Abstract
Context: Cyclosporin A (CsA) is one of the most important immunosuppressive agents. However, its clinical use is strongly limited by several side effects including hepatotoxicity which remains a major clinical problem. Involvement of reactive oxygen species (ROS) in CsA-induced hepatotoxicity has been reported.
Objective: This study investigates the potential protective role of wheat germ oil (WGO) as an antioxidant against CsA-induced hepatotoxicity.
Materials and methods: Twenty-four male Wistar albino rats (six animals in each group) received castor oil, the vehicle of CsA i.p. (control) or either CsA (25 mg/kg/d i.p.), WGO (900 mg/kg/d by oral gavage), or CsA in combination with WGO daily for 21 d.
Results: CsA administration significantly increased serum levels of the liver enzymes alanine aminotransferase (ALT) and aspartate aminotransferase (AST). In addition, an increase in lipid peroxidation, inducible NO-synthase (iNOS), and NF-κB expression were observed in hepatic tissues of CsA-alone-treated rats. Furthermore, significant reduction in the hepatic content of reduced glutathione (GSH), superoxide dismutase (SOD), and catalase (CAT) was also observed in CsA-alone-treated animals. Moreover, histopathological changes occurred in CsA-alone-treated rats. Concomitant administration of WGO along with CsA improved all these parameters. Most interestingly, the immunosuppressive effect of CsA was not affected by WGO.
Conclusion: The present study suggests that concomitant use of WGO might be useful in reducing liver toxicity induced by CsA via inhibition of ROS, iNOS, and NF-κB expression.
Introduction
Cyclosporin A (CsA) is one of the most efficient immunosuppressive agents that suppress T-cell activation by inhibiting the cellular phosphatase calcineurin (Schreiber & Crabtree, Citation1992). Calcineurin inhibitors (CNI) are among the most important immunosuppressive drugs and, therefore, are commonly used in organ transplantation and for the treatment of autoimmune diseases. However, the clinical use of CsA is strongly limited by several side effects including cardiotoxicity, nephrotoxicity, and hepatotoxicity (Akool et al., Citation2008; Fellstrom, Citation2004; Mihatsch et al., Citation1998; Rezzani, Citation2006). Hepatotoxicity induced by CsA remains one of the major clinical problems. The mechanisms of CsA-induced hepatotoxicity are not fully elucidated. However, increased production of reactive oxygen species (ROS) and a consequent imbalance between oxidants and endogenously produced antioxidants have been reported to play an important role in CsA-induced hepatotoxicity (Durak et al., Citation2004; Hagar, Citation2004; Rezzani, Citation2006). ROS induced by CsA can be antagonized by using a powerful antioxidant agent (Kaya et al., Citation2008; Rezzani, Citation2006). Wheat germ oil (WGO) is an excellent source of natural vitamin E, the most powerful natural antioxidant (Ohkatsu et al., Citation2001; Shurpalekar & Rao, Citation1977; Wolf et al., Citation1998). WGO is also rich in unsaturated fatty acids, mainly oleic, linoleic, and α-linolenic acids that may inhibit oxidative stress (Alessandri et al., Citation2006; Sjovall et al., Citation2000). Furthermore, WGO is rich in functional phytochemicals, mainly flavonoids, sterols, octacosanols, and glutathione (Zhu et al., Citation2006). Recently, it has been shown that WGO intake results in a rapid increase in the level of vitamin E in different rat tissues and exerts high protection against oxidative damage (Field et al., Citation2008; Mehranjani et al., Citation2007). Thus, it was interesting to investigate the potential protective role of WGO against CsA-induced hepatotoxicity in rats.
Materials and methods
Animals
Twenty-four male Wistar albino rats weighing 190–220 g were housed in a 12 h dark/light cycle animal facility with controlled humidity and constant temperature. The animals were fed a standard diet and water was supplied ad libitum. The animals were kept under observation for 1 week before the treatments for adaptation. The experimental protocol used in this study was approved by the Institutional Animal Ethics Committee.
Drugs and chemicals
Cyclosporin A (CsA) was purchased from Sandoz Ltd, Basel, Switzerland. WGO was purchased from Sedico Pharmaceutical Co., 6 October City, Egypt. Nuclear factor kappa-B (NF-κB), inducible NO-synthase (iNOS), and catalase (CAT) ELISA kits were purchased from EIAab Science Co., Ltd., Wuhan, China. Superoxide dismutase (SOD), reduced glutathione (GSH), and thiobarbituric acid reactive substances (TBARS) assay kits were purchased from Trevigen (USA), Oxford Biomedical Research (Oxford, MI), and Cell Biolabs (San Diego, CA), respectively. Interleukin-2 (IL-2) ELISA kit was purchased from Uscn Life Science Inc, Wuhan, China. Alanine aminotransferase (ALT) and aspartate aminotransferase (AST) assay kits were purchased from Teco Diagnostics (Anaheim, CA).
Experimental design
Twenty-four male Wistar albino rats (190–220 g) were used in this study. The animals were randomly divided into four groups, six animals in each group. The first group (Control) was administered castor oil, the vehicle of CsA intraperitoneal (i.p.) daily for 21 d. The second group received CsA (25 mg/kg/d i.p.) daily for 21 d. The third group was administered WGO (900 mg/kg/d by oral gavage) 5 d before and concurrently during CsA administration daily for 21 d. The fourth group received WGO daily for 21 d. Twenty-four hours after the last treatment, blood samples were obtained for the determination of serum levels of ALT and AST as well as IL-2. After terminal bleeding, animals were sacrificed by cervical dislocation. The liver was dissected immediately after death, washed with ice-cold phosphate buffered saline (PBS), and kept at −20 °C till used. Liver specimens were fixed in 10% neutral-buffered formal saline for histopathological investigation.
Assessment of serum enzymes
Serum levels of ALT and AST were determined by commercial kits according to the manufacturer’s instructions (Teco Diagnostics, Anaheim, CA).
Assessment of serum cytokine
Serum level of IL-2 was determined by enzyme-linked immunosorbent assay (ELISA) according to the manufacturer’s instructions (Uscn Life Science Inc, Wuhan, China).
Determination of oxidative damage markers
Determination of lipid peroxides
The by-product of lipid peroxidation malondialdehyde (MDA) in hepatic tissues was measured using a TBARS assay kit according to the manufacturer’s instructions (Cell Biolabs, Inc., San Diego, CA). Briefly, tissue samples were homogenized in ice-cold PBS containing 1× BHT (butylated hydroxytoluene) and centrifugated at 10 000 g for 5 min at 4 °C to collect the supernatant. The unknown MDA containing samples or MDA standards were first reacted with thiobarbituric acid (TBA) at 95 °C. After a brief incubation, the samples and standards were measured spectrophotometrically at a wavelength of 540 nm. The standard curve was used for the calculation of the unknown samples.
Determination of iNOS expression
The level of iNOS protein expression in hepatic tissues was determined by enzyme-linked immunosorbent assay (ELISA) according to the manufacturer’s instructions (EIAab Science Co., Ltd., Wuhan, China).
Determination of NF-κB expression
Total NF-κB level in hepatic tissues was determined by enzyme-linked immunosorbent assay (ELISA) according to the manufacturer’s instructions (EIAab Science Co., Ltd., Wuhan, China).
Determination of endogenous antioxidants
Determination of GSH content
Hepatic content of GSH was determined by colorimetric assay according to the manufacturer’s instructions (Oxford Biomedical Research, Oxford, MI). Briefly, the method is based on the reaction of GSH with Ellman’s reagent [5,5′-dithiobis-2-nitrobenzoic acid (DTNB)] which gives rise to a product that can be quantified spectrophotometrically at 412 nm.
Determination of SOD activity
Hepatic SOD activity was determined with an assay kit according to the manufacturer’s instructions (Trevigen, Gaithersburg, MD). In Trevigen’s superoxide dismutase assay, superoxide radicals generated from the conversion of xanthine to uric acid and hydrogen peroxide by xanthine oxidase, converts nitroblue tetrazolium (NBT) to NBT-diformazan. NBT-diformazan absorbs light at 550 nm. SODs reduce superoxide radical concentrations and thereby lower the rate of NBT-diformazan formation. The extent of reduction in the appearance of NBT-diformazan is a measure of SOD activity.
Determination of CAT activity
Hepatic CAT activity was determined by enzyme-linked immunosorbent assay (ELISA) according to the manufacturer’s instructions (EIAab Science Co., Ltd., Wuhan, China).
Histopathological examination
Liver specimens from all animals were dissected immediately after death, and fixed in 10% neutral-buffered formal saline for at least 72 h. All the specimens were washed in tap water for 30 min and then dehydrated in ascending grades of alcohol, cleared in xylene, and embedded in paraffin. Serial sections of 6 µm thick were cut and stained with hematoxylin and eosin (Drury & Walligton, Citation1980) for histopathological investigation.
Statistical analysis
Results are expressed as means ± SD. Statistical analysis was performed using Student’s t-test, and for multiple comparisons the ANOVA test was used for significance. p Values below 0.05 were considered as indication for statistically significant differences between conditions compared.
Results
Effects of CsA and/or WGO on serum ALT and AST levels
As shown in , treatment of rats with CsA produced a significant increase in serum ALT () and AST () levels. On the other hand, concomitant administration of WGO along with CsA induced a significant reduction in ALT () and AST levels () compared with CsA-alone-treated animals. No significant changes were observed in animals treated with WGO alone.
Effects of CsA and/or WGO on oxidative damage markers
Effects of CsA and/or WGO on lipid peroxides
Treatment of rats with CsA significantly induced lipid peroxidation as indicated by an increase in the by-product of lipid peroxidation MDA. However, concomitant administration of WGO along with CsA significantly attenuated lipid peroxidation as compared with CsA-alone-treated animals (). No significant changes were observed in animals treated with WGO alone.
Effects of CsA and/or WGO on iNOS expression
As shown in , treatment of rats with CsA significantly induced iNOS expression. On the other hand, concomitant administration of WGO along with CsA significantly reduced iNOS expression as compared with CsA-alone-treated animals. The basic level of iNOS expression was not changed in animals treated with WGO alone.
Effects of CsA and/or WGO on NF-κB expression
Treatment of rats with CsA significantly induced NF-κB expression. However, concomitant administration of WGO along with CsA significantly reduced the expression of NF-κB as compared with CsA-alone-treated animals (). The expression of NF-κB was not changed in animals treated with WGO alone.
Effects of CsA and/or WGO on endogenous antioxidants
Effects of CsA and/or WGO on GSH content
As demonstrated in , treatment of rats with CsA significantly reduced the hepatic content of GSH. On the other hand, concomitant administration of WGO along with CsA significantly increased the hepatic content of GSH as compared with CsA-alone-treated animals. Hepatic GSH content in animals treated with WGO alone significantly increased compared with the control group.
Effects of CsA and/or WGO on SOD activity
As shown in , treatment of rats with CsA produced a significant reduction in SOD activity. However, concomitant administration of WGO along with CsA significantly increased the hepatic activity of SOD as compared with the CsA-alone-treated group. The SOD activity in animals treated with WGO alone significantly increased compared with the control group.
Effects of CsA and/or WGO on CAT activity
Treatment of rats with CsA significantly reduced the hepatic activity of CAT. However, concomitant administration of WGO along with CsA significantly increased the CAT activity as compared with CsA-alone-treated animals (). No significant changes were observed in animals treated with WGO alone.
Histopathological examination
As demonstrated in , histopathological examination of animals treated with CsA alone showed local hepatic area of necrosis (thin arrow) and area with deposition of hemosidrin in hepatocytes and inflammatory cells (thick arrow) (). However, concomitant administration of WGO along with CsA showed improvement at the necrotic areas (thin arrows) and deposition of hyaline material in the central vein (thick arrow) with minimal edema in the hepatic cells (). Histopathological examination of WGO-alone-treated animal showed more or less normal hepatic architecture without any pathological finding ().
Figure 4. (A) A photomicrography of normal liver tissue (H&E 100). (B) A photomicrography of liver tissue of rats received CsA showing local hepatic area of necrosis (thin arrow) and area with deposition of hemosidrin and inflammatory cells (thick arrow) (H&E 100). (C) A photomicrography of liver tissue for the third group (CsA + WGO) showing improvement at the necrotic areas (thin arrows) and deposition of hyaline material in the central vein (thick arrow) with minimal edema in the hepatic cells (H&E 200). (D) A photomicrography of liver tissue for the fourth group (WGO) showing more or less the normal hepatic architecture without any pathological finding (H&E 200).
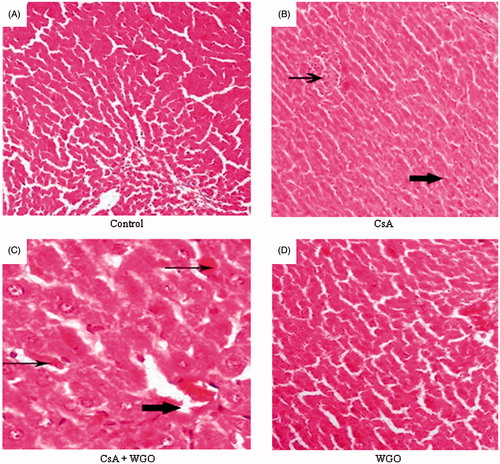
Effects of CsA and/or WGO on serum IL-2 level
Finally, to test whether the immunosuppressive effect of CsA would also be affected in the presence of WGO, serum level of IL-2 was evaluated as a marker of the immunosuppressive efficiency of CsA (Matsuda & Koyasu, Citation2000). As demonstrated in , treatment of rats with CsA produced a significant reduction in the serum level of IL-2. No significant changes were observed in animals treated with CsA in combination with WGO as compared with the CsA-alone-treated group.
Discussion
CsA is one of the most important immunosuppressive agents. It is commonly used in organ transplantation and in the treatment of autoimmune diseases. However, the clinical use of CsA is strongly limited by several side effects including hepatotoxicity which remains a major clinical problem. The mechanisms of CsA-induced hepatotoxicity are still not fully elucidated. Recently, it has been reported that increased production of ROS and consequently oxidative stress plays a major role in CsA-induced hepatic damage (Kaya et al., Citation2008; Kurus et al., Citation2009; Rezzani et al., Citation2005). Furthermore, it has been shown that antioxidants have the ability to protect against hepatotoxicity induced by CsA. In 2008, Kaya et al. reported that N-acetylcysteine (NAC) has the ability to protect against hepatotoxicity induced by CsA through its antioxidant and radical scavenging effects. In addition, it has been shown that the antioxidant melatonin plays a protective role against CsA-induced oxidative stress and hepatotoxicity in rats (Rezzani et al., Citation2005). WGO was found to contain the highest content of the most powerful natural antioxidant vitamin E than any other natural source (Ohkatsu et al., Citation2001; Shurpalekar & Rao, Citation1977; Wolf et al., Citation1998). Thus, it was interesting to investigate the potential protective role of WGO on CsA-induced hepatotoxicity. In the present work, hepatotoxicity induced by CsA was characterized by significant increase in liver enzymes (ALT and AST) and histopathological changes. Furthermore, CsA administration significantly increased hepatic MDA, iNOS, and NF-κB and decreased hepatic GSH, SOD, and CAT suggesting a role of oxidative stress in hepatotoxicity induced by CsA. High levels of serum ALT and AST indicated that there was hepatic injury. These results are in agreement with previous findings (Kaya et al., Citation2008; Pari & Sivasankari, Citation2008). Hepatic damage has been shown to be associated with lipid peroxidation induced by CsA (Rezzani, Citation2006; Pari & Sivasankari, Citation2008). Lipid peroxidation usually leads to changes in the permeability and fluidity of the biological membrane and can dramatically affect cell integrity (Dix & Aikens, Citation1993). The increased hepatic MDA level (the by-product of lipid peroxidation) in CsA-alone-treated rats may implicate oxidative damage in the liver (Rezzani, Citation2006; Pari & Sivasankari, Citation2008). Tissue injury induced by CsA has also been reported to be associated with induction of the pro-oxidant enzyme iNOS (Navarro-Antolín et al., Citation2007) that catalyze the production of NO. Furthermore, the highly reactive peroxynitrite (ONOO−) that is usually produced by the reaction between nitric oxide (NO) and superoxide () has been demonstrated to be involved in cellular damage (Josephine et al., Citation2007). In the present work, CsA was found to induce iNOS expression indicating that NO is also involved in the hepatic oxidative damage induced by CsA. The transcription factor NF-κB has been reported to be involved in the transcription of the inflammatory enzyme iNOS and other inflammatory genes (Aktan, Citation2004; Tak & Firestein, Citation2001). CsA was found to induce NF-κB expression indicating that CsA has the ability to induce not only iNOS expression but also the expression of other inflammatory genes. An efficient endogenous antioxidant defense system operates to scavenge the ROS. The most important endogenous antioxidants are GSH, SOD, and CAT. GSH plays a major role in cells protection against oxidative damage and detoxification of xenobiotics including CsA (Inselmann et al., Citation1994; Meister & Anderson, Citation1983). Also, SOD and CAT play an important role in cellular protection by scavenging ROS from the cell where SOD metabolizes superoxide radical into hydrogen peroxide (less toxic) that can be detoxified by catalase, which converts H2O2 into H2O and O2 (Deisseroth & Dounce, Citation1970; McCord et al., Citation1971). Moreover, oxidative damage induced by CsA has been shown to be associated with GSH depletion (Hagar, Citation2004) and inhibition of the antioxidant enzymes SOD and CAT (Pari & Sivasankari, Citation2008). In the present study, antioxidant capacity was highly reduced in hepatic tissues of CsA-alone-treated rats as previously demonstrated (Hagar, Citation2004; Kaya et al., Citation2008; Pari & Sivasankari, Citation2008; Rezzani et al., Citation2005). This reduction in antioxidant capacity due to the direct toxic effects of CsA leads to oxidative stress and hepatic injury. In the present work, WGO was found to protect against oxidative stress and hepatotoxicity induced by CsA as indicated by the improvement not only in the serum levels of liver enzymes but also in histopathological changes. These effects were associated with an increase in the endogenous antioxidants GSH, SOD, and CAT and inhibition of lipid peroxidation, iNOS, and NF-κB expression. Interestingly, the immunosuppressive effect of CsA was not altered by WGO. Previously, it has been reported that synthetic vitamin E does not have the same biological activity of natural vitamin E (Ingold et al., Citation1987; Pfluger et al., Citation2004). The richest known natural dietary source of vitamin E is WGO (Shurpalekar & Rao, Citation1977). Furthermore, it has been demonstrated that WGO intake results in a rapid increase in the content of vitamin E in the liver and gives powerful antioxidant protection to liver tissues (Mehranjani et al., Citation2007). In addition to the most powerful natural antioxidant vitamin E, WGO is also rich in unsaturated fatty acids, mainly oleic, linoleic, and α-linolenic acids that may exert inhibition of oxidative stress (Alessandri et al., Citation2006; Sjovall et al., Citation2000). Thus, WGO can be considered as one of the most important hepatoprotective agents that may work efficiently against various liver injuries. A daily dose of WGO is the perfect way to enrich diet with a high concentration of the most powerful natural antioxidant vitamin E that may protect the liver through its unique cytoprotective properties.
Conclusion
The findings of the present study suggest that the significant protection against CsA-induced hepatotoxicity by WGO can be attributed to its ability to restore the balance between oxygen radical production and the endogenous antioxidant defense system which was disturbed by CsA in the liver tissue. Finally, concomitant use of WGO might be useful in reducing liver toxicity induced by CsA. Additional clinical studies are warranted to investigate such an effect in human subjects.
Declaration of interest
The author reports no conflicts of interest. The author alone is responsible for the content and writing of this article.
References
- Akool El-S, Doller A, Babelova A, et al. (2008). Molecular mechanisms of TGFbeta receptor-triggered signalling cascades rapidly induced by the calcineurin inhibitors cyclosporin A and FK506. J Immunol 181:2831–45
- Aktan F. (2004). iNOS-mediated nitric oxide production and its regulation. Life Sci 75:639–53
- Alessandri C, Pignatelli P, Loffredo L, et al. (2006). Alpha-linolenic acid-rich wheat germ oil decreases oxidative stress and CD40 ligand in patients with mild hypercholesterolemia. Arterioscler Thromb Vasc Biol 26:2577–8
- Deisseroth A, Dounce AL. (1970). Catalase: Physical and chemical properties, mechanism of catalysis, and physiological role. Physiol Rev 50:319–75
- Dix TA, Aikens J. (1993). Mechanisms and biological relevance of lipid peroxidation initiation. Chem Res Toxicol 6:2–18
- Drury RVA, Walligton EA. (1980). Carltons Histological Techniques, 5th ed. New York: Oxford University Press. Pronto 206
- Durak I, Ozbek H, Elgun S. (2004). Cyclosporine reduces hepatic antioxidant capacity: Protective roles of antioxidants. Int Immunopharmacol 4:469–73
- Fellstrom B. (2004). Cyclosporine nephrotoxicity. Transplant Proc 36:220–3
- Field R, Verghese M, Walker LT, Boateng J. (2008). Feeding wheat germ meal and wheat germ oil reduced azoxymethane-induced aberrant crypt foci in fisher 344 male rats. Int J Cancer Res 4:127–36
- Hagar HH. (2004). The protective effect of taurine against cyclosporine A-induced oxidative stress and hepatotoxicity in rats. Toxicol Lett 151:335–43
- Ingold KU, Burton GW, Foster DO, et al. (1987). Biokinetics of and discrimination between dietary RRR- and SRR-alpha-tocopherols in the male rat. Lipids 22:163–72
- Inselmann G, Lawerenz HU, Nellessen U, Heidemann HT. (1994). Enhancement of cyclosporine A induced hepato- and nephrotoxicity by glutathione depletion. Eur J Clin Invest 24:355–9
- Josephine A, Amudha G, Veena CK, et al. (2007). Oxidative and nitrosative stress mediated renal cellular damage induced by cyclosporine A: Role of sulphated polysaccharides. Biol Pharm Bull 30:1254–9
- Kaya H, Koc A, Sogut S, et al. (2008). The protective effect of N-acetylcysteine against cyclosporine A-induced hepatotoxicity in rats. J Appl Toxicol 28:15–20
- Kurus M, Esrefoglu M, Sogutlu G, Atasever A. (2009). Melatonin prevents cyclosporine-induced hepatotoxicity in rats. Med Princ Pract 18:407–10
- Matsuda S, Koyasu S. (2000). Mechanisms of action of cyclosporine. Immunopharmacology 47:119–25
- McCord JM, Keele BB Jr, Fridovich I. (1971). An enzyme-based theory of obligate anaerobiosis: The physiological function of superoxide dismutase. Proc Natl Acad Sci USA 68:1024–7
- Mehranjani MS, Abnosi MH, Mahmodi M. (2007). Preventing effects of wheat germ oil on sex hormones, liver enzymes, lipids and proteins in rat serum following treatment with pnonylphenol. J Biol Sci 7:1408–11
- Meister A, Anderson ME. (1983). Glutathione. Ann Rev Biochem 52:711–60
- Mihatsch MJ, Kyo M, Morozumi K, et al. (1998). The side-effects of cyclosporine -A and tacrolimus. Clin Nephrol 49:356–63
- Navarro-Antolín J, Redondo-Horcajo M, Zaragoza C, et al. (2007). Role of peroxynitrite in endothelial damage mediated by cyclosporine A. Free Radic Biol Med 42:394–403
- Ohkatsu Y, Kajiyama T, Arai Y. (2001). Antioxidant activities of tocopherols. Polym Degrad Stabil 72:303–11
- Pari L, Sivasankari R. (2008). Effect of ellagic acid on cyclosporine A-induced oxidative damage in the liver of rats. Fund Clin Pharmacol 22:395–401
- Pfluger P, Kluth D, Landes N, et al. (2004). Vitamin E: Underestimated as an antioxidant. Redox Rep 9:249–54
- Rezzani R. (2006). Exploring cyclosporine A-side effects and the protective role-played by antioxidants: The morphological and immunohistochemical studies. Histol Histopathol 21:301–16
- Rezzani R, Buffoli B, Rodella L, et al. (2005). Protective role of melatonin in cyclosporine A-induced oxidative stress in rat liver. Int Immunopharmacol 5:1397–405
- Schreiber SL, Crabtree GR. (1992). The mechanism of action of cyclosporine A and FK506. Immunol Today 13:136–42
- Shurpalekar SR, Rao PH. (1977). Wheat germ. Adv Food Res 23:187–304
- Sjovall O, Virtalaine T, Lapvetelainen A, Kallio H. (2000). Development of rancidity in wheat germ analyzed by headspace gas chromatography and sensory analysis. J Agric Food Chem 48:3522–7
- Tak PP, Firestein GS. (2001). NF-κB: A key role in inflammatory diseases. J Clin Invest 107:7–11
- Wolf R, Wolf D, Ruocco V. (1998). Vitamin E: The radical protector. J Eur Acad Dermatol Venereol 10:103–17
- Zhu K, Zhou HM, Qian HF. (2006). Proteins extracted from defatted wheat germ, nutritional and structural properties. Cereal Chem 83:69–75