Abstract
Context: Seven dark-septate endophytic (DSE) fungi have been isolated from the roots of Epimedium wushanense T. S. Ying (Berberidaceae), an important medicinal plant with various pharmacological activities.
Objective: The current study explores the effects of seven DSE fungi on the growth and accumulation of bioactive compounds in E. wushanense.
Materials and methods: Each 1-month-old E. wushanense seedling was inoculated with one of the seven DSE fungi and was grown under greenhouse conditions for 90 d. The molecular identification of the fungi was based on the ITS1-5.8S-ITS2 nuclear ribosomal gene cluster.
Results: The results showed that the influence of DSE fungi inoculation varied between strains. Inoculation with DSE8 not only significantly enhanced plant height, root length, leaf area, leaf number, and shoot and root biomass but also improved the total flavonoid and icariin content, with an increase ranging from 20.24% to 237.97%. Three of the seven DSE fungi caused the inoculated plants to die, and the remaining three DSE strains showed neutral or negative effects on plant growth and the accumulation of bioactive compounds. According to the ITS sequence, DSE8 is congeneric to the genus Leptodontidium.
Discussion and conclusion: The findings indicate that application of DSE8 may be valuable to facilitate the cultivation of E. wushanense with a higher biomass and improved medicinal quality.
Introduction
Epimedium wushanense T. S. Ying (Berberidaceae) is a medicinal plant and the dried leaves, Epimedii wushanensis herba, are an important medicinal material commonly used in China and East Asia, with more than two thousand years of history. This plant is officially adopted in the Chinese Pharmacopeia, and its pharmacological effect in enhancing libido and sexual performance, treating cardiovascular failure, reducing blood pressure and blood glucose, and boosting anti-inflammation and immunity are well recognized (Xu et al., Citation2007).
The pharmacological activities of E. wushanense are attributed to flavonoids and polysaccharides (Li et al., Citation2012; Xu et al., Citation2007). As the major bioactive constituents of Epimedium spp. plants, the total flavonoids showed androgenic, antioxidant, antidepressant, antiosteoporosis, and antitumor activities (Wu et al., Citation2012; Zhao et al., Citation2008). Among the flavonoids, icariin has antiosteoporosis and anticancer effects and relieves cardiovascular diseases, and this is considered a quality control criterion (Pei et al., Citation2008; Wang et al., Citation2012).
To meet the increasing demand for E. wushanense in the herbal industry, artificial cultivation of E. wushanense is presently performed. Furthermore, to develop effective methods of biomass production and to obtain high-quality plant material, recent research has focused on exploring soil microorganisms beneficial to medicinal plant performance (Zubek & Błaszkowski, Citation2009).
Most medicinal plants host mutualistic arbuscular mycorrhizal (AM) and dark septate endophytic (DSE) fungi (Zhang et al., Citation2010; Zubek & Błaszkowski, Citation2009), both of which enhance plant growth, photosynthetic activity, and phosphorus content, act antagonistically towards soil-borne fungal pathogens, and modify the concentrations of the plant’s secondary metabolites (Newsham, Citation2011; Zhang et al., Citation2012; Zubek & Błaszkowski, Citation2009). Hence, recognizing mycorrhizal status, monitoring soil fungi, and selecting beneficial microbial consortia to inoculate cultivated medicinal plants could be of particular value (Zubek et al., Citation2011).
Dark septate endophytes (DSE) are conidial or sterile fungi that are likely to be ascomycetous and colonize plant roots (Jumpponen & Trappe, Citation1998). Similar to AM, DSE may play an important role in improving plant performance, especially in the case of taxa that are rarely or never colonized by AM fungi (Zubek et al., Citation2011). Previous studies showed that DSE inoculation not only promoted the growth of medicinal plants but also improved the production and quantity of the chemicals (Wu & Guo, Citation2008; Wu et al., Citation2010; Zhang et al., Citation2012). Hence, there is an increasing demand for research on using DSE fungi to improve the growth and the medicinal quality of medicinal plants.
Compared with the extensive amount of literature about the effects of AM fungi on medicinal plant species (Abu-Zeyad et al., Citation1999; Copetta et al., Citation2006; Jurkiewicz et al., Citation2010; Sawilska et al., Citation2009; Zubek et al., Citation2010), research on DSE symbioses is relatively limited. The effects of DSE fungi on plants are not as well understood and are currently the subject of much debate in the scientific community (Jumpponen & Trappe, Citation1998; Mandyam & Jumpponen, Citation2005; Newsham, Citation2011; Zhang et al., Citation2012).
To date, to the best of our knowledge, investigations of how symbiotic microorganisms affect the cultivation of high-quality E. wushanense have been conducted only rarely. In our previous work, we preliminarily characterized the dark septate endophytes (DSE) isolated from the roots of E. wushanense plants. Among 97 endophytic strains isolated from surface-sterilized E. wushanense roots, seven were found to be DSE fungi. These seven strains were labeled as DSE1, DSE2, DSE3, DSE5, DSE7, DSE8, and DSE10. Hence, present research investigates the influence of the aforementioned seven DSE fungi on the growth and bioactive constituent accumulation of E. wushanense. The results from this study will help to refine the basis for further investigation of the interaction between DSE fungi and E. wushanense as well as for an optimized cultivation protocol.
Material and methods
Sampling and isolation of endophytes
Wild E. wushanense seedlings were collected from Leishan County, Guizhou Province, China, in April 2009. The roots were washed in deionized water, sterilized in 70% ethanol for 40 s, incubated in 0.1% HgCl2 for 7 min, rinsed three times in deionized water for surface sterilization, and placed in 90 mm Petri dishes containing potato dextrose agar (PDA). The Petri dishes were sealed with parafilm and incubated at 25°C to facilitate the growth of mycelia. The mycelia excised from the root surface were subcultured and maintained on PDA media separately. Colony morphology and growth of the isolates were studied on PDA. The isolate strains were deposited at the Institute of Modern Chinese Medicinal Materials, Guizhou Academy of Agricultural Sciences in the Guizhou Province, China, where they were maintained at low temperatures (4–5°C).
Molecular identification of the DSE fungi
For molecular identification of the aforementioned seven DSE fungi, we extracted DNA from 0.2 g of fresh mycelium of each DSE fungi using the optimized CTAB method (Cubero et al., Citation1999) and amplified the internal transcribed spacer (ITS) region of the nuclear rDNA repeat using the ITS1 (5′-TCCGTAGGTGAA CCTGCGG-3′)/ITS4 (5′-TCCTCCGCTTATTGATATGC-3′) primer set (White et al., 1990). Polymerase chain reaction (PCR) was performed in 25 µL reaction volumes containing 1 µL of genomic DNA, 1 µL of each primer, 0.5 µL of each deoxynucleotide triphosphate, 2.5 µL of 10× PCR buffer (containing MgCl2), and 0.125 µL of Taq DNA polymerase (TaKaRa Bio, Madison, WI). PCR was performed in a Bio-RED with the following program: 5 min initial denaturation at 95°C, followed by 30 cycles of 40 s denaturation at 95°C, 30 s primers annealing at 54 °C, 90 s extension at 72°C, and a final 7 min extension at 72 °C. The PCR products were analyzed in 1% agarose gels (mixed with goldview) by electrophoresis and visualized under UV light. The ITS sequences were used to retrieve similar sequences from GenBank using the NCBI BLAST program. The sequence alignments and maximum parsimony analysis were carried out using Clustal 1.83 (Helix Systems, Bessemer, AL). Bootstrap percentages used to assess the support for the branching topologies were calculated using PAUP* 4.0 (Sinauer Associates Inc., Bethesda, MD) (Swofford, 1998). Bootstrap analysis was performed with 1000 replications.
Plant materials and inoculation with DSE fungi
Seeds of E. wushanense were surface sterilized with 75% ethanol for 40 s and planted onto the sterile chambers containing 50 mL of vermiculite–perlite (1:1, v/v) in November 2009. The seedlings were irrigated with 30% sterile Hoagland medium. One-month-old seedlings were transplanted into sterile chambers containing vermiculite–perlite (1:1, v/v) and sprayed with sterile Hoagland liquid medium twice a week. Each 1-month-old seedling was inoculated around the root system with four plugs of actively growing mycelium from one of the following strains: DSE1, DSE 2, DSE 3, DSE 5, DSE 7, DSE 8, and DSE 10. The uninoculated controls were inoculated with uncolonized agar. All the culturing occurred in a growth chamber at 25°C (the humidity was 62%) with a 12 h photoperiod. All of the experiments were carried out in a completely randomized block design, consisting of seven different isolates and an uninoculated control. Each treatment was replicated with 30 seedlings, and all the experiments were repeated three times.
Growth response of E. wushanense
After inoculation for 90 d, the plants were harvested. To determine the total flavonoid and icariin content, the shoots of 30 plants and the roots of 20 plants from each treatment were carefully separated and oven-dried at 60°C to constant weight before being ground until they were able to pass through a 0.5-mm sieve. Plant height, root length, leaf number, and leaf area were measured for each plant. The roots of the remaining 10 plants from each treatment were fixed in formalin–acetic acid–alcohol (FAA).
Light microscopy studies
The fresh roots of 10 plants were cut into 1 cm long pieces and fixed in FAA. To assess colonization, fine roots were cleared with 10% KOH and stained with 0.05% Trypan Blue (Phillips & Hayman, Citation1970). The preparations were observed and photographed on an Olympus microscope.
Determination of the total flavonoid and icariin content
The total flavonoid and icariin content was estimated by following the standard methods (Chinese Pharmacopeia Commission, 2005) recommended for studies using UV and HPLC.
The total flavonoid content was determined with a spectrophotometric method. The standard curve was generated by setting the UV value of icariin at 270 nm. For the sample preparation for HPLC quantitation, 0.2 g of powdered leaf samples were extracted by sonication for 1 h in 20 mL ethanol. The upper solution was filtered through a 0.45-µm organic membrane filter before injection into the HPLC system. The icariin content was determined using an HPLC system consisting of an LC-20AT Liquid Chromatograph (Shimadzu, Kyoto, Japan). The HPLC fingerprint was carried out on a C18 column (YWG-C18, 4.6 mm×150 mm, 10 μm) at 30°C with a sample injection volume of 10 μL. The detection wavelength was 270 nm.
Statistical analysis
All the experiments were repeated three times, and the data were statistically analyzed with LSD analysis of variance using SAS 9.0 for Windows (SAS Inc., Cary, NC). The data were presented as the mean ± standard error (SE).
Results
Microstructure of the dark septate endophytes
The colonies were grey to dark brown, although aerial hyphae in the center of the colonies were lighter than the submerged hyphae along the colony edges (exemplified by DSE8, ). The fungus produced no conidia or other reproductive structures in the culture media.
Figure 1. Clone characteristics and micrograph of DSE8 isolated from E. wushanense root on PDA. (A) Front of the clone; (B) back of the clone; (C) hyphae ×400.
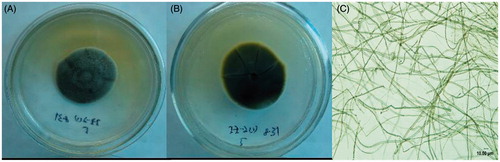
Anatomic observations of the infected roots showed that there were morphologically typical DSE structures in the roots of E. wushanense (). Melanized, septate hyphae invaded the epidermal or cortical cells. The hyphae grew parallel to the main axis of the root and from cell to cell within the cortical cells. The intracellular hyphae formed “microsclerotia” in the root cortical cells.
Figure 2. Microstructure in the roots of E. wushanense plants inoculated with different DSE strains. (A) Microsclerotia in cortical cells ×400; (B) resynthesis treatment inoculated with the DSE8 strain; (C) resynthesis treatment inoculated with the DSE10 strain; (D) resynthesis treatment inoculated with the DSE5 strain; (E) resynthesis treatment inoculated with the DSE2 strain; (a) melanized hyphae.
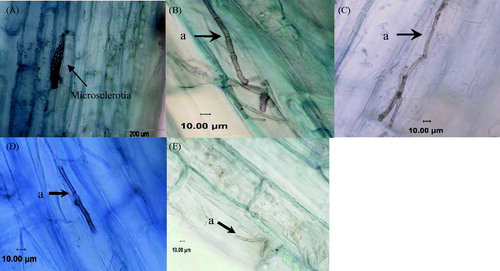
Molecular analysis of isolation
The phylogenesis of the MP tree based on the ITS1-5.8S-ITS2 rDNA is shown in . A maximum-parsimony tree of 589 steps was generated, with CI, RI, and RC indices of 0.735, 0.921, and 0.677, respectively. The results indicated that all seven DSE strains belong to the ascomycetes, and the sequences were recovered in seven sequence groups.
Figure 3. Phylogenesis analysis of seven DSE fungi isolated from E. wushanense roots. The phylogenesis of the MP tree is based on ITS1-5.8S-ITS2 rDNA. Blumeria graminis was chosen as an outgroup (tree length = 589, CI = 0.735, RI = 0.921, RC = 0.677, HI = 0.265). The number at each branch indicates the percentage of trees from 1000 bootstrap replications in which the branch occurred. Bootstraps above 50% are indicated.
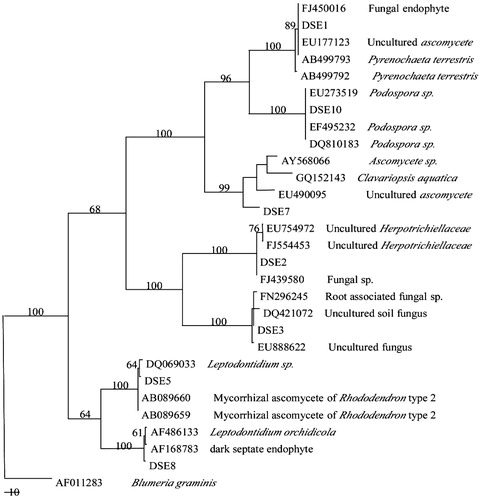
Based on the phylogenetic analysis and sequence similarity of the ITS regions, a high level of similarity in the ITS and 5.8S sequences between DSE8, Leptodontidium orchidicola, and AF168783 was observed. DSE5 was closely related to the genus Leptodontidium, while DSE10 could be placed in the genus Podospora. The remaining sequences showed high homology to a large number of currently unassigned anamorphic ascomycete sequences.
Effect of isolates on the growth of E. wushanense
The results indicated the effect of the DSE inoculation on the growth of E. wushanense plants varied with the DSE strains (). DSE5, DSE10, DSE8, and DSE1 were not pathogenic to seedlings, and the colonized E. wushanense plants were alive and healthy. In contrast, the leaves of the E. wushanense plants inoculated with DSE1, DSE3, or DSE7 became chlorotic, and the seedlings wilted and died at the end of the 90 d inoculation period (data not shown). Hereafter, we focus on the influence of DSE2, DSE5, DSE8, and DSE10 on the growth and medicinal quality of E. wushanense.
Figure 4. The growth of E. wushanense plants inoculated with different DSE strains for 90 d. CK means uninoculated control.
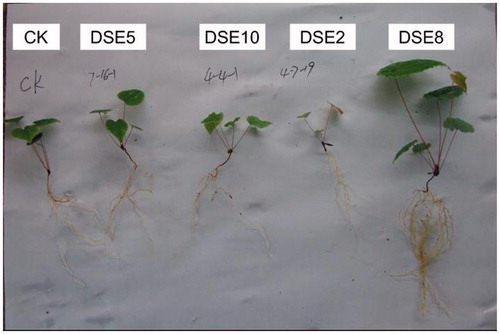
Figure 5. The effects of DSE inoculations on the shoot biomass, root biomass, plant height, and root length of E. wushanense (, n = 3). Control means uninoculated plants. Different letters indicate a significant difference at p < 0.01.
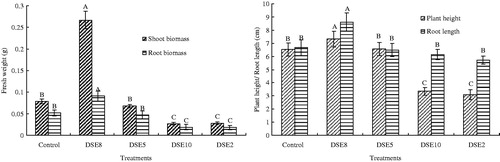
Figure 6. Effects of DSE inoculations on leaf area and leaf number of E. wushanense (, n = 3). Control means uninoculated plants. Different letters indicate a significant difference at p < 0.01.
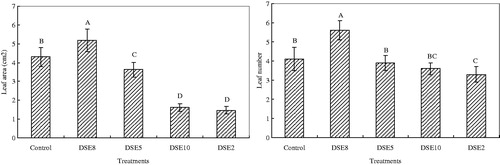
The effects of DSE2, DSE 5, DSE 8, and DSE 10 fungi on E. wushanense height, shoot fresh weight, and root fresh weight exhibited a similar trend (). DSE8 displayed a significant and positive effect compared with the uninoculated control, while DSE10 and DSE2 showed a significant negative influence. And no significant difference was found in plant height, shoot fresh weight, and root fresh weight between E. wushanense seedlings inoculated with DSE5 and the uninoculated control. Interestingly, DSE8 inoculation increased the shoot fresh weight and root fresh weight by 237.97% and 75.00%, respectively. In contrast, the shoot fresh weight in the plants inoculated with DSE2 and DSE10 decreased by 64.56% and 65.82% when compared with the uninoculated plants. And the corresponding values of root fresh weight decrease were 65.38% and 63.46%, respectively.
In the case of root length, plants inoculated with DSE2, DSE5, DSE8, and DSE10 all showed significant improvement in comparison with the uninoculated control, and there was no significant difference among the four DSE inoculation treatments. Inoculation with DSE8 fungus increased the leaf number by 36.59%, while treatment with the DSE2 fungus resulted in a 19.51% decrease in the leaf number relative to the control (). The leaf number was unaffected by DSE5 and DSE10 inoculation. The leaf area was significantly increased with DSE8 inoculation relative to the uninoculated control. In contrast, the E. wushanense seedlings inoculated with DSE2, DSE5, and DSE10 showed a significantly smaller leaf area.
Effect of isolates on the total flavonoid and icariin content of E. wushanense leaves
Compared with the uninoculated control, DSE8 treatment showed a significant, positive effect on the total flavonoids and icariin content, with a 20.24% increase in the total flavonoid content and a 42.15% increase in the icariin content (). DSE5 treatment resulted in higher total flavonoids and icariin content relative to the control; however, the difference was not significant. DSE10 and DSE2, especially DSE2, displayed a negative influence on the accumulation of the flavonoids and icariin. Total flavonoid content in DSE2 inoculated E. wushanense leaves was 85.34% of that in the control plants, while the corresponding icariin content was 36.67%.
Table 1. The effects of the DSE strains on the icariin and total flavonoid content in E. wushanense leaves (
, n = 3).
Discussion
DSE fungi exhibit a very broad host range. Muthukumar et al. (Citation2006) investigated the roots of 107 medicinal and aromatic plants (MAPs) in the Western Ghats region of Southern India for association with arbuscular mycorrhizal (AM) and dark septate endophytes (DSE). Of the 107 MAPs, belonging to 98 genera in the 52 families examined, 79 were AM and 38 harbored an association with DSE. While Zubek et al. (Citation2011) found that in 36 medicinal plant species from 33 genera and 17 families, the mycelia of DSE were observed in 13 plant species (36%); however, the percent of the roots colonized by these fungi was low. Interestingly, seven DSE isolates were found in the E. wushanense roots. Similar to the AM associations, the DSE associations vary when measured by host performance or host tissue nutrient concentrations and are negative, neutral, and positive. This range of host responses is partially attributable to variation between different fungal taxa and strains (Jumpponen, Citation2001; Menkis et al., Citation2004; Tejesvi et al., Citation2013).
In the present study, the effect of DSE fungus on the growth of E. wushanense was dependent on the DSE isolates. DSE1, DSE3, and DSE7 inoculation led to E. wushanense wilting and death during the 90-d inoculation period. The detailed mechanism of how DSE1, DSE3, or DSE7 is involved in the pathogenic process deserves further investigation.
DSE8 displayed a significant and positive influence on E. wushanense performance. DSE8 inoculation increased the shoot and root fresh biomass by 237.97% and 75.00% compared with the uninoculated control, respectively. Furthermore, E. wushanense inoculated with DSE8 had a 42.15% increase in the icariin content and a 20.24% increase in the total flavonoid content relative to the control. Our results are consistent with the work of Wu et al. (Citation2010) and Zhang et al. (Citation2012) in which the authors found DSE had positive effects on the growth and medicinal quality of the medicinal plant Saussurea involucrata Kar. et Kir and Lycium barbarum L, respectively. In contrast, DSE5, DSE10, and DSE2 showed a neutral or negative effect on plant growth and the accumulation of icariin and total flavonoids in E. wushanense leaves.
The ITS sequence analysis suggested that DSE8 was congeneric to the genus Leptodontidium. Interestingly, the DSE isolate EF-M, which is beneficial for the growth performance and medicinal quality of Saussurea involucrata, also belongs to the genus Leptodontidium (Wu et al., Citation2010).
Studies of the relationship between the DSE fungi and their host plants show little consensus and the ecological roles of DSE fungi are still poorly understood (Alberton et al., Citation2010; Mayerhofer et al., Citation2013; Vega-Frutis et al., Citation2013). Alberton et al. (Citation2010) reported a meta-analysis of data from 11 studies on the effects of DSE on shoot and root biomass. They concluded that the DSE have little or no effect on shoot biomass, and these fungi may increase root biomass. However, according to the results of Newsham (Citation2011) based on meta-analyses of data from 18 research articles, inoculation increased total, shoot and root biomasses by 52–138% when the plants had not been supplied with additional inorganic N or when all, or the majority, of the N was supplied in an organic form. In contrast, Mayerhofer et al. (Citation2013) found that the total biomass of the endophyte-inoculated plants was reduced compared with the non-inoculated controls; although individually, the shoot biomass, root biomass, and nitrogen concentration responses were neutral. The most obvious explanation for the conflicting results is the inclusion of fungi other than the dark septate endophytes. These results, however, imply that positive growth responses are more commonly elicited by the DSE (Mayerhofer et al., Citation2013).
Many studies indicated that the DSE fungi could improve nutrient uptake, increase tolerance against root pathogens, improve the ability to withstand adverse environmental conditions, produce hormones stimulating plant growth, and accelerate the mineralization of organic compounds in the soil (Addy et al., Citation2005; Alberton et al., Citation2010; Mandyam & Jumpponen, Citation2005; Mayerhofer et al., Citation2013; Newsham, Citation2011; Zubek et al., Citation2012).
With regard to the plant–microbe interactions, co-evolution between plants and their microbial partners are mediated via plant chemical defenses (Yuan et al., Citation2007). The process of colonization by fungi can cause a series of resistance reactions in host plants, including a stimulative effect on secondary metabolism such as alkaloid and terpenoid (Wu et al., Citation2010). For example, Wu and Guo (Citation2008) and Wu et al. (Citation2010) found that the DSE fungus can promote the growth of the endangered Chinese medicinal plant Saussurea involucrata and increase its rutin content. In the present work, we suggested that the increased production of total flavonoids and icariin may be a defense response to the DSE8 presence.
AM fungi have been shown to influence the content of several groups of plant metabolites in the roots and/or aerial parts of plants, e.g., alkaloids, terpenoids, carotenoids, flavonoids, glucosinolates, and phenolic acids (Zubek et al., Citation2010). Zubek et al. (Citation2010) summarized the possible mechanism as follows: first, modification of the compounds produced in the roots may be the consequence of signaling mechanisms that function between the symbiont's and plant’s responses to AM fungal colonization. Second, the enhanced production of secondary metabolites may involve several metabolic processes that may be mediated by improved P or N nutrition that is due to the AM fungal symbiosis. Finally, a possible mechanism may reside in the potential of the AM fungus to induce changes in the phytohormone levels in plants. The mechanism by which the AM fungi trigger changes in the phytochemical concentration in plant tissues may provide a reference for future research on the corresponding mechanism of DSE.
Growth promotion and secondary metabolite accumulation in plants with a plant–symbiotic fungal interaction impels the development of attractive strategies to bring medicinal plant cultivation into a new era for pharmaceutical use. Hence, the enhancement of growth and increased production of the total flavonoids and icariin is of great importance for E. wushanense cultivation. Moreover, the co-culture system of the DSE8 fungus and E. wushanense can result in a high yield of quality Epimedii wushanensis herba. Further work is needed to explore the specific mechanisms underlying the beneficial effects of the DSE8 fungi on E. wushanense plant performance and to confirm these benefits under field conditions.
Conclusions
The anatomic observations of the infected roots showed that there were typical DSE morphological structures in the roots of E. wushanense. The effects of the DSE fungus on the growth and accumulation of the total flavonoids and icariin in E. wushanense were dependent on the specific DSE isolates. There were significant positive effects of DSE8 on E. wushanense growth and intrinsic quality, while DSE2, DSE5, and DSE10 showed neutral or negative effects. In contrast, DSE1, DSE3, or DSE7 inoculation led to E. wushanense wilting and, ultimately, death. According to the ITS sequence, DSE8 belongs to the genus Leptodontidium. These data indicate that the DSE8 isolate might be used in future research with the objective of developing methods to enhance biomass production and obtain high-quality E. wushanense materials.
Declaration of interest
The authors report that they have no conflicts of interest. The authors alone are responsible for the content and writing of the paper. This study was supported financially by the National Science and Technology Support Project of China (2009BAI74B02).
References
- Abu-Zeyad R, Khan AG, Khoo C. (1999). Occurrence of arbuscular mycorrhiza in Castanospermum australe A. Cunn. & C. Fraser and effects on growth and production of castanospermine. Mycorrhiza 9:111–17
- Addy HD, Piercey MM, Currah RS. (2005). Microfungal endophytes in roots. Can J Bot 83:1–13
- Alberton O, Kuyper TW, Summerbell RC. (2010). Dark septate root endophytic fungi increase growth of Scots pine seedlings under elevated CO2 through enhanced nitrogen use efficiency. Plant Soil 328:459–70
- Chinese Pharmacopoeia Commission. (2010). Pharmacopoeia of the People's Republic of China, 2010 ed. Beijing: People's Medical Publishing House, 306
- Copetta A, Lingua G, Berta G. (2006). Effects of three AM fungi on growth, distribution of glandular hairs, and essential oil production in Ocimum basilicum L. var. Genovese. Mycorrhiza 16:485–94
- Cubero OF, Crespo A, Fatehi J, Bridge PD. (1999). DNA extraction and PCR amplification method suitable for fresh, herbarium-stored, lichenized, and other fungi. Plant Syst Evol 216:243–9
- Jumpponen A, Trappe JM. (1998). Dark septate endophytes: A review of facultative biotrophic root-colonizing fungi. New Phytol 140:295–310
- Jumpponen A. (2001). Dark septate endophytes – Are they mycorrhizal? Mycorrhiza 11:207–11.
- Jurkiewicz A, Ryszka P, Anielska T, et al. (2010). Optimization of culture conditions of Arnica montana L.: Effects of mycorrhizal fungi and competing plants. Mycorrhiza 20:293–306
- Li HF, Guan XY, Yang WZ, et al. (2012). Antioxidant flavonoids from Epimedium wushanense. Fitoterapia 83:44–8
- Mandyam K, Jumpponen A. (2005). Seeking the elusive function of the root-colonising dark septate endophytic fungi. Stud Mycol 53:173–89
- Mayerhofer MS, Kernaghan G, Harper KA. (2013). The effects of fungal root endophytes on plant growth: A meta-analysis. Mycorrhiza 23:119–28
- Menkis A, Allmer J, Vasiliauskas R, et al. (2004). Ecology and molecular characterization of dark septate fungi from roots, living stems, coarse and fine woody debris. Mycol Res 108:965–73
- Muthukumar T, Senthilkumar M, Rajangam M, Udaiyan K. (2006). Arbuscular mycorrhizal morphology and dark septate fungal associations in medicinal and aromatic plants of Western Ghats, Southern India. Mycorrhiza 17:11–24
- Newsham KK. (2011). A meta-analysis of plant responses to dark septate root endophytes. New Phytol 190:783–93
- Pei LK, Sun SQ, Guo BL, et al. (2008). Fast quality control of Herba Epimedii by using Fourier transform infrared spectroscopy. Spectrochim Acta Part A 70:258–64
- Phillips JM, Hayman DS. (1970). Improved procedures for clearing roots and staining parasitic and vesicular–arbuscular mycorrhizal fungi for rapid assessment of infection. Trans Br Mycol Soc 55:157–60
- Sawilska AK, Jendrzejczak E, Welc M, Kieliszewska-Rokicka B. (2009). Influence of mycorrhizal fungi on the growth and development of sandy everlasting Helichrysum arenarium (L.) Moench. Acta Agrobot 62:67–76
- Swofford DL. (1998). PAUP*. Phylogenetic Analysis Using Parsimony (*and Other Methods). Version 4. Sunderland (MA): Sinauer Associates
- Tejesvi MV, Sauvola T, Pirttilä AM, Ruotsalainen AL. (2013). Neighboring Deschampsia flexuosa and Trientalis europaea harbor contrasting root fungal endophytic communities. Mycorrhiza 23:1–10
- Vega-Frutis R, Varga S, Kytöviita MM. (2013). Sex-specific interaction between arbuscular mycorrhizal and dark septate fungi in the dioecious plant Antennaria dioica (Asteraceae). Plant Biol 15:558–65
- Wang LB, Wang XB, Kong LY. (2012). Automatic authentication and distinction of Epimedium koreanum and Epimedium wushanense with HPLC fingerprint analysis assisted by pattern recognition techniques. Biochem Syst Ecol 40:138–45
- White TJ, Bruns T, Lee S, Taylor JW. (1990). Amplification and direct sequencing of fungal ribosomal RNA genes for phylogenetics. In: Innis MA, Gelfand DH, Sninsky JJ, White TJ, eds. PCR Protocols: A Guide to Methods and Applications. New York: Academic Press, Inc., 315–22
- Wu B, Chen Y, Huang JH, et al. (2012). Icariin improves cognitive deficits and activates quiescent neural stem cells in aging rats. J Ethnopharmacol 142:746–53
- Wu LQ, Guo SX. (2008). Interaction between an isolate of dark-septate fungi and its host plant Saussurea involucrata. Mycorrhiza 18:79–85
- Wu LQ, Lv YL, Meng ZX, et al. (2010). The promoting role of an isolate of dark-septate fungus on its host plant Saussurea involucrata Kar. et Kir. Mycorrhiza 20:127–35
- Xu YQ, Li ZZ, Wang Y, Huang HW. (2007). Allozyme diversity and population genetic structure of three medicinal Epimedium species from Hubei. J Genet Genomics 34:56–71
- Yuan ZL, Dai CC, Chen LQ. (2007). Regulation and accumulation of secondary metabolites in plant-fungus symbiotic system. Afr J Biotech 6:1266–71
- Zhang HH, Tang M, Chen H, et al. (2010). Arbuscular mycorrhizas and dark septate endophytes ccolonization status in medicinal plant Lycium barbarum L. in arid Northwestern China. Afr J Microbiol Res 4:1914–20
- Zhang HH, Tang M, Chen H, Wang YJ. (2012). Effects of a dark-septate endophytic isolate LBF-2 on the medicinal plant Lycium barbarum L. J Microbiol 50:91–6
- Zhao HY, Sun JH, Fan MX, et al. (2008). Analysis of phenolic compounds in Epimedium plants using liquid chromatography coupled with electrospray ionization mass spectrometry. J Chromatogr A 1190:157–81
- Zubek S, Błaszkowski J. (2009). Medicinal plants as hosts of arbuscular mycorrhizal fungi and dark septate endophytes. Phytochem Rev 8:571–80
- Zubek S, Błaszkowski J, Mleczko P. (2011). Arbuscular mycorrhizal and dark septate endophyte associations of medicinal plants. Acta Soc Bot Pol 80:285–92
- Zubek S, Stefanowicz AM, Błaszkowski J, et al. (2012). Arbuscular mycorrhizal fungi and soil microbial communities under contrasting fertilization of three medicinal plants. Appl Soil Ecol 59:106–15
- Zubek S, Stojakowska A, Anielska T, Turnau K. (2010). Arbuscular mycorrhizal fungi alter thymol derivative contents of Inula ensifolia L. Mycorrhiza 20:497–504