Abstract
Context: Commiphora myrrha (Burseraceae), a shrub resembling a small tree, has been used for several centuries for the treatment of various diseases.
Objective: This study investigates the hepatoprotective activity of C. myrrha ethanol extract against d-galactosamine/lipopolysaccharide (d-GalN/LPS)-induced acute hepatic injury in an animal model.
Materials and methods: Rats were pretreated with ethanolic extract C. myrrha (250 and 500 mg/kg; p.o.) for 7 d prior to the induction of an acute phase response by d-GalN/LPS. Animals were sacrificed 24 h after d-GalN/LPS (800 mg/kg and 50 µg/kg i.p.) administration for the biochemical and histological analyses.
Results: The administration of d-GalN/LPS increased plasma aminotransferases (174.47 ± 4.5761 and 260.96 ± 1.9839 µkat/l) and total bilirubin levels (1.012 ± 0.0288 mg/dl), which were attenuated by C. myrrha treatment. Hepatic lipid peroxidation activity and nitric oxide content also increased, while the antioxidant activity measured by GSH (0.76 nmol/g protein), SOD (81.91 U/mg protein), and CAT (15.78 U/mg protein) was reduced. Commiphora myrrha provided significant restoration of GSH (0.815 nmol/gm protein), SOD (140.57 U/mg protein), and CAT (27.02 U/mg protein) levels. Furthermore, the acute phase response elicited by d-GalN/LPS administration enhanced mRNA expressions of TNF-α, IL-6, IL-10, iNOS-2, and HO-1, which were ameliorated by C. myrrha treatment.
Discussion and conclusion: These findings indicate that C. myrrha considerably reduces the oxidative stress of d-GalN/LPS-induced hepatic injury via multiple pathways including adown regulation of inflammatory mediators and cytokines. Such a property might be sufficient to combat cellular damage caused by various conditions that resemble fulminant hepatitis and could be of a potential clinical application.
Introduction
Oxidative stress causes increase of reactive oxygen species (ROS) by the activation of inducible nitric oxide synthase (NOS-2) (Diesen & Kuo, Citation2010). Heme oxygenase-1 (HO-1), glutathione peroxidase 1 (GPx1), and catalase (CAT) are important antioxidant enzymes, which along with the reactions that they catalyze, play important roles in defense against the oxidative stress induced by toxins (Farombi & Surh, Citation2006; Mari et al., Citation2009). It is also well known that ROS are critically involved in various pathological conditions such as cancer, cardiovascular disorder, arthritis, bacterial infections, and liver diseases (Gowri Shankar et al., Citation2008). Furthermore, evidence (Takemura et al., Citation1999) exists that nitric oxide (NO) is a major mediator of endotoxemia and sepsis (Takemura et al., Citation1999). As for the immune modulation, interleukin-10 (IL-10) is an important anti-inflammatory cytokine that potently inhibits the production of pro-inflammatory cytokines such as tumor necrosis factor-α (TNF-α) and interleukin-1 (IL-1) during sepsis and the associated systemic inflammatory response syndrome (SIRS) (Cassatella et al., Citation1993). In fact, IL-10 is well recognized as an important negative regulator of pro-inflammatory genes induced in LPS-stimulated macrophages by modulating both transcription and stability of specific mRNAs (Hamilton et al., Citation2002). It has also been reported that survival and apoptotic liver injury in response to SIRS are mainly dependent on secreted TNF-α signaling (Nowak et al., Citation2000).
Combination of d-galactosamine and lipopolysaccharide (d-GalN/LPS) is a well-established experimental model for studying endotoxin-related acute hepatic injury (Feng et al., Citation2007). In particular, administration of d-GalN/LPS causes TNF-α release that contributes to increased oxidative stress and formation of ROS, which are fatal to the cell and result in hepatocyte death as manifested by apoptosis and/or necrosis (Hong et al., Citation2009). In addition, d-galactosamine is a specific hepatotoxic agent that depletes the uridine triphosphate pool and thereby inhibits protein synthesis (Stachlewitz et al., Citation1999). Consequently, several studies have reported that hydrogen peroxide (H2O2) and reduced glutathione (GSH) depletion sensitize primary hepatocytes to TNFα-induced apoptosis (Han et al., Citation2006; Matsumaru et al., Citation2003).
Several in vitro and in vivo studies demonstrated the protective effect of naturally occurring antioxidant against inflammatory conditions (Yoshinari et al., Citation2014). Commiphora myrrha (Burseraceae) commonly known as Bol, Hirabol, Murmukhi in Hindi and Murr, Qafal in Arabic is a shrub resembling a small tree, found mostly in dry forest of Arabian Peninsula, Africa, and India (Miller et al., Citation1996) (). The resin of C. myrrha has been used for several centuries for the treatment of various diseases in the traditional medicines of India, China, Rome, Greece, and Babylon. The primary chemical constituents of Myrrh include the gum, resin, and essential oil (limonene, eugenol, furanosesquiterpenes, and pinene). The main active constituents include volatile oil (m-cresol, eugenol, formic acid, acetic acid, and heerabolone); resin up to 40% (including commiphoric acids); gums up to 60%, and bitter principle. Previous phytochemical investigations of C. myrrha resin revealed that terpenoids were the characteristic constituents (Su et al., Citation2009). Moreover, it has been demonstrated that some of the extracts or active principles obtained from plants of the Burseraceae family have a broad spectrum of biological activities including anti-bacterial, anti-nociceptive, anti-inflammatory, hepatoprotective, and anti-ulcer activity (Fraternale et al., Citation2011; Gowri Shankar et al., Citation2008).
In the present study, the protective effect of C. myrrha against d-GalN/LPS-induced acute hepatic injury in an animal model has been evaluated. Further, mechanism(s) of the cellular injury focusing on involvement of pro-inflammatory genes including TNF-α, IL-6, IL-10, iNOS-2, and HO-1 were investigated.
Materials and methods
Plant material and chemicals
Commiphora myrrha (Voucher # 19564) was purchased on September 2013 from Bin Menqash Spices Shop, Riyadh, and was authenticated by a taxonomist Dr. Mohammed Yousuf Yaqoob at the College of Pharmacy, King Saud University, Riyadh, Saudi Arabia. Shade dried and coarsely powdered plant gum resinous material was extracted with chilled ethanol for 48 h. The extract was then filtered, concentrated on a water bath, and dried under reduced pressure for use in the in vivo experiments. d-Galactosamine hydrochloride (d-GalN) Cat # G0500 and lipopolysaccharides from Escherichia coli 055:B5 (LPS) Cat # L-2880 were obtained from Sigma Aldrich Chemicals (St. Louis, MO). All other chemicals used were of analytical grade.
Phytochemical investigation
Gas chromatography-mass spectrometry (GC-MS) analysis was carried out at the College of Pharmacy Research Center, King Saud University, Riyadh, Saudi Arabia, to study the phytochemical components present in C. myrrha. To achieve this, a 50 g of the gum resinous material were soaked in 95% ethanol for 48 h. The extracts were then filtered through Whatmann filter paper. The filtrate was concentrated by rotary evaporator (Büchi, Oldham, UK) and 2 μl of the solutions was employed. The GC-MS analysis was performed in a Perkin Elmer (Waltham, MA), Clarus 600 gas chromatography linked to a mass spectrometer (Turbomass, Perkin Elmer, Waltham, MA) available at our Research Center, College of Pharmacy, King Saud University, Riyadh. An aliquot of 2 µl of extract was injected into the Elite −5MS column of 30 m, 0.25 mm film thickness, and 0.25 µm internal diameters. Initial oven temperature of 30 °C for 2 min, increasing to 150 °C at a rate of 30 °C for 5 min and then again increases to 300 at the rate of 5 °C for 5 min. The injector temperature was maintained at 280 °C. The interface temperature was 220 °C and the electron energy used was 70 ev. Helium was used as a mobile phase at a flow rate of 1.0 ml/min. Mass spectral detection was carried out in electron ionization mode by scanning at 40–600 (m/z). Finally, unknown compounds were identified by comparing the spectra with that of the National Institute of Standard and Technology library. The total time required for analyzing a single sample was 82 min.
Animals and study protocol
Albino male Wistar rats weighing approximately 200 ± 20 g were obtained from the Experimental Animal Care Center, College of Pharmacy, King Saud University, Riyadh, Saudi Arabia. The animals were kept under controlled environmental conditions (23 °C and a 12 h light/dark cycle). Animals were given free access to standard rat pellet food and tap water. The study was approved by Research Ethics Committee of College of Pharmacy, King Saud University (Riyadh, Saudi Arabia) (clearance no. 021692-0621; November, 2013). The animals were handled in accordance with the Guide for the Care and Use of Laboratory Animals by the Animal Care Center.
The animals were divided into five groups of six rats each. Group 1 served as a vehicle control and was administered Tween-80 in saline. Group II was induced with d-GalN/LPS (800 mg/kg and 50 µg/kg i.p., 24 h before the experiment). Group III was pretreated with 500 mg/kg C. myrrha extract for 7 d. Animals in Groups IV and V received C. myrrha, 250 and 500 mg/kg; p.o., respectively, for 7 d prior to sepsis induction by d-GalN/LPS.
Assessment of liver function
The animals were sacrificed after 24 h of d-GalN/LPS injection, and blood sample was collected. A midline abdominal incision was made and the liver was quickly harvested, perfused with cold isotonic saline, dried carefully on filter papers, weighed, and then stored at −80 °C until further enzymatic analysis and histological studies. Commercially available reagent kits for assays of liver markers such as aspartate aminotransferase (AST), alanine transaminase (ALT), and bilirubin were purchased from Human Diagnostic Worldwide (Wiesbaden, Germany), using spectrophotometer (Shimadzu – Model UV 2401, Kyoto, Japan).
Assessment of oxidative stress
On the day of biochemical estimation, the entire harvested liver was homogenized with 10% (w/v) cold phosphate-buffered saline (0.1 mol/l, pH 7.4) using a homogenizer Potter-Elvehjem type C homogenizer (Thomas Scientific, Swedesboro, NJ). The homogenates were used to estimate liver thiobarbituric acid reactive substances (TBARS) and GSH after centrifugation at 800g for 5 min at 4 °C to separate the nuclear debris. The supernatant obtained was further centrifuged at 2500g for 20 min at 4 °C to obtain the post-mitochondrial supernatant, which was used to assay superoxide dismutase (SOD) activity.
The quantitative measurement of lipid peroxidation in the liver was performed according to the method of Wills (Citation1966). The malondialdehyde content, a measure of lipid peroxidation, was assayed in the form of TBARS. In brief, the reaction mixture consisted of 0.5 ml of tissue homogenate and 0.5 ml Tris HCl (pH 7.4) and was incubated at 37 °C for 2 h. The reaction mixture was brought to 2 ml with 1 ml of 10% ice-cold trichloroacetic acid. After centrifugation at 5000g for 10 min, 1 ml of 0.67% of thiobarbituric acid was added to 1 ml of supernatant. The tubes were then kept in a boiling water-bath for 10 min and after cooling under tap water, 1 ml of distilled water was added. The absorbance was measured at 540 nm.
Assessment of antioxidant activity
GSH content in the liver was determined by the method of Jollow et al. (Citation1974) while the SOD activity was assayed by the method of Kono (Citation1978). The CAT activity was measured by the method of Aebi (Citation1974). Briefly, supernatant (0.1 ml) was added to a cuvette containing 1.9 ml of 50 mM phosphate buffer (pH 7.0). The reaction was started by the addition of 1.0 ml of freshly prepared 30 mM H2O2. The rate of decomposition of H2O2 was measured spectrophotometrically from changes in the absorbance at 240 nm. The enzymatic activities are expressed as U/mg protein.
Assessment of nitric oxide level
Production of nitric oxide was estimated by quantification of its related end products, nitrite/nitrate. In the assay, nitrate is converted to nitrite by nitrate reductase and total nitrite is measured using the Griess reaction (Green et al., Citation1982). Liver homogenates were incubated with nitrate reductase (0.2 U/ml), FAD (5 mM), and NADPH (50 mM) for 20 min at 37 °C. The reaction was stopped by the addition of sodium pyruvate (10 mM) and lactate dehydrogenase (24 mg/ml) for 5 min at 37 °C, and precipitated with 1.4% ZnSO4. Total nitrite reacted with Griess reagent (1% sulfanilamide, 2.5% PO4H3, and 0.1% n-naphthyl-ethylene-diamine) for 10 min at 37 °C was read using a 540 nm filter in a titrated Multiskan® 96-well Plate Reader (MTX Lab Systems Inc., Vienna, VA).
RNA isolation and quantification
Harvested liver samples were used for total RNA isolation by Trizol reagent (Life Technologies, Inc., Grand Island, NY). Subsequently, the reverse transcription from total RNA to cDNA was processed by high capacity cDNA reverse transcription kit of (Applied Biosystems, Carlsbad, CA) according to the instructions of the manufacturer. Concisely, RNA was diluted to 2 × reverse transcriptase (RT) master mix buffer, 2 μl dNTP (10 mM), 1 μl RNase inhibitor, and 1 μl hexamer primers were added to 10 μl of diluted RNA on ice. Prior to the addition of 2 μl of RT (200 unit/μl), the mixture was heated at 65 °C for 10 min and then snap-cooled on ice for 2 min. The reaction was carried out at 25 °C for 10 min then 37 °C for 120 min fallowed by 72 °C for 4 min. The cDNA was stored at −80 °C until further use.
Quantitative analysis of hepatic-specific mRNA expression of genes of interest was performed by real time PCR by subjecting the resulting cDNA to PCR amplification using 96-well optical reaction plates in the ABI Prism 7500 System (Applied Biosystems, Carlsbad, CA). Rat primers for TNF-α, IL-6, IL-10, HO-1, iNOS-2, and β-ACTIN gene () (Eskandari et al., Citation1999; Grilli et al., Citation2003; Ibrahim et al., Citation2008; Salazar-Montes et al., Citation2000; Siegling et al., Citation1994) were purchased from Integrated DNA technologies (IDT, Coralville, IA). The 25 µl reaction mixture contained 0.1 µl of 10 µM forward primer and 0.1 µl of 10 µM reverse primer (40 nM final concentration of each), 12.5 µl of SYBR Green Universal Master mix (Applied Biosystems, Foster City, CA), 11.05 µl of nuclease-free water, and 1.25 µl of cDNA sample. The hot start polymerase was activated by heating at 95 °C for 3 min. The cycling conditions were 1 min at 95 °C (melting) and 45 s at 60 °C (annealing and extension) for 30 cycles. The fold change in the level of mRNA between treated and untreated groups were corrected by the levels of β-ACTIN. The real-time PCR data were analyzed using the relative gene expression (i.e., the DD-Ct method) as described previously (Pfaffl et al., Citation2002). The fold change in the level of target genes between treated and untreated groups, corrected by the level of β-ACTIN, was determined using the following equation: fold change = 2−Δ(ΔCt), where ΔCt = Ct(target)−Ct(β-actin) and Δ(ΔCt) = ΔCt(treated)−ΔCt(untreated). All reactions were run in duplicate.
Table 1. List of primer sequence used in amplification of TNF-α, IL-6, IL-10, iNoS-2, HO-1, and β-actin mRNA.
Histological evaluation
Liver tissues were sliced in small pieces and immersed in neutral buffered 10% formalin for 24 h. The fixed tissues were processed routinely, embedded in paraffin (to get paraffin sections 4–5 µm), sectioned, deparaffinized, and rehydrated using the standard techniques (Bancroft and Gamble). The extent of d-GalN/LPS-induced hepatotoxicity was evaluated by assessing the morphological changes in the liver sections stained with hematoxylin and eosin was examined under microscopy.
Statistical analysis
Data are given as mean ±SEM. Differences between groups were determined by one-way ANOVA using a multiple comparison procedure (Tukey post hoc test). A p value of less than 0.05 was considered significant. All statistical analyses were performed employing Graph Pad Prism 5 (Graphpad Software, Inc., San Diego, CA).
Results
The GC-MS analysis of C. myrrha showed the presence of 88 phytochemical constituents that could contribute to the medicinal activity of the plant. Among the 88 phyto-components identified in the extract, curezerine (65%),3-[(E)-2-phenyl-1-propenyl]cyc (28.1%), bicycle [3.1.1]hept-2-ene-2-car (9%), 8-isopropenyl-9-isopropyltetra (8%), β-elemene (3.6%), 2,4-bis(3-methyl-1-pentynyl)-4 (3%), and δ-elemene (1.1%) were found to be the major constituents. These phytochemicals with of their retention time, molecular weight, and percentage peak area are illustrated in .
The administration of d-GalN/LPS to rats produced an acute hepatic injury manifested by a significant increase in ALT, AST, and bilirubin levels compared with controls (p < 0.01). Nevertheless, pretreatment with C. myrrha at 250 and 500 mg/kg doses ameliorated this injury as evident by a reduction in liver enzymes in a dose-dependent manner (). Furthermore, the histological evaluation of liver tissues showed that d-GalN/LPS caused massive fatty changes, focal central vein congestion, ballooning formation, necrosis with inflammation, and loss of cellular boundaries. Commiphora myrrha treatment improved liver histology towards more normalization especially with the 500 mg/kg dose.
Table 2. Effect of CME on plasma AST, ALT and total bilirubin level against d-GalN/LPS induced liver toxicity in rats.
The extent of lipid peroxidation as measured by the formation of TBARS level showed a sharp increase following d-GalN/LPS administration, which is indicative of increased oxidative stress in animals. Similar to the liver enzymes trend, C. myrrha treatment led to a significant dose-dependent reduction in TBARS concentrations (; p < 0.001). depicts the effect of the acute phase response due to d-GalN/LPS exposure on NO production measured by nitrite/nitrate level. Commiphora myrrha significantly attenuated this effect in a dose-dependent fashion (p < 0.001). The anti-oxidant activity measured by GSH, SOD, and CAT also increased by C. myrrha treatment, whereas the d-GalN/LPS administration produced a notable reduction in these parameters ().
Figure 3. Effect of CME (250 and 500 mg/kg; p.o.) pretreatment on hepatic lipid peroxidation (A: TBARS) and nitric oxide levels (B: NO). The results are presented as the mean ± SEM of six animals per group. “**” Denote significant differences from the control group (p < 0.01); “b” denote significant differences from the d-GalN/LPS group (p < 0.01). GalN, galactosamine; LPS, lipopolysaccharide; NO: nitric oxide; TBARS: thiobarbituric acid reactive substance.
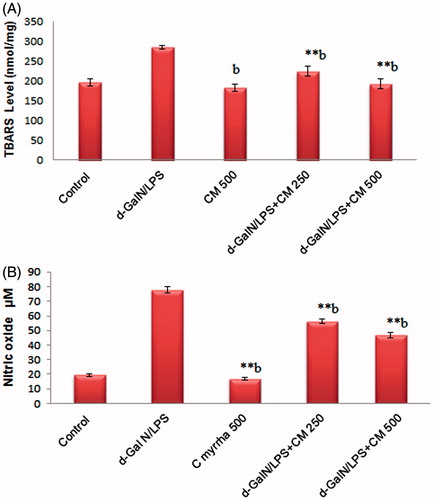
Table 3. Effect of CME on antioxidant parameters GSH, SOD, CAT, and d-GalN/LPS induced liver toxicity in rats.
Hepatic mRNA expression of TNF-α and IL-6 in response to d-GalN/LPS showed that there is an approximately four and two-fold up-regulation compared with controls. As illustrated in , rats received C. myrrha for 1 week prior to d-Gal/LPS administration exhibited an important reduction in TNF-α and IL-6 mRNA levels. IL-10 is a major anti-inflammatory cytokine that potently inhibits the production of TNFα. Treatment with C. myrrha increased mRNA expression of IL-10 as shown in . Hepatic iNOS-2 mRNA expression also increased approximately 7.3-fold following d-Gal/LPS exposure. Commiphora myrrha treatment significantly down-regulated iNOS-2 mRNA expression in d-GalN/LPS treated rats (). This closely correlates with nitrites levels measured in various groups (). Similarly, the inducible HO-1 expression was increased (8.6-fold, p ≤ 0.01) due to d-GalN/LPS (). Conversely, C. myrrha pre-treatment reduced HO-1 mRNA level (p ≤ 0.01).
Figure 4. Effect of CME (250 and 500 mg/kg; p.o.) pretreatment on hepatic mRNA expression of pro-inflammatory cytokine gene (A: TNFα, B: IL-6, C: IL-10) and inflammatory stress gene (D: iNos-2 and E: HO-1) against β-actin. The results are presented as the mean ± SEM of six animals per group. “**” Denote significant differences from the control group (p < 0.01); “b” denote significant differences from the d-GalN/LPS group (p < 0.01). d-GalN, d-galactosamine; IL, interleukin; LPS, lipopolysaccharide; TNF, tumor necrosis factor.
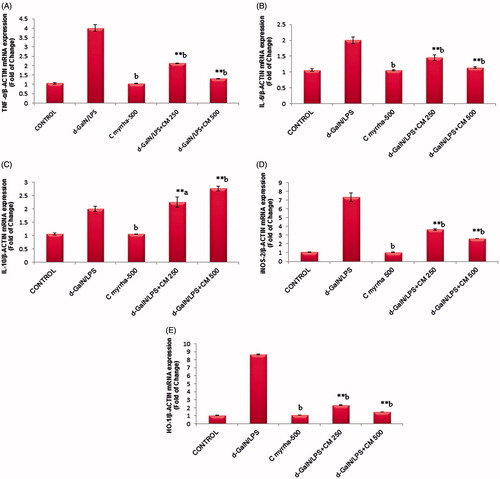
The histological observations basically support the results obtained from plasma enzyme assays. Liver section of normal control rats shows the central vein surrounded by hepatic cord of cells while d-GalN/LPS-treated rats showing massive fatty changes, focal central vein congestion, ballooning formation, necrosis with inflammation, and loss of cellular boundaries. Liver section of rats treated with d-GalN/LPS and 250 mg/kg of CME showed mild central vein congestion, ballooning, necrosis with sinusoidal dilatation and rats treated with d-GalN/LPS and 500 mg/kg of CME showing the absence of ballooning, inflammatory cells, regeneration of hepatocytes around central vein but slight congestion in central vein and almost toward near normal liver architecture and possessing higher hepatoprotective action as shown in .
Figure 5. Light photomicrographs of rat liver (hematoxylin and eosin stains, magnification 100×): (A) control rat liver section; (B) d-GalN/LPS group liver section; (C) liver section of rat pre-treated with CME 500 mg/kg; (D) liver section of rat pre-treated with CME 250 mg/kg and received d-GalN/LPS; (E) liver section of rat pre-treated with CME 500 mg/kg and received d-GalN/LPS. CME pretreatment caused improvement of liver histology as manifested with only mild central vein congestion, ballooning, and necrosis with sinusoidal dilatation. Animals treated with CME 500 mg/kg of prior to d-GalN administration, showed the absence of ballooning, inflammatory cells, regeneration of hepatocytes around central vein but slight congestion in central vein and more normalization of liver structure.
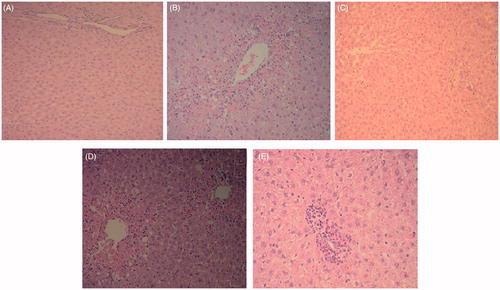
Discussion
Hepatoprotection remains one of the major problems of pharmacotherapy to limit liver dysfunction considering that impairments of the liver are a worldwide problem arises from various etiologies. Viral infection, immune-induced, alcohol, drug toxicity, or under ischemia reperfusion injuries can elicit an interaction with the liver cells that may lead to hepatic damage (Chang et al., Citation2005). A limited number of herbal remedies are used traditionally as hepatoprotectives, however, with a variable degree of success. These herbs contain flavonoids, terpens, and alkaloids and are antioxidants in general. Therefore, the use of compounds derived from herbal origin needs further experimental and clinical evaluations (Stickel & Schuppan, Citation2007). Owing to its anti-inflammatory and antioxidant activities, Commiphora opobalsamum demonstrated a hepatoprotective effect in a CCL4-treated animals (Al-Howiriny et al., Citation2004; Gowri Shankar et al., Citation2008).
The administration of d-GalN/LPS is a well-established model of LPS-induced liver injury (Motobu et al., Citation2006). Various dose combinations of LPS and d-GalN were used to produce a sub-lethal liver failure, which is relevant to clinical scenarios. In the present study, we used a low dose of 0.5 μg/kg of LPS combined with 800 mg/kg d-GalN as previously reported (Aniya et al., Citation2005). This regimen induces changes in the permeability of the plasma membrane and leakage of liver enzymes from the cells (Mitra et al., Citation2000). Pre-treatment with C. myrrha extract (at the two tested doses of 250 and 500 mg/kg) attenuated the liver injury caused by d-GalN/LPS administration. Recovery towards normalization also suggests that C. myrrha extract may bring on parenchymal cell regeneration and/or protect membrane integrity, thereby, decreasing enzyme leakage and hepatocytes function.
The administration of d-GalN/LPS also affects the antioxidant capacity of the body; this is in agreement with Zhou et al. (Citation2008). The current results also demonstrate that C. myrrha increases hepatic antioxidant activity and decreases the oxidative stress, which are evident by reduced TBARS concentration and enhanced GSH, SOD, and CAT levels. GSH is a powerful antioxidant that protects cells from the oxidative injury by scavenging ROS, and a homeostatic decrease in GSH pool can make cells more vulnerable to further damage by toxins (Ballatori et al., Citation2009). In addition, GSH and CAT work together to counteract the oxidation of proteins, lipids, and DNA by removing ROS from the cells (Yuan & Kaplowitz, Citation2009).
TNF-α plays an important role in the pathogenesis of d-GalN/LPS-induced fulminant hepatic failure and it also induces apoptosis in the early stages of liver damage (Mignon et al., Citation1999). In fact, TNF-α acts by caspase-8 and then stimulates caspase-3, which is a downstream cysteine proteinase through multiple apoptotic pathways (Mignon et al., Citation1999). Interestingly, C. myrrha treatment markedly reduced TNF-α mRNA expression, and at the same time, enhanced the level of IL-10. Furthermore, the pathophysiological conditions of the liver involving oxidative stress initiate an up-regulation of HO-1 mRNA expression and increase in products of heme degradation pathway (Ryter & Choi, Citation2009).
Heme degradation leads to production of biliverdin/bilirubin and carbon monoxide, which are the key mediators of inducible HO-1-mediated cytoprotection, for the reason that they help to restore intracellular homeostatic balance under oxidative stress conditions and suppressing inflammation through down-regulation of pro-inflammatory mediators (Lekic et al., Citation2013; Ryter & Choi, Citation2009). The results of HO-1 gene expression data showed that d-GalN/LPS increases HO-1 level, while pretreatment with C. myrrha d-GalN/LPS rats exhibited a marked reduction in HO-1 mRNA expression. This clearly indicates that C. myrrha has a cytoprotective role as well.
As for the second enzyme of interest, iNOS-2, its mRNA expression, and nitrite levels were significantly increased following d-GalN/LPS administration, which can be attributed to the reported LPS-induced NO production (Chakravortty et al., Citation2001). Similarly, C. myrrha pretreatment had a significant reducing effect on iNOS-2 and nitrate levels in septic rats. Furthermore, it has been reported that C. myrrha mediates its cytoprotective actions by suppressing NO production due to induction of HO-1 (Chen et al., Citation2005; Zhang et al., Citation2011). The GC-MS analysis that was carried out in our study indicated the presence of curezerine, delta-elemene, beta-elemene, 3-[(E)-2-phenyl-1-propenyl] cyc, bicycle [3.1.1]hept-2-ene-2-car, 8-isopropenyl-9-isopropyltetra, and 2,4-bis(3-methyl-1-pentynyl)-4, which were reported to have antioxidant, cytoprotective as well as anticancer properties (Fraternale et al., Citation2011; Marcotullio et al., Citation2011; Racine & Auffray, Citation2005).
Liver histological changes to the inflammatory response such as fatty changes, focal central vein congestion, vocalization, necrosis, and loss of cellular boundaries were visible throughout many lobules in the d-GalN/LPS-administered group. The condensation of Kupffer cells can be seen in C. myrrha pretreated (250 and 500 mg/kg; p.o.) rats may be due to apoptosis (Kim et al., Citation2008). There was a marked attenuation of these inflammatory alterations in a dose-dependent manner in C. myrrha pretreated rat. This clearly indicates a rapid reversal and/or protection against d-GalN/LPS induces hepatotoxicity ().
Conclusion
In conclusion, the present study demonstrates that C. myrrha significantly reduces the oxidative stress of d-GalN/LPS-induced hepatic injury via multiple pathways including a down-regulation of important key players such as TNF-α, IL-6, IL-10, iNOS-2, and HO-1, which might be sufficient to combat cellular damage. Histological recovery towards normalization also suggests that C. myrrha extract may protect parenchymal cells and improve liver tissue regeneration.
Declaration of interest
The authors have confirmed that there is no conflict of interest. The author would like to extend their sincere appreciation to the Deanship of Scientific Research at King Saud University for its funding through research group number (RG# 1435-017).
References
- Aebi H. (1974). Catalase. In: Bergmeyer HV, ed. Methods in Enzymatic Analysis, vol. 2. Cheime, Weinheim, FRG, New York: Academic Press, 674–84
- Al-Howiriny TA, Al-Sohaibani MO, Al-Said MS, et al. (2004). Hepatoprotective properties of Commiphora opobalsamum (“Balessan”), a traditional medicinal plant of Saudi Arabia. Drugs Exp Clin Res 30:213–20
- Aniya Y, Koyama T, Miyagi C, et al. (2005). Free radical scavenging and hepatoprotective actions of the medicinal herb, Crassocephalum crepidioides from the Okinawa Islands. Biol Pharm Bull 28:19–23
- Ballatori N, Krance SM, Notenboom S, et al. (2009). Glutathione dysregulation and the etiology and progression of human diseases. Biol Chem 390:191–14
- Cassatella MA, Meda L, Bonora S, et al. (1993). Interleukin 10 (IL-10) inhibits the release of proinflammatory cytokines from human polymorphonuclear leukocytes. Evidence for an autocrine role of tumor necrosis factor and IL-1 beta in mediating the production of IL-8 triggered by lipopolysaccharide. J Exp Med 178:2207–11
- Chakravortty D, Kato Y, Sugiyama T, et al. (2001). Inhibition of caspase 3 abrogates lipopolysaccharide-induced nitric oxide production by preventing activation of NF-kappaB and c-Jun NH2-terminal kinase/stress-activated protein kinase in RAW 264.7 murine macrophage cells. Infect Immun 69:1315–21
- Chang TS, Lo SK, Shyr HY, et al. (2005). Hepatitis C virus infection facilitates gallstone formation. J Gastroenterol Hepatol 20:1416–21
- Chen JC, Ho FM, Pei-Dawn Lee C, et al. (2005). Inhibition of iNOS gene expression by quercetin is mediated by the inhibition of IkappaB kinase, nuclear factor-kappa B and STAT1, and depends on heme oxygenase-1 induction in mouse BV-2 microglia. Eur J Pharmacol 521:9–20
- Diesen DL, Kuo PC. (2010). Nitric oxide and redox regulation in the liver: Part I. General considerations and redox biology in hepatitis. J Surg Res 162:95–109
- Eskandari MK, Kalff JC, Billiar TR, et al. (1999). LPS-induced muscularis macrophage nitric oxide suppresses rat jejunal circular muscle activity. Am J Physiol 277:G478–86
- Farombi EO, Surh YJ. (2006). Heme oxygenase-1 as a potential therapeutic target for hepatoprotection. J Biochem Mol Biol 39:479–91
- Feng B, Wu S, Lv S, Liu F, et al. (2007). Metabolic profiling analysis of a d-galactosamine/lipopolysaccharide-induced mouse model of fulminant hepatic failure. J Proteome Res 6:2161–7
- Fraternale D, Sosa S, Ricci D, et al. (2011). Anti-inflammatory, antioxidant and antifungal furanosesquiterpenoids isolated from Commiphora erythraea (Ehrenb.) Engl. resin. Fitoterapia 82:654–61
- Gowri Shankar NL, Manavalan R, Venkappayya D, David Raj C. (2008). Hepatoprotective and antioxidant effects of Commiphora berryi (Arn) Engl bark extract against CCl(4)-induced oxidative damage in rats. Food Chem Toxicol 46:3182–5
- Green LC, Wagner DA, Glogowski J, et al. (1982). Analysis of nitrate, nitrite, and [15N]nitrate in biological fluids. Anal Biochem 126:131–8
- Grilli A, De Lutiis MA, Patruno A, et al. (2003). Inducible nitric oxide synthase and heme oxygenase-1 in rat heart: Direct effect of chronic exposure to hypoxia. Ann Clin Lab Sci 33:208–15
- Hamilton TA, Ohmori Y, Tebo J. (2002). Regulation of chemokine expression by antiinflammatory cytokines. Immunol Res 25:229–45
- Han D, Hanawa N, Saberi B, Kaplowitz N. (2006). Hydrogen peroxide and redox modulation sensitize primary mouse hepatocytes to TNF-induced apoptosis. Free Radic Biol Med 41:627–39
- Hong JY, Lebofsky M, Farhood A, Jaeschke H. (2009). Oxidant stress-induced liver injury in vivo: Role of apoptosis, oncotic necrosis, and c-Jun NH2-terminal kinase activation. Am J Physiol Gastrointest Liver Physiol 296:G572–81
- Ibrahim ZS, Ishizuka M, Soliman M, et al. (2008). Protection by Nigella sativa against carbon tetrachloride-induced downregulation of hepatic cytochrome P450 isozymes in rats. Jpn J Vet Res 56:119–28
- Jollow DJ, Mitchell JR, Zampaglione N, Gillette JR. (1974). Bromobenzene-induced liver necrosis. Protective role of glutathione and evidence for 3,4-bromobenzene oxide as the hepatotoxic metabolite. Pharmacology 11:151–69
- Kim SH, Kim YS, Kang SS, et al. (2008). Anti-apoptotic and hepatoprotective effects of gomisin A on fulminant hepatic failure induced by d-galactosamine and lipopolysaccharide in mice. J Pharmacol Sci 106:225–33
- Kono Y. (1978). Generation of superoxide radical during autoxidation of hydroxylamine and an assay for superoxide dismutase. Arch Biochem Biophys 186:189–95
- Lekic N, Canova NK, Horinek A, Farghali H. (2013). The involvement of heme oxygenase 1 but not nitric oxide synthase 2 in a hepatoprotective action of quercetin in lipopolysaccharide-induced hepatotoxicity of d-galactosamine sensitized rats. Fitoterapia 87:20–6
- Maayah ZH, Ansari MA, El Gendy MA, et al. (2014). Development of cardiac hypertrophy by sunitinib in vivo and in vitro rat cardiomyocytes is influenced by the aryl hydrocarbon receptor signaling pathway. Arch Toxicol 88:725–38
- Marcotullio MC, Messina F, Curini M, et al. (2011). Protective effects of Commiphora erythraea resin constituents against cellular oxidative damage. Molecules 16:10357–69
- Mari M, Morales A, Colell A, Garcia-Ruiz C, Fernandez-Checa JC. (2009). Mitochondrial glutathione, a key survival antioxidant. Antioxid Redox Signal 11:2685–700
- Matsumaru K, Ji C, Kaplowitz N. (2003). Mechanisms for sensitization to TNF-induced apoptosis by acute glutathione depletion in murine hepatocytes. Hepatology 37:1425–34
- Mignon A, Rouquet N, Fabre M, et al. (1999). LPS challenge in d-galactosamine-sensitized mice accounts for caspase-dependent fulminant hepatitis, not for septic shock. Am J Respir Crit Care Med 159:1308–15
- Miller AG, Thomas AC, Nyberg JA. (1996). Flora of the Arabian Peninsula and Socotra, vol. 1. Westport, CT: Greenwood Press
- Mitra SK, Seshadri SJ, Venkataranganna MV, et al. (2000). Effect of HD-03 – A herbal formulation in galactosamine-induced hepatopathy in rats. Indian J Physiol Pharmacol 44:82–6
- Motobu M, Amer S, Koyama Y, et al. (2006). Protective effects of sugar cane extract on endotoxic shock in mice. Phytother Res 20:359–63
- Nowak M, Gaines GC, Rosenberg J, et al. (2000). LPS-induced liver injury in d-galactosamine-sensitized mice requires secreted TNF-alpha and the TNF-p55 receptor. Am J Physiol Regul Integr Comp Physiol 278:R1202–9
- Pfaffl MW, Horgan GW, Dempfle L. (2002). Relative expression software tool (REST) for group-wise comparison and statistical analysis of relative expression results in real-time PCR. Nucleic Acids Res 30:e36
- Racine P, Auffray B. (2005). Quenching of singlet molecular oxygen by Commiphora myrrha extracts and menthofuran. Fitoterapia 76:316–23
- Ryter SW, Choi AM. (2009). Heme oxygenase-1/carbon monoxide: From metabolism to molecular therapy. Am J Respir Cell Mol Biol 41:251–60
- Salazar-Montes A, Delgado-Rizo V, Armendáriz-Borunda J. (2000). Differential gene expression of pro-inflammatory and anti-inflammatory cytokines in acute and chronic liver injury. Hepatol Res 16:181–94
- Siegling A, Lehmann M, Platzer C, et al. (1994). A novel multispecific competitor fragment for quantitative PCR analysis of cytokine gene expression in rats. J Immunol Methods 177:23–8
- Stachlewitz RF, Seabra V, Bradford B, et al. (1999). Glycine and uridine prevent d-galactosamine hepatotoxicity in the rat: Role of Kupffer cells. Hepatology 29:737–45
- Stickel F, Schuppan D. (2007). Herbal medicine in the treatment of liver diseases. Dig Liver Dis 39:293–304
- Su SL, Duan JA, Tang YP, et al. (2009). Isolation and biological activities of neomyrrhaol and other terpenes from the resin of Commiphora myrrha. Planta Med 75:351–5
- Takemura S, Minamiyama Y, Imaoka S, et al. (1999). Hepatic cytochrome P450 is directly inactivated by nitric oxide, not by inflammatory cytokines, in the early phase of endotoxemia. J Hepatol 30:1035–44
- Wills ED. (1966). Mechanisms of lipid peroxide formation in animal tissues. Biochem J 99:667–76
- Yoshinari O, Shiojima Y, Igarashi K. (2014). Hepatoprotective effect of germanium-containing Spirulina in rats with d-galactosamine- and lipopolysaccharide-induced hepatitis. Br J Nutr 111:135–40
- Yuan L, Kaplowitz N. (2009). Glutathione in liver diseases and hepatotoxicity. Mol Aspects Med 30:29–41
- Zhang ZJ, Cheang LC, Wang MW, Lee SM. (2011). Quercetin exerts a neuroprotective effect through inhibition of the iNOS/NO system and pro-inflammation gene expression in PC12 cells and in zebrafish. Int J Mol Med 27:195–203
- Zhou Y, Park CM, Cho CW, Song YS. (2008). Protective effect of pinitol against d-galactosamine-induced hepatotoxicity in rats fed on a high-fat diet. Biosci Biotechnol Biochem 72:1657–66