidation (Benedet & Shibamoto, Citation2008). Therefore, F1 and F2 may have an inhibitory effect on lipid peroxidation by stabilizing the Fe2+ metal ions. β-Caryophyllene, a major compound present in the F1 fraction, was reported to inhibit significantly lipid peroxidation by reacting with peroxyl radicals (Khunkitti et al., Citation2012).
The in vivo antioxidant activity was also evaluated using CCl4-induced hepatotoxicity in mice. CCl4 is metabolized by the cytochrome P450 in the hepatic endoplasmic reticulum to produce the chemically reactive species, trichloromethyl () and trichloromethyl peroxyl (
) free radicals, which attack membrane lipids and proteins leading to lipid peroxidation and ultimately cell damage (Johnston & Kroening, Citation1998; Shenoy et al., Citation2001). As expected, mice treated with CCl4 developed significant hepatic damage marked by a substantial increase in serum levels of AST and ALT which are considered as specific markers of liver injury (Ferreira et al., Citation2010; Vozarova et al., Citation2002). However, pretreatment with F1 and F4 fractions significantly decreased the activities of these enzymes compared with the CCl4-treated control, hence minimizing hepatic damage and exerting hepatoprotective activity. Additionally, the decrease in the activity of antioxidant liver enzymes SOD and CAT caused by CCl4 treatment was reversed by F4 and to a lesser extent by F1 pretreatment. SOD is a key defense enzyme that catalyzes the conversion of
to O2 and H2O2. The latter is then metabolized by CAT into molecular oxygen and water, protecting cells against free radical induced damage (Lee et al., Citation2003). Therefore, inhibition or reduction in the activity of these protective enzymes may lead to excessive cellular damage due to the accumulation of free radicals. The antioxidant and hepatoprotective effects of DCOE fractions, mainly F1 and F4, can be attributed to the presence of sesquiterpenes in the F1 and flavonoids and phenolic compounds in the F4 fractions. These compounds may act as primary antioxidants or as free radical scavengers, resulting in the protection of these defense enzymes as shown in several previous in vivo studies (He et al., Citation2012; Jain et al., Citation2012; Naskar et al., Citation2009; Oyedemi et al., Citation2010). Furthermore, the present data showed a significant increase in the activity of GST in the four pretreated groups compared with the CCl4-treated control. GST is a phase-II metabolic enzyme that catalyzes the glutathione conjugation reaction involved in the detoxification of endogenous substances and xenobiotics providing protection against chemical toxicity and carcinogenesis (Yang et al., Citation2001).
In conclusion, the current study demonstrates the importance of DCOE fractions F3 and F4 as potential sources of antioxidants, and F1 and F4 as hepatoprotective remedies. Moreover, the results indicate that the chemical composition of the native Lebanese Daucus carota oil extract presents a unique profile from those of other origins.
Declaration of interest
The authors report no conflicts of interest. The authors alone are responsible for the content and writing of this article.
References
- Ajith TA, Janardhanan KK. (2001). Antioxidant and anti-inflammatory activities of methanol extract of Phellinus rimosus (Berk) Pilat. Indian J Exp Biol 39:1166–9
- Al-Saffar F, Ganabadi S, Fakurazi S, Yaakub H. (2011). Response of hepatic metabolizing enzymes and oxidative stress in orally administrated zerumbone against MIA-induced osteoarthritis in rats. J Anim Vet Adv 10:566–73
- Benedet J, Shibamoto T. (2008). Role of transition metals, Fe (II), Cr (II), Pb (II), and Cd (II) in lipid peroxidation. Food Chem 107:165–8
- Benzie IF, Strain J. (1996). The ferric reducing ability of plasma (FRAP) as a measure of “antioxidant power”: The FRAP assay. Anal Biochem 239:70–6
- Boots AW, Haenen GR, Bast A. (2008). Health effects of quercetin: From antioxidant to nutraceutical. Eur J Pharmacol 585:325–37
- Braca A, Sortino C, Politi M, et al. (2002). Antioxidant activity of flavonoids from Licania licaniaeflora. J Ethnopharmacol 79:379–81
- Chang CC, Yang MH, Wen HM, Chern JC. (2002). Estimation of total flavonoid content in propolis by two complementary colorimetric methods. J Food Drug Anal 10:178–82
- Coballase-Urrutia E, Pedraza-Chaverri J, Cárdenas-Rodríguez N, et al. (2011). Hepatoprotective effect of acetonic and methanolic extracts of Heterotheca inuloides against CCl4-induced toxicity in rats. Exp Toxicol Pathol 63:363–70
- Dinis TC, Madeira VM, Almeida LM. (1994). Action of phenolic derivatives (acetaminophen, salicylate, and 5-aminosalicylate) as inhibitors of membrane lipid peroxidation and as peroxyl radical scavengers. Arch Biochem Biophys 315:161–9
- Evans MD, Dizdaroglu M, Cooke MS. (2004). Oxidative DNA damage and disease: Induction, repair and significance. Mutat Res 567:1–61
- Ferreira EA, Gris EF, Felipe KB, et al. (2010). Potent hepatoprotective effect in CCl(4)-induced hepatic injury in mice of phloroacetophenone from Myrcia multiflora. Libyan J Med 5:4891
- Gonny M, Bradesi P, Casanova J. (2004). Identification of the components of the essential oil from wild Corsican Daucus carota L. using 13C-NMR spectroscopy. Flavour Frag J 19:424–33
- Gülçin İ. (2006). Antioxidant activity of caffeic acid (3,4-dihydroxycinnamic acid). Toxicology 217:213–20
- Hazra B, Biswas S, Mandal N. (2008). Antioxidant and free radical scavenging activity of Spondias pinnata. BMC Complement Altern Med 8:63
- He J, Huang B, Ban X, et al. (2012). In vitro and in vivo antioxidant activity of the ethanolic extract from Meconopsis quintuplinervia. J Ethnopharmacol 141:104–10
- Heijnen CG, Haenen GR, Minou Oostveen R, et al. (2002). Protection of flavonoids against lipid peroxidation: The structure activity relationship revisited. Free Radical Res 36:575–81
- Hemnani T, Parihar MS. (1998). Reactive oxygen species and oxidative DNA damage. Indian J Physiol Pharmacol 42:440–52
- Jain M, Kapadia R, Jadeja RN, et al. (2012). Hepatoprotective potential of Tecomella undulata stem bark is partially due to the presence of betulinic acid. J Ethnopharmacol 143:194–200
- Johnston DE, Kroening C. (1998). Mechanism of early carbon tetrachloride toxicity in cultured rat hepatocytes. Pharmacol Toxicol 83:231–9
- Khunkitti W, Veerapan P, Hahnvajanawong C. (2012). In vitro bioactivities of clove buds oil (Eugenia caryophyllata) and its effect on dermal fibroblast. Int J Pharm Pharm Sci 4:556–60
- Kim DO, Lee CY. (2004). Comprehensive study on vitamin C equivalent antioxidant capacity (VCEAC) of various polyphenolics in scavenging a free radical and its structural relationship. Crit Rev Food Sci 44:253–73
- Kumar R, Prakash O, Pant A, et al. (2012). Chemical composition, antioxidant and myorelaxant activity of essential oils of Globba sessiliflora Sims. J Essent Oil Res 24:385–91
- Lee SE, Shin HT, Hwang HJ, Kim JH. (2003). Antioxidant activity of extracts from Alpinia katsumadai seed. Phytother Res 17:1041–7
- Legault J, Pichette A. (2007). Potentiating effect of β-caryophyllene on anticancer activity of α-humulene, isocaryophyllene and paclitaxel. J Pharm Pharmacol 59:1643–7
- Leung AY. (1980). Encyclopedia of Common Natural Ingredients Used in Food, Drugs, and Cosmetics. New York, NY: Wiley
- Manda G, Nechifor MT, Neagu T-M. (2009). Reactive oxygen species, cancer and anti-cancer therapies. Curr Chem Biol 3:342–66
- Mantle D, Eddeb F, Pickering AT. (2000). Comparison of relative antioxidant activities of British medicinal plant species in vitro. J Ethnopharmacol 72:47–51
- Maxia A, Marongiu B, Piras A, et al. (2009). Chemical characterization and biological activity of essential oils from Daucus carota L. subsp. carota growing wild on the Mediterranean coast and on the Atlantic coast. Fitoterapia 80:57–61
- Mayachiew P, Devahastin S. (2008). Antimicrobial and antioxidant activities of Indian gooseberry and galangal extracts. LWT – Food Sci Technol 41:1153–9
- Medina-Holguín AL, Omar Holguín F, Micheletto S, et al. (2008). Chemotypic variation of essential oils in the medicinal plant, Anemopsis californica. Phytochemistry 69:919–27
- Mitich L. (1996). Intriguing world of weeds: Wild carrot (Daucus carota L.). Weed Technol 10:455–7
- Mladěnka P, Macáková K, Filipský T, et al. (2011). In vitro analysis of iron chelating activity of flavonoids. J Inorg Biochem 105:693–701
- Mockute D, Nivinskiene O. (2004). The sabinene chemotype of essential oil of seeds of Daucus carota L. ssp. carota growing wild in Lithuania. J Essent Oil Res 16:277–81
- Mossa A, Nawwar G. (2011). Free radical scavenging and antiacetylcholinesterase activities of Origanum majorana L. essential oil. Hum Exp Toxicol 30:1501–13
- Naskar S, Islam A, Mazumder U, et al. (2009). In vitro and in vivo antioxidant potential of hydromethanolic extract of Phoenix dactylifera fruits. J Sci Res 2:144–57
- Nehir El S, Karakaya S. (2004). Radical scavenging and iron-chelating activities of some greens used as traditional dishes in Mediterranean diet. Int J Food Sci Nutr 55:67–74
- Oyedemi S, Bradley G, Afolayan A. (2010). In vitro and in vivo antioxidant activities of aqueous extract of Strychnos henningsii Gilg. Afr J Pharm Pharmacol 4:70–8
- Park JS, Rho HS, Kim DH, Chang IS. (2006). Enzymatic preparation of kaempferol from green tea seed and its antioxidant activity. J Agric Food Chem 54:2951–6
- Pedraza-Chaverri J, Barrera D, Medina-Campos O, et al. (2005). Time course study of oxidative and nitrosative stress and antioxidant enzymes in K2Cr2O7-induced nephrotoxicity. BMC Nephrol 6:4
- Pérez Gutiérrez R, Hernández Luna H, Hernández Garrido S. (2006). Antioxidant activity of Tagetes erecta essential oil. J Chil Chem Soc 51:883–6
- Remya M, Vashum N, Sivasankar S. (2013). Bioactivity studies on Lantana camara Linn. Int J Pharm Biol Sci 4:81–90
- Rodrıguez-Delgado M, Malovaná S, Pérez J, et al. (2001). Separation of phenolic compounds by high-performance liquid chromatography with absorbance and fluorimetric detection. J Chromatogr 912:249–57
- Rossi PG, Bao L, Luciani A, et al. (2007). (E)-Methylisoeugenol and elemicin: Antibacterial components of Daucus carota L. essential oil against Campylobacter jejuni. J Agric Food Chem 55:7332–6
- Shebaby WN, El-Sibai M, Smith KB, et al. (2013). The antioxidant and anticancer effects of wild carrot oil extract. Phytother Res 27:737–44
- Shenoy KA, Somayaji S, Bairy K. (2001). Hepatoprotective effects of Ginkgo biloba against carbon tetrachloride induced hepatic injury in rats. Indian J Pharmacol 33:260–6
- Shiu LL, Chen WC, Kuo YH. (1999). Five new cis-himachalane-type sesquiterpenes from the heartwood of Juniperus chinensis var. tsukusiensis. Chem Pharm Bull 47:557–60
- Shukla S, Mehta A, Bajpai VK, Shukla S. (2009). In vitro antioxidant activity and total phenolic content of ethanolic leaf extract of Stevia rebaudiana Bert. Food Chem Toxicol 47:2338–43
- Sibanda S, Chigwada G, Poole M, et al. (2004). Composition and bioactivity of the leaf essential oil of Heteropyxis dehniae from Zimbabwe. J Ethnopharmacol 92:107–11
- Škerget M, Kotnik P, Hadolin M, et al. (2005). Phenols, proanthocyanidins, flavones and flavonols in some plant materials and their antioxidant activities. Food Chem 89:191–8
- Staniszewska M, Kula J, Wieczorkiewicz M, Kusewicz D. (2005). Essential oils of wild and cultivated carrots – The chemical composition and antimicrobial activity. J Essent Oil Res 17:579–83
- Sun J, Chu Y-F, Wu X, Liu RH. (2002). Antioxidant and antiproliferative activities of common fruits. J Agric Food Chem 50:7449–54
- Tachakittirungrod S, Okonogi S, Chowwanapoonpohn S. (2007). Study on antioxidant activity of certain plants in Thailand: Mechanism of antioxidant action of guava leaf extract. Food Chem 103:381–8
- Terao J. (2009). Dietary flavonoids as antioxidants. Forum Nutr 61:87–94
- Thomas KJ, Nicholl JP, Coleman P. (2001). Use and expenditure on complementary medicine in England: A population based survey. Complement Ther Med 9:2–11
- Velioglu Y, Mazza G, Gao L, Oomah B. (1998). Antioxidant activity and total phenolics in selected fruits, vegetables, and grain products. J Agric Food Chem 46:4113–17
- Vozarova B, Stefan N, Lindsay RS, et al. (2002). High alanine aminotransferase is associated with decreased hepatic insulin sensitivity and predicts the development of type 2 diabetes. Diabetes 51:1889–95
- Wang T, Jonsdottir R, Ólafsdóttir G. (2009). Total phenolic compounds, radical scavenging and metal chelation of extracts from Icelandic seaweeds. Food Chem 116:240–8
- Waris G, Ahsan H. (2006). Reactive oxygen species: Role in the development of cancer and various chronic conditions. J Carcinog 5:14(1–8)
- Wehbe K, Mroueh M, Daher CF. (2009). The potential role of Daucus carota aqueous and methanolic extracts on inflammation and gastric ulcers in rats. J Complement Integr Med 6:1553–3840
- Wyk B-EV, Wink M. (2004). Medicinal Plants of the World: An Illustrated Scientific Guide to Important Medicinal Plants and Their Uses. Portland, OR: Timber Press
- Yang X, Zhang H, Zhang Y, et al. (2010). Analysis of the essential oils of pine cones of Pinus koraiensis Steb. et Zucc. and P. sylvestris L. from China. J Essent Oil Res 22:446–8
- Yang Y, Cheng J-Z, Singhal SS, et al. (2001). Role of glutathione S-transferases in protection against lipid peroxidation overexpression of HGSTA2-2 in K562 cells protects against hydrogen peroxide-induced apoptosis and inhibits JNK and caspase 3 activation. J Biol Chem 276:19220–30
- Yin J, Si C-L, Wang M-H. (2008). Antioxidant activity of flavonoids and their glucosides from Sonchus oleraceus L. J Appl Biol Chem 51:57–60
- Zeinab RA, Mroueh M, Diab-Assaf M, et al. (2011). Chemopreventive effects of wild carrot oil against 7, 12-dimethylbenz(a)anthracene-induced squamous cell carcinoma in mice. Pharm Biol 49:955–61
- Zheng W, Wang SY. (2001). Antioxidant activity and phenolic compounds in selected herbs. J Agric Food Chem 49:5165–70