Abstract
Context: Kaempferol is a flavonoid found in many edible plants (e.g. tea, cabbage, beans, tomato, strawberries, and grapes) and in plants or botanical products commonly used in traditional medicine. Numerous preclinical studies have shown that kaempferol have a wide range of pharmacological activities, including antioxidant, anti-inflammatory, anticancer, cardioprotective, neuroprotective, and antidiabetic activities.
Objective: The present study investigates the effect of kaempferol on membrane-bound ATPases in erythrocytes and in liver, kidney, and heart of streptozotocin (STZ)-induced diabetic rats.
Materials and methods: Diabetes was induced into adult male albino rats of the Wistar strain, by intraperitoneal administration of STZ (40 mg/kg body weight (BW)). Kaempferol (100 mg/kg BW) or glibenclamide (600 µg/kg BW) was administered orally once daily for 45 d to normal and STZ-induced diabetic rats. The effects of kaempferol on membrane-bound ATPases (total ATPase, Na+/K+-ATPase, Ca2+-ATPase, and Mg2+-ATPase) activity in erythrocytes and in liver, kidney, and heart were determined.
Results: In our study, diabetic rats had significantly (p < 0.05) decreased activities of total ATPases, Na+/K+-ATPase, Ca2+-ATPase, and Mg2+-ATPase in erythrocytes and tissues. Oral administration of kaempferol (100 mg/kg BW) or glibenclamide (600 µg/kg BW) for a period of 45 d resulted in significant (p < 0.05) reversal of these enzymes' activities to near normal in erythrocytes and tissues when compared with diabetic control rats.
Discussion and conclusion: Thus, obtained results indicate that administration of kaempferol has the potential to restore deranged activity of membrane-bound ATPases in STZ-induced diabetic rats. Further detailed investigation is necessary to discover kaempferol’s action mechanism.
Introduction
Diabetes mellitus (DM) is a heterogeneous metabolic disorder characterized by high levels of blood glucose with disturbances of carbohydrate, lipid, and protein metabolism resulting from defects in insulin secretion, insulin action, or both. The World Health Organization reports that the number of diabetics is expected to increase to 366 million or more by 2030 from 171 million in 2000 (Wild et al., Citation2004). In DM, higher glucose levels can cause depression of the natural antioxidant system which leads to coronary artery disease and beta destruction. And increased glucose levels lead to induced stress in diabetic patients as a result of high levels of free radicals through the non-enzymatic glycation of proteins, glucose oxidation, the subsequent oxidative degradation of glycated proteins (Maritim et al., Citation2003), and depression of the antioxidant defense system. Increased oxidative stress plays an important pathogenic role in the development and progression of diabetes and its complications (Brownlee, Citation2001).
ATPases (Na+/K+, Mg2+, and Ca2+) are membrane-bound enzymes responsible for the transport of ions through the membrane, and they thus regulate cellular volume, osmotic pressure, and membrane permeability (Reddy & Philip, Citation1992). Hyperglycemia has been shown to generate reactive oxygen species (ROS) such as superoxide radicals from autooxidation of glucose, resulting in an increase in the cellular lipid peroxidation (LPO) in diabetic patients and animals (Chang et al., Citation1993; Jain, Citation1989). Peroxidation of membrane phospholipids not only alters the lipid milieu and structure as well as the functional integrity of the cell membranes but also affects the activities of various membrane-bound enzymes including total ATPase, Mg2+-ATPase, Ca2+-ATPase, and Na+/K+-ATPase (Hazarika & Sarkar, Citation2001; Rauchcova et al., Citation1995). Because membrane integrity is important for cell viability, the present study was undertaken to demonstrate the membrane-protective effect of the antihyperglycmic drug kaempferol in STZ-induced rats.
Flavonoids are a group of naturally occurring compounds widely distributed as secondary metabolites in the plant kingdom. They have been recognized for displaying interesting clinical properties such as anti-inflammatory, antiallergic, antiviral, antibacterial, and antitumoral activities (Middleton, Citation1998). Kaempferol (), a flavonoid, naturally occurs in a variety of fruits, vegetables, wine, and tea. It can be isolated from tea, broccoli, witch-hazel, propslis, grapefruit, and other plants (Yoshida et al., Citation2008). The medicinal properties of kaempferol include antioxidant, anti-inflammatory, and anticancer activities (Kampkötter et al., Citation2007; Kim et al., Citation2010; Nguyen et al., Citation2003). Several studies have shown that intake of foods containing kaempferol is associated with reductions in mortality, the incidence of myocardial infarction, and the incidence of cerebrovascular disease, as well as with a slightly reduced risk of coronary heart disease (Geleijnse et al., Citation2002; Knekt et al., Citation2002; Lin et al., Citation2006). Previously, in an in vitro study, it was shown that kaempferol ameliorates hyperglycemia by improving insulin-stimulated glucose uptake in adipocytes (Fang et al., Citation2008). Kaempferol also performs a beneficial role in diabetes by preventing oxidative damage in pancreatic β cells (Lee et al., Citation2010).
Glibenclamide are widely used to treat type 2 DM and also used for standard antidiabetic drug for the experimental animal model because they stimulate insulin secretion from pancreatic β-cells. In our experimental study, diabetes was induced in adult male albino rats of the Wistar strain, by STZ. STZ inhibits insulin secretion and causes a state of insulin-dependent diabetes mellitus. A previous study, Ramesh and Pugalendi (Citation2007) used the dose of 600 µg/kg glibenclamide as a standard drug for experimental STZ-induced diabetic rats. For that, in this study, we are using glibenclamide as a standard drug for comparison of efficacy of kaempferol versus glibenclamide-treated diabetic rats.
No study has been conducted on the effect of kaempferol on membrane-bound ATPases activity in streptozotocin (STZ)-induced diabetic rats. Hence, in the present study, we examined the effects of administering kaempferol on the activity of membrane-bound enzymes such as Na+/K+-ATPase, Ca2+-ATPase, and Mg2+-ATPase in erythrocytes and tissues in STZ-induced diabetic rats and the efficacy of kaempferol was compared with glibenclamide, a standard antidiabetic drug.
Materials and methods
Drugs and chemicals
STZ and kaempferol were purchased from Sigma-Aldrich (St. Louis, MO). All other chemicals were of analytical grade.
Experimental animals
Male albino rats of Wistar strain of body weight (BW) ranging from 180 to 200 g were procured from Central Animal House, King Saud University, and they were maintained in an air-conditioned room (25 ± 1 °C) with a 12 h light/dark cycle. The animals were fed ad libitum with normal laboratory pellet diet used in the study and procedures involving animals and their care were accordance with the Policy of Research Centre, King Saud University.
Experimental induction of diabetes
The animals were made diabetic by a single intraperitoneal injection of streptozotocin (STZ, 40 mg/kg BW, between 8:00 am and 9:00 am) in a freshly prepared citrate buffer (0.1 M, pH 4.5) after an overnight fast. STZ-injected animals were given 20% glucose solution for 24 h to prevent initial drug-induced hypoglycemic mortality. Diabetes was confirmed by measuring the fasting plasma glucose concentration 96 h after induction. Albino rats with a plasma glucose level above 220 mg/dL were considered diabetic and were used in this experiment.
Experimental design
The animals were randomly divided into five groups consisting of six animals each. Kaempferol or glibenclamide was dissolved in 5% DMSO and administered by intubation (p.o.) once a day, between 9 am and 10 am, for 45 d. The oral LD50 value of kaempferol was reported at 980 mg/kg in rats (Anonymous, Citation2011). Therefore, in our previous studies, we have chosen 50, 100, and 200 mg/kg doses of kaempferol for testing the glucose lowering action (Al-Numair et al., Citation2013), which is relatively safe and can achieve the maximum protective activity in STZ-induced diabetic rats. Of the three doses of kaempferol (50, 100, and 200 mg/kg BW), 100 mg gave the maximum improvement in plasma glucose and insulin (Al-Numair et al., Citation2013). Hence, the active dose of 100 mg was used in this study:
Group I: normal control rats (5% DMSO alone)
Group II: normal rats + kaempferol (100 mg/kg BW)
Group III: diabetic control
Group IV: diabetic rats + kaempferol (100 mg/kg BW)
Group V: diabetic rats + glibenclamide (600 µg/kg BW)
After 45 d of administration of kaempferol and glibenclamide, the rats were fasted for 12 h, anesthetized by ketamine (24 mg/kg BW via intramuscular injection) and sacrificed by decapitation. Blood was collected in the tubes with EDTA and erythrocytes were separated by washing with 0.15 M sodium chloride solution. Erythrocytes and tissues (liver, kidney, and heart) were collected for the measurement of membrane bound ATPases such as total ATPases, (Na+/K+)-ATPase, Ca2+-ATPase, and Mg2+-ATPase. We have prepared Tris buffer using redistilled water and tissues were homogenized in Tris buffer and redistilled water was used throughout the experiment to avoid interference prior to phosphorous estimation in the assay of ATPases.
Biochemical assays
Estimation of total protein
Total protein was estimated by the method of Lowery et al. (Citation1951). The standard ranging from 0.2 to 1.0 ml containing 20–100 μg of protein was taken in different test tubes. The volume in each test tube was made up to 1 ml with distilled water and 1 ml of water was taken as a blank. Alkaline copper reagent (5 ml) was added to each tube and mixed thoroughly. The test tubes were allowed to stand at room temperature for 10 min. Folins–Ciocalteu reagent (0.5 ml) was added to each test tube rapidly and mixed thoroughly. After incubation at room temperature, the color developed was read against the blank at 680 nm and 0.1 ml of the sample was treated similarly. The level of total protein was expressed as mg/g of wet tissue.
Estimation of total ATPases
Total ATPase was assayed by the method of Evans (Citation1969). To 1.5 ml of buffer, 0.1 ml of each NaCl, KC1, MgCl2, CaCl2, and ATP solutions were added. Then 0.1 ml of sample was added. The tubes were incubated at 37 °C for 20 min. The reaction was arrested by addition 1 ml of 10% TCA. The tubes were centrifuged and the phosphorous content in the supernatant was determined by the Fiske and Subbarow method (Citation1925) using commercial diagnostic kit (Qualigens Diagnostics, Mumbai, India). The supernatant and aliquots of standards were made up to 5.0 ml with water. To these test tubes, 1.0 ml ammonium molybdate followed by the 0.5 ml ANSA reagent were added and mixed. The amount of phosphorous liberated was read at 620 nm after 20 min against a reagent blank in a colorimeter. The activity of total ATPases was expressed as μmoles of phosphorous liberated/h/mg protein.
Estimation of Na+K+-ATPase
Na+/K+-ATPase was assayed according to the method of Bonting (Citation1970). To 1 ml of Tris buffer, 0.2 ml of each of MgSO4, KC1, NaCl, and EDTA were added and equilibrated at 37 °C for 10 min and the enzyme reaction was initiated by the addition of 0.1 ml of sample. The assay medium was then incubated for 1 h at 37 °C. The reaction was arrested by the addition of 1 ml of 10% TCA. The tubes were centrifuged and the phosphorous content in the supernatant was estimated according to the method of Fiske and Subbarow (Citation1925) using commercial diagnostic kit (Qualigens Diagnostics, Mumbai, India). The activity of Na+/K+-ATPase was expressed as μmoles of phosphorous liberated/h/mg protein.
Estimation of Ca2+-ATPase
Ca2+-ATPase was assayed by the method of Hjerten and Pan (Citation1983). The incubation mixture contained 0.1 ml each buffer, CaCl2ATP and water. After equilibrating the tubes at 37 °C, the reaction was initiated by the addition of 0.1 ml of sample. The contents were incubated at 37 °C for half an hour. The reaction was arrested by the addition of 1 ml of cold 10% TCA. The tubes were centrifuged and the phosphorous content in the supernatant was estimated by Fiske and Subbarow method (Citation1925) using commercial diagnostic kit (Qualigens Diagnostics, Mumbai, India). The activity of Ca2+-ATPase was expressed as μmoles of phosphorous liberated/h/mg protein.
Estimation of Mg2+-ATPase
The activity of Mg2+-ATPase was assayed by the method of Ohnishi et al. (Citation1982). The incubation mixture contained 0.1 ml of 375 mM Tris-HCl buffer (pH 7.6), 0.1 ml of 25 mM MgCl2, 0.1 ml of 10 mM ATP, 0.1 ml water, and 0.1 ml of sample. The contents were incubated for 15 min at 37 °C and the reaction was arrested by adding 0.5 ml of 10% TCA. The tubes were centrifuged and the phosphorous content in the supernatant was determined by the Fiske and Subbarow method (Citation1925) using commercial diagnostic kit (Qualigens Diagnostics, Mumbai, India). The activity of Mg2+-ATPase was expressed as μmoles of phosphorous liberated/h/mg protein.
Statistical analysis
Statistical analysis was performed using one-way analysis of variance (ANOVA) followed by Duncan's multiple range test (DMRT) using SPSS software package 9.05 (SPSS Inc., Chicago, IL). Results were expressed as mean ± standard deviation (SD) from six rats in each group. p values <0.05 were considered as significant.
Results
and represent the activity of total ATPase and Na+/K+-ATPase in erythrocytes and tissues (liver, kidney, and heart) of normal and STZ-diabetic rats. In diabetic rats, the activity of total ATPase and Na+/K+-ATPase was significantly (p < 0.05) lowered when compared with that of the activity in the control rats. Administration of kaempferol and glibenclamide to diabetic rats significantly (p < 0.05) elevated the activity of total ATPase in erythrocytes (129% and 145%), in liver (55% and 71%), in kidney (82% and 95%), and in heart (70% and 79%), and the activity of Na+/K+-ATPase also significantly elevated (p < 0.05) in erythrocytes (109% and 118%), in liver (121% and 137%), in kidney (82% and 104%), and in heart (65% and 80%), as compared with that of the activity in the diabetic control rats.
Figure 2. Effect of kaempferol on total ATPases in the erythrocytes and tissues of normal and STZ-induced diabetic rats. Values are given as means ± SD from six rats in each group. Values not sharing a common superscript vertically differ significantly at p < 0.05 (DMRT). a-μmol of Pi liberated per hour. Group 1: normal control; Group 2: normal + kaempferol (100 mg/kg/d); Group 3: diabetic control; Group 4: diabetic + kaempferol (100 mg/kg/d); Group 5: diabetic + glibenclamide (600 µg/kg/d).
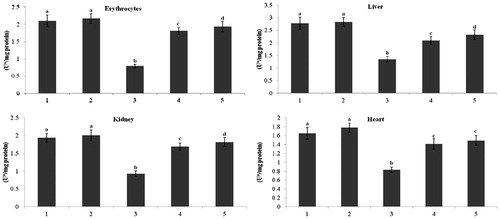
Figure 3. Effect of kaempferol on Na+/K+-ATPase in the erythrocytes and tissues of normal and STZ-induced diabetic rats. Values are given as means ± SD from six rats in each group. Values not sharing a common superscript vertically differ significantly at p < 0.05 (DMRT). a-μmol of Pi liberated per hour. Group 1: normal control; Group 2: normal + kaempferol (100 mg/kg/d); Group 3: diabetic control; Group 4: diabetic + kaempferol (100 mg/kg/d); Group 5: diabetic + glibenclamide (600 µg/kg/d).
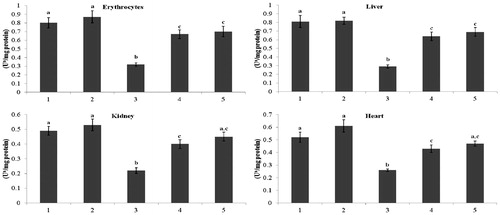
and present the activity of Ca2+-ATPase and Mg2+-ATPase in erythrocytes and tissues (liver, kidney, and heart) of normal and the STZ-diabetic rats. The diabetic rats showed decreased (p < 0.05) activity of Ca2+-ATPase and Mg2+-ATPase activity compared with that of the activity in the control rats. Administration of kaempferol and glibenclamide to diabetic rats significantly (p < 0.05) elevated the activity of Ca2+-ATPase in erythrocytes (31% and 40%), in liver (77% and 88%), in kidney (125% and 143%), and in heart (57% and 71%), and the activity of Mg2+-ATPase also significantly elevated (p < 0.05) in erythrocytes (41% and 63%), in liver (125% and 155%), in kidney (67% and 77%), and in heart (67% and 90%), when compared with that of the activity in the diabetic control rats.
Figure 4. Effect of kaempferol on Ca2+-ATPase in the erythrocytes and tissues of normal and STZ-induced diabetic rats. Values are given as means ± SD from six rats in each group. Values not sharing a common superscript vertically differ significantly at p < 0.05 (DMRT). a-μmol of Pi liberated per hour. Group 1: normal control; Group 2: normal + kaempferol (100 mg/kg/d); Group 3: diabetic control; Group 4: diabetic + kaempferol (100 mg/kg/d); Group 5: diabetic + glibenclamide (600 µg/kg/d).
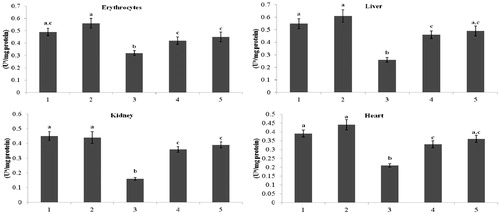
Figure 5. Effect of kaempferol on Mg2+-ATPase in the erythrocytes and tissues of normal and STZ-induced diabetic rats. Values are given as means ± SD from six rats in each group. Values not sharing a common superscript vertically differ significantly at p < 0.05 (DMRT). a-μmol of Pi liberated per hour. Group 1: normal control; Group 2: normal + kaempferol (100 mg/kg/d); Group 3: diabetic control; Group 4: diabetic + kaempferol (100 mg/kg/d); Group 5: diabetic + glibenclamide (600 µg/kg/d).
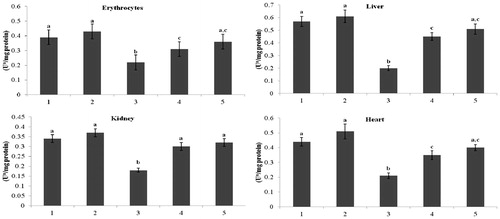
Discussion
Determination of membrane-associated enzyme activity like that of ATPases indicates membrane changes under pathological conditions (Suhail & Rizvi, Citation1987). ATPases are lipid dependent, membrane-bound enzymes involved in actively transporting sodium, potassium, calcium, and magnesium (Kimelberg, Citation1977). Enhanced susceptibility of membranes to lipid peroxides can lead to loss of protein thiol, thereby changing membrane functions (Adhirai & Selvam, Citation1997). Diabetes-induced hyperlipidemia and the alterations in membrane phospholipids and fatty acids have been shown to depress membrane-bound enzyme activity (Kuwahara et al., Citation1997). Total ATPase consists of Na+/K+-ATPase, Ca2+-ATPase, and Mg2+-ATPase. The Na+/K+ pump catalyses the hydrolysis of ATP and couples it to the transport of Na+ and K+ across the cell membrane, thereby generating the transmembranous Na+/K+ gradient. Ca2+-ATPase catalyses the active transport of Ca2+ across the cell membrane to maintain low intracellular calcium content. Mg2+-ATPase are all cell surface ATPases that hydrolyze intracellular ATP (Sabbadini & Dahms, Citation1989). Thus, the pumps are essential for the regulation of cell volume, uptake of nutrients, and cell growth and differentiation, and are also critical for normal functioning of excitable and non-excitable tissues.
STZ-induced diabetes is characterized by a severe rearrangement of subcellular metabolism and structural alteration of cell membranes, which may in turn play an important role in the development of diabetic vascular complications. The reduced activity of ATPases in erythrocytes and other tissues of STZ-induced diabetic rats have already been reported (Anandh Babu et al., Citation2006). Consistent with the previous reports, we also found decreased activity of total ATPase, Na+/K+-ATPase, Ca2+-ATPase, and Mg2+-ATPase in erythrocytes and tissues of diabetic rats. This might be associated with insulin deficiency as insulin administration partially restored Na+/K+ ATPase activity (Gupta et al., Citation1996). Insulin and catecholamines are the principal mediators of acute hormonal control of Na+/K+-ATPase (Clausen & Everts, Citation1989). Insulin is a major regulator of potassium homeostasis and has multiple effects on sodium pump activity. Within minutes of elevated insulin secretion, pumps containing α-1 and 2 isoforms have an increased affinity for sodium and increased turnover rate. Sustained elevations in insulin cause upregulation of α-2 synthesis. In skeletal muscles, insulin may also recruit pumps stored in the cytoplasm or activate latent pumps already present in the membrane. The oxidative damage of tissue lipids and proteins might be the cause of the inactivation of Na+/K+-ATPase, which is rich in thiol groups, and oxidation of thiol groups has been reported to inhibit enzyme activity (Unlucerci et al., Citation2001). The decrease in Ca2+-ATPase activity in the diabetic state may be due to altered membrane properties (Winegrad, Citation1987). It has been reported that glycosylation of membrane proteins in diabetes significantly inhibits Ca2+-ATPase activity (Ramana Devi et al., Citation1997). Insulin directly regulates the membrane bound Ca2+, Mg2+-ATPase (Hope-Gill & Nanda, Citation1979). This Mg2+-ATPase deficiency may be due to insulin deficiency as insulin regulates the enzyme.
Administration of kaempferol to diabetic rats significantly elevated the activity of total ATPase, Na+/K+-ATPase, Ca2+-ATPase, and Mg2+-ATPase in erythrocytes and tissues when compared with that of the diabetic control rats. The reversal of membrane-bound ATPase activity in diabetic rats by kaempferol treatment could be due to lowering of glucose, an increase of insulin concentrations, or antioxidant activity in the diabetic rats. The results of our previous study in which kaempferol was administered to diabetic rats showed a significantly decreased level of blood glucose and increased level of plasma insulin in the rats (Al-Numair et al., Citation2013). Previously, it was shown in an in vitro study that kaempferol ameliorated hyperglycemia by improving insulin-stimulated glucose uptake in adipocytes (Fang et al., Citation2008). Antioxidants are becoming increasingly popular in oxidative stress-related disorders and hold promise as therapeutic agents. The antioxidant compounds can counteract the decrease in ATPase activity and the increase in oxidative stress that are induced by STZ (Ashafaq et al., Citation2014). The beneficial effects of kaempferol may also be attributed to improve antioxidant activity in tissues, which potentially reduces the membrane LPO (Lee et al., Citation2010).
In conclusion, our study has shown that administration of kaempferol to diabetic rats significantly increased the activity of membrane-bound ATPases in erythrocytes, and in liver, kidney, and heart tissue. This could be due to an increase in insulin secretion, lowering of the glucose concentration, or an increase in the diabetic rats’ antioxidant defense system. Our previous study found that the administration of kaempferol to diabetic rats significantly decreased their plasma glucose and increased their insulin levels (Al-Numair et al., Citation2013). Further detailed investigation is necessary to discover kaempferol’s mechanism of action and establish its therapeutic potential in the treatment of diabetes and diabetic complications.
Declaration of interest
The authors would like to extend their sincere appreciation to the Deanship of Scientific Research at King Saud University for its funding of this research through the Research Group Project no. RGP-VPP-249.
References
- Adhirai M, Selvam R. (1997). Effect of cyclosporin A on tissue lipid peroxidation and membrane-bound phosphatases in hyperoxaluric rat and the protection by Vitamin E pre-treatment. Jpn J Med Sci Biol 50:9–17
- Al-Numair KS, Veeramani C, Alsaif MA, Chandramohan G. (2013). Influence of kaempferol on lipid metabolic changes in streptozotocin-induced diabetic rats. Prog Nutr 15:255–64
- Anandh Babu P, Sabitha KE, Shyamaladevi CS. (2006). Green tea impedes dyslipidemia, lipid peroxidation, protein glycation and ameliorates Ca2+-ATPase and Na+/K+-ATPase activity in the heart of streptozotocin-diabetic rats. Chem Biol Interact 162:157–64
- Anonymous. Available from: http://www.clearsynth.com/docs/MSD-CS-N-00277.pdf [last accessed 27 Jul 2011]
- Ashafaq M, Varshney L, Khan MH, et al. (2014). Neuromodulatory effects of hesperidin in mitigating oxidative stress in streptozotocin induced diabetes. Biomed Res Int 2014: Article ID 249031, 1--9
- Bonting SL. (1970). Sodium-potassium activated adenosine-triphosphatase and cation transport. In: C. Bittar, ed. Membrane and Iron Transport. London: Wiley Interscience, 25–8
- Brownlee M. (2001). Biochemistry and molecular cell biology of diabetic complications. Nature 414:813–20
- Chang KC, Chung SY, Chong WS, et al. (1993). Possible superoxide radical-induced alteration of vascular reactivity in aortas from streptozotocin-treated rats. Pharmacol Exp Ther 266:992–1000
- Clausen T, Everts ME. (1989). Regulation of the Na, K-pump in skeletal muscle. Kidney Int 35:1–13
- Evans WH. (1969). Membrane adenosine triphosphatase of E. coli activation by calcium ions inhibition by monovalent cations. J Bacteriol 100:914–22
- Fang XK, Gao J, Zhu DN. (2008). Kaempferol and quercetin isolated from Euonymus alatus improve glucose uptake of 3T3-L1 cells without adipogenesis activity. Life Sci 82:615–22
- Fiske CH, Subbarow Y. (1925). The colorimetric determination of phosphorus. J Biol Chem 66:375–400
- Geleijnse JM, Launer LJ, Van der Kuip DA, et al. (2002). Inverse association of tea and flavonoid intakes with incident myocardial infarction: The Rotterdam Study. Am J Clin Nutr 75:880–6
- Gupta S, Phipps K, Ruderman NB. (1996). Differential stimulation of Na+ pump activity by insulin and nitric oxide in rabbit aorta. Am J Physiol 270:H1287–93
- Hazarika A, Sarkar SN. (2001). Effect of isoproturon pre-treatment on the biochemical toxicodynamics of anilofs in male rats. Toxicology 165:87–95
- Hjerten S, Pan H. (1983). Purification and characterization of two forms of a low-affinity Ca2+-ATPase from erythrocyte membranes. Biochim Biophys Acta 728:281–8
- Hope-Gill HF, Nanda V. (1979). Stimulation of calcium ATPase by insulin, glucagon, cyclic AMP and cyclic GMP in tri-346 triton X-100 extracts of purified rat liver plasma membrane. Horm Metab Res 11:698–700
- Jain SK. (1989). Hyperglycemia can cause membrane lipid peroxidation and osmotic fragility in human red blood cells. J Biol Chem 264:21340–5
- Kampkötter A, Gombitang Nkwonkam C, Zurawski RF, et al. (2007). Effects of the flavonoids kaempferol and fisetin on thermotolerance, oxidative stress and FoxO transcription factor DAF-16 in the model organism Caenorhabditis elegans. Arch Toxicol 81:849–58
- Kim JM, Lee EK, Kim DH, et al. (2010). Kaempferol modulates pro-inflammatory NF-kappa B activation by suppressing advanced glycation end products-induced NADPH oxidase. Age (Dordr.) 32:197–208
- Kimelberg HK. (1977). The influence of membrane fluidity on the activity of membrane-bound enzymes. In: Poste G, Nicolson GL, eds. Dynamic Aspects of Cell Surface Organization, vol. 3. North-Holland, Amsterdam, New York, Oxford: Elsevier, 205–93
- Knekt P, Kumpulainen J, Jarvinen R, et al. (2002). Flavonoid intake and risk of chronic diseases. Am J Clin Nutr 76:560–8
- Kuwahara Y, Yanagishita T, Konno N, Katagiri T. (1997). Changes in microsomal membrane phospholipids and fatty acids and in activities of membrane-bound enzyme in diabetic rat heart. Basic Res Cardiol 92:214–22
- Lee YJ, Suh KS, Choi MC, et al. (2010). Kaempferol protects HIT-T15 pancreatic beta cells from 2-deoxy-D-ribose-induced oxidative damage. Phytother Res 24:419–23
- Lin J, Zhang SM, Wu K, et al. (2006). Flavonoid intake and colorectal cancer risk in men and women. Am J Epidemiol 164:644–51
- Lowery OH, Rosebrough JN, Farr AL, Randall RJ. (1951). Protein measurement with the folin phenol reagent. J Biol Chem 193:265–75
- Maritim AC, Sanders RA, Watkins JB. (2003). Diabetes, oxidative stress, and antioxidants: A review. J Biochem Mol Toxicol 17:24–38
- Middleton E Jr. (1998). Effect of plant flavonoids on immune and inflammatory cell function. Adv Exp Med Biol 439:175–82
- Nguyen TT, Tran E, Ong CK, et al. (2003). Kaempferol-induced growth inhibition and apoptosis in A549 lung cancer cells is mediated by activation of MEK-MAPK. J Cell Physiol 197:110–21
- Ohnishi T, Suzuki T, Suzuki Y, Ozawa K. (1982). A comparative study of plasma membrane magnesium ion ATPase activity in normal, regenerating and malignant cells. Biochim Biophys Acta 684:67–74
- Ramana Devi CHV, Hema Prasad M, Padmaja reddy T, Reddy PP. (1997). Glycosylation of hemoglobin and erythrocyte membrane proteins mediated changes in osmotic fragility of erythrocytes. Indian J Med Sci 51:5–9
- Ramesh B, Pugalendi KV. (2007). Influence of umbelliferone on membrane-bound ATPases in streptozotocin-induced diabetic rats. Pharmacol Rep 59:339–48
- Rauchcova H, Ledvinkova J, Kalous M, Drahota Z. (1995). The effect of lipid peroxidation on the activity of various membrane-bound ATPases in rat liver kidney. Int J Biochem Cell Biol 27:251–5
- Reddy MP, Philip HG. (1992). Changes in the levels of respiration and ions in the tissues of freshwater fish, under fenvalerate stress. Chemosphere 25:843–52
- Sabbadini RA, Dahms AS. (1989). Biochemical properties of isolated transverse tubular membranes. J Bioenerg Biomembr 21:163–213
- Suhail M, Rizvi SI. (1987). Red cell membrane (Na++K+)-ATPase in diabetes mellitus. Biochem Biophys Res Commun 146:179–86
- Unlucerci Y, Kocak H, Seferoglu G, BekpInar S. (2001). The effect of aminoquanidine on diabetes-induced inactivation of kidney (Na+/K+)-ATPase in rats. Pharmacol Res 44:95–8
- Wild S, Roglic G, Green A, et al. (2004). Global prevalence of diabetes. Diabetes Care 27:1047–53
- Winegrad AI. (1987). Does a common mechanism induce the diverse complications of diabetes? Diabetes 36:396–406
- Yoshida T, Konishi M, Horinaka M, et al. (2008). Kaempferol sensitizes colon cancer cells to TRAIL-induced apoptosis. Biochem Biophys Res Commun 375:129–33