Abstract
Context: Hepatic ischemia/reperfusion injury (IRI) is a major cause of liver damage during liver surgery and transplantation. Plants have historically been used in treating liver damage, and Hammada scoparia (Pomel) (Chenopodiaceae) has been reported to possess a broad spectrum of pharmacological and therapeutic activities.
Objective: In this study, a flavonoid-enriched fraction was used before the warm ischemia (WI) process as pharmacological preconditioning and in combination with technical postconditioning to evaluate their protective effects.
Materials and methods: The rats were divided into five groups: a sham group; a control group (Control-IR) that was submitted to 60 min WI; a Pharmacological Preconditioning group (PreC-IR) that received flavonoid-enriched fraction (200 mg/kg body weight); a Postconditioning group (PostC) and a PreC + PostC group.
Results: The use of the flavonoid-enriched fraction was noted to significantly (p < 0.05) reduce liver injury, as evidenced by the decrease in liver transaminase activities (AST and ALT) and lactic dehydrogenase (LDH), alkaline phosphatase (ALP), and lipid peroxidation (TBARS), levels as well as the enhancement of antioxidant enzymes (catalase (CAT), superoxide dismutase (SOD), and glutathione peroxidase (GPx)) responses. The results also indicated that, compared with the separate application of pharmacological preconditioning and postconditioning, the combination of both treatments was more effective in reducing tissue oxidative stress levels through modulating SOD, GSH-PX, and CAT activities. Furthermore, the combined protocol further decreased the liver morphological score compared with solo treatment.
Discussion and conclusion: Overall, the results indicate that the H. scoparia flavonoid-enriched fraction could be a promising candidate for future application as a pharmacological preconditioning agent against hepatic IRI.
Introduction
Hepatic ischemia/reperfusion injury (IRI) is a one of the major complications in clinical hepatic surgeries, including liver transplantation and hepatic resection procedures (DeRougemont et al., Citation2009; Jaeschke, Citation2003a). Ischemia/reperfusion injury is a major cause of liver damage, which can be mediated by diverse and complex cellular and biochemical processes, such as the deregulation of the healthy phenotype of all liver cellular components (Peralta et al., Citation2013). Several signaling events can contribute to hepatocellular damage, including the interaction between hepatocytes, liver sinusoidal endothelial cells (LSEC), Kupffer cells (KC), hepatic stellate cells (HSC), infiltrating neutrophils, macrophages, and platelets (Jaeschke, Citation2003b). Both pro-inflammatory cytokines and reactive oxygen species (ROS) are key mediators of hepatic IRI (Penna et al., Citation2009; Zhou et al., Citation2009). ROS generated by Kupffer cells and hepatocytes can lead to direct damage on endothelial cells (ECs) and hepatocytes.
The pathogenesis of hepatic IR injury has attracted increasing attention in recent research, and hepatic IR injury has been described to follow a two-phase time course consisting of an initial and a late phase (Glantzounis et al., Citation2005). The initial phase of injury (within the first 2 h after reperfusion) is characterized by oxidative stress, where the production and the release of ROS directly result in hepatocellular injury (He et al., Citation2006; Wu et al., Citation2009). The late phase, which lasts between 6 and 48 h after hepatic reperfusion, is an inflammatory disorder mediated by recruited neutrophils (Jaeschke & Lemasters, Citation2003; Vardanian et al., Citation2008). The restoration of the blood flow triggers a chain of negative consequences, such as oxygen-free radical generation. This includes the reaction of superoxide and hydroxyl radicals with unsaturated lipids of biomembranes, resulting in the generation of lipid peroxide radicals and fragmentation products, such as malondialdehyde (MDA) (Cano et al., Citation2003).
The balance of redox is pivotal for the normal function and integrity of tissues. Ischemic injury occurs due to a variety of conditions, leading to the accumulation of reactive oxygen species (ROS) and imbalances in redox levels in the tissues (Wu et al., Citation2009). The major regulators are ROS scavengers, which include superoxide dismutases (SODs), catalase (CAT), and glutathione peroxidase (GSH-Px). ROS scavengers are responsible for reducing ROS inside the tissues (Song et al., Citation2012). When the injury is pronounced or persistent, compensatory responses become inadequate to correct the imbalanced redox status, giving rise to oxidant stress, which, with the activation of subsequent signaling events, leads to inflammatory responses and tissue damage. Several studies have recently postulated that redox imbalances can be corrected by treatments with antioxidants, free radical scavengers, or their mimetics (Wu et al., Citation2009).
Several procedures have been proposed to improve liver tolerance of reperfusion damage (DeRougemont et al., Citation2009; Selzner et al., Citation2003). Among these, the ischemic preconditioning (DeRougemont et al., Citation2009; Peralta et al., Citation2003; Zhang et al., Citation2009) and ischemic postconditioning of the liver by intermittent interruptions of blood flow in the early phase of reperfusion have been reported to improve post-transplant liver function in rat models (Wang et al., Citation2008; Zhang et al., Citation2009) and to induce tissue-protective effects after ischemia and reperfusion injury.
The use of antioxidants to reduce oxidant injury during the ischemia and reperfusion possess has been reported to offer promising opportunities for improving hepatic recovery from dysfunction (Song et al., Citation2012). Of particular interest, Hammada scoparia is a medicinal plant known to exhibit a broad spectrum of biological, pharmacological, and therapeutic activities (Bourogaa et al., Citation2011, Citation2013; ElRhaffari et al., Citation2002; Mezghani-Jarraya et al., Citation2009). Hammada scoparia (Pomel) Iljin (Chenopodiaceae) is locally known in Tunisia as “Rimth”. Several in vivo studies reported that polar extracts of H. scoparia leaves has potent antioxidative activity and hepatoprotective effects against ethanol-induced liver injury in male rats (Bourogaa et al., Citation2013, Citation2014).
Despite the experimental evidence in support for the promising protective effects of H. scoparia flavonoids, little work is currently available on its potential effects against hepatic IRI. Accordingly, the present study was undertaken to investigate and evaluate the potential anti-IRI effects of a flavonoid-enriched fraction derived from H. scoparia leaves used as a separate pharmacological ischemic preconditioning or in combination with postconditioning protocols.
Materials and methods
Hammada scoparia extract
The flavonoid-enriched fraction used in the present study was extracted as described by Bourogaa et al. (Citation2011). Briefly, dried leaves of H. scoparia were extracted by incubation with H2O or EtOH/H2O (1–9 v/v) under stirring for 24 h, and extracts were then dried by lyophilization. Different fractions were prepared from the EtOH/H2O extracts using a chromatographical separation over Silicaphase column (Kiesel gel) eluted by a stepwise CHCl3/MeOH gradient. In other cases, the EtOH/H2O extract was mixed with CH2Cl2 (at pH 2 and then at pH 9), and the residue was treated with BuOH. The dried butanolic extract was used for a flavonoid precipitation assay. This assay involved a dissolution in EtOH/ACOET (1–2 v/v), which yielded into the flavonoid fraction. The obtained fraction was then evaporated in a vacuum rotary evaporator, weighed, and kept in the dark at 4 °C until further use.
Animals
Young male Wistar rats weighing 250–270 g were used in this study. The animals were housed in the animal facility of the University of Sfax and treated in accordance with the National Institutes of Health guidelines for the maintenance, care, and treatments of experimental animals. The rats were housed under standard (25 ± 2 °C) laboratory conditions with a 12 h dark and light cycle. They had access to standard rat chow pellets and water ad libitum. The animals were acclimatized to laboratory conditions before the test.
Induction of partial hepatic ischemia
The animals were fasted for 8 h, with free access to water, before IR injury. The segmental (70%) hepatic ischemia model was used in the present study (Nakashima et al., Citation2003; Saidi et al., Citation2015). A midline laparotomy was performed under anesthesia with 10% chloral hydrate (0.3 g/kg, i.p.) in rats. The liver was exposed through a midline incision, and all structures in the portal triad (hepatic artery, portal vein, and bile duct) to the cephalad lobes of the liver were interrupted for 60 min with a vascular clip (Harvard Apparatus, Inc., Hollinston, MA). This method of partial hepatic ischemia prevented mesenteric venous congestion by permitting portal decompression through the right and caudate lobes. The clip was removed after 60 min of ischemia in the left lateral and median lobes, initiating hepatic reperfusion. Sham operation consisted in laparotomy and liver manipulation without vessel clamping.
Ten rats from each group were killed at 60 min, 12 h, and 48 h of reperfusion. Blood samples were collected to determine liver function parameters, and tissue samples were taken from the left lateral hepatic lobe at the end of reperfusion to determine tissue MDA, antioxidants enzymes activities (CAT, SOD, and GPx), and histologic changes. At the end of the ischemic period, the rats were allowed to recover, housed in individual cages, and fed the standard chow diet. Survival rates were assessed every 24 h for 7 d after operation.
Treatment groups
The rats were divided into five groups as follows (the grouping is described in detail in ).
Figure 1. Experimental protocols. Black areas represent periods of hepatic warm ischemia (WI). PostC procedure: 1 min of reperfusion (R) followed by 1 min of reocclusion (WI) for three times at the onset of reperfusion.
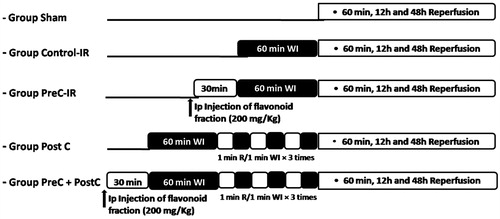
Sham group: this group of rats was submitted to the surgical procedures, except for liver WI/R, and maintained under anesthesia for an equivalent duration.
Control-IR group: the rats belonging to this group were submitted to the surgical procedures and then to 60 min of WI. One hour later, the ischemic liver was reperfused by opening the vascular clamp, and the rats were killed 1 h, 12 h, and 48 h later.
PreC-IR group: the animals from this group received the flavonoid-enriched fraction (200 mg/kg, intraperitoneal) as pharmacological preconditioning 30 min prior to ischemia–reperfusion. They were killed after 1 h, 12 h, and 48 h of reperfusion.
PostC group: the rats forming this group were submitted to three 5-min reperfusion cycles after 60 min of WI, and WI was performed before final reperfusion.
PreC + PostC group: this group of rats received the flavonoid-enriched fraction (200 mg/kg, intraperitoneal) 30 min prior to the surgical procedures and after 60 min WI, three cycles of 5 min reperfusion was performed before final reperfusion.
Hepatocellular injury assay
Aspartase aminotransferase (AST), alanine aminotransferase (ALT), lactic dehydrogenase (LDH), and alkaline phosphatase (ALP) levels in the blood serum were determined as indicators of hepatocyte function using commercial kits supplied by Sigma (Munich, Germany). Blood samples were centrifuged immediately at 8000 × g for 10 min. Serum enzyme levels were measured with a Siemens ADVIA 1800 analyzer (Siemens Healthcare Diagnostic, New York, NY).
Oxidative stress parameters
Oxidative stress analysis was performed by the examination of thiobarbituric acid-reactive substances (TBARS) and SOD, CAT, and GPx activities. Liver tissue (1 g) was homogenized in 2 ml TBS (50 mM Tris, 150 mM NaCl, pH 7.4) in an ultrasound homogenizer and centrifuged at 9000 rpm for 15 min. The supernatants were removed and stored at −80 °C for subsequent analysis.
TBARS assay: As a marker of lipid peroxidation, the TBARS concentration was measured based on the method described by Esterbauer et al. (Citation1991). In brief, 250 μl of the supernatant was mixed with 100 μl TBS buffer and 250 μl of trichloroacetic acid–BHT (20–1%), and then vortexed and centrifuged at 1000 rpm for 10 min. Besides, 400 μl of the collected supernatant were mixed with 80 μl HCl (0.6 M) and 320 μl of Tris-TBA (26–120 mM), and then vortexed and incubated at 80 °C for 10 min. The resulting colored upper layer was measured at 532 nm. The TBARS concentration was calculated by referring to the extinction coefficient of TBARS (1.56 × 105 M−1 cm−1) and expressed per milligram of protein.
SOD: Activity was determined at 25 °C by measuring the ability to inhibit the photoreduction of nitrobluetetrazolium (NBT) to the blue formazan (Durak et al., Citation1993). The assay was performed in an aerobic mixture consisting of PBS (50 mM), methionine (13 mM), EDTA (0.1 mM) riboflavine (2 μM), and NBT (75 μM). The activity was expressed as units/milligram protein, with 1 U being the amount inhibiting the photoreduction of NBT by 50%.
CAT: CAT activity was determined by measuring the decrease in absorbance at 240 nm for 2 min at 25 °C of H2O2 (a final concentration of 20 mM) according to the method of Aebi (Citation1984). CAT activity was expressed as micromoles of H2O2 destroyed/min/milligram protein at 25 °C.
GPx: GPx activity was assayed at 25 °C according to the method of Flohe and Günzler (Citation1984) with some modifications. The activity was measured in 250 μl cellular extract mixed with GSH (a final concentration of 0.35 mM). Reactions were started with the addition of H2O2 (0.2 mM). After the reactions were stopped, 5,5′-dithiobis-2-nitrobenzoic acid was added, and the absorbance was recorded at 412 nm. GPx activity was expressed as micromoles of GSH oxidized/min/g protein.
Morphological assessment of ischemia reperfusion injury
After 48 h of liver perfusion, biopsies were taken, fixed with formaldehyde (6%), paraffin embedded, sectioned (6 μm), and stained with hematoxylin and eosin. Ischemia reperfusion injury was evaluated based on a morphological score used by Liu et al. (Citation2013). The extent and the severity of the following parameters were scored: dilatation of sinusoids, anoxic vacuoles, enlarged space of Disse, loss of cellular cohesion, parenchymal cell loss, neutrophils, and congestion. All biopsies were scored by two experienced microscopists (Bourogaa E. and Saaoudi M.).
Data analysis
The results were expressed as mean ± SD. Statistical comparisons were carried out using one-way analysis of variance ANOVA, followed by the Fisher test (StatView, Cary, NC). Differences between groups were considered significant at p < 0.05.
Results
Animal survival
Five out of nine control animals died within 7 d after reperfusion, resulting in a 55% survival. In contrast, 77% (7/9) of the treated animals survived for 7 d. One animal in the treated group died within 1 d after reperfusion. The treatment improved animal survival.
Serum AST, ALT, LDH, and PAL levels
In the first set of experiments, ALT, AST, LDH, and ALP activities were used as markers to evaluate the effects of various ischemic conditioning strategies against hepatic ischemia and reperfusion injury. Compared with the control group, the plasma activities of ALT, AST, LDH, and ALP significantly decreased in the PreC-IR and PostC-IR groups (p < 0.05). It was hypothesized that combinations of the pharmacological preconditioning and postconditoning procedures would show more effective protective effects against hepatic ischemia and reperfusion injury than the individual treatment. The results showed that the hepatic parameters decreased more obviously in the PreC + PostC group, as shown in . Hence, the combinations of the pharmacological preconditioning and postconditioning procedures provided synergistic protective effects against ischemic reperfusion injury.
Response of antioxidative pathways in the pre- and postconditioning process
Another important factor causing ischemia and reperfusion injury is reactive oxygen species (ROS) mediating cell apoptosis. To investigate the role of ROS in IR injury, the level of malondialdehyde (MDA), which indicates the overall level of the tissue oxidative stress, was measured. As shown in , both the separate or combined use of the PostC and pharmacological PreC protocols could decrease the oxidative stress level indicated by reduced MDA content. To quantify the contribution of different ROS scavengers, the activity of SOD, CAT, and GPx were measured in the liver homogenate after perfusion. The activities of all those enzymes increased in the PreC, PostC, and PreC + PostC groups after reperfusion (p < 0.05) compared with the control group ().
Protective effect on the liver tissue from I/R injury
To further confirm the protective effect of the flavonoid-enriched fraction on hepatic I/R injury, sections of the liver obtained from the ischemic right lobe at 48 h after reperfusion were submitted to histopathological analysis. On one hand, the liver tissues from the control-IR group (), I/R rats showed significant cytoplasmic vacuolization, sinusoidal dilatation, extensive hepatic cellular necrosis, and massive cellular infiltration. The liver tissues of the rats from the PostC-IR and PreC-IR groups of rats were, on the other hand, noted to show almost normal parenchymal appearance and to preserve lobular architecture. The evaluation of the histological features of I/R injury revealed that the PreC + PostC group exhibited significantly lower scores.
Figure 5. Morphological score after 48 h reperfusion for liver injury. Representative H&E staining of a liver from Control-IR showing signs of liver damage: dilatation of sinusoids, anoxic vacuoles, enlarged space of Disse, etc. (original magnification 200 × ). Results presented as mean and Box–Whiskers. *p < 0.05 when compared with the control-IR group.
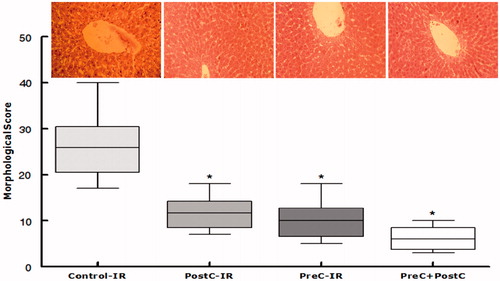
Discussion
The results of the present study showed that H. scoparia flavonoid-enriched fraction, as a type of phenolic compound widely distributed in plants, reduced hepatocellular injury in I/R. The flavonoid-enriched fraction was also noted to attenuate oxidative damage after I/R. Compared with those observed for the Control-IR group, the AST, ALT, LDH and ALP activities recorded for the pharmacological PreC and PostC groups were noted to undergo a significant decrease. The activity of antioxidant enzymes in the PreC and PostC groups was also significantly higher, demonstrating their stronger ability to clear oxygen-free radicals and reduce the degree of lipid peroxidation caused by oxygen free radicals, which was significantly lower than the one recorded in the Control-IR group.
The understanding of the pathogenesis of I/R and identification of potential therapeutic approaches are important steps for the improvement of clinical outcomes. One particular approach is through the inhibition of ROS production. During ischemic reperfusion (I/R) injury, several components of reactive oxygen species (ROS) are involved (Kakkar et al., Citation2013). The initial hepatic I/R injury is known to be triggered by ROS with inflammation involving chemokines and cytokines, followed by neutrophil-mediated hepatic injury occurring in the late period of reperfusion (Jaeschke, Citation2006). Recent evidence has shown that ROS can also induce apoptosis, which is a mechanism for cell death following the reperfusion of the ischemic liver (Yu et al., Citation2011). Endogenous antioxidant levels were previously reported to decrease significantly during reperfusion (Marubayashi et al., Citation1987). Therefore, the administration of exogenous antioxidants, particularly from natural origins, could possibly decrease the severity of I/R damage (Kim et al., Citation2013). Hammada scoparia leaves have previously been reported to exhibit a wide range of attractive bioactivities, including antibacterial and antileishmaniasis (ElRhaffari et al., Citation2002). Several studies have also shown that H. scoparia leaves extracts exhibit molluscicidal activity characteristic to principal alkaloids against Galba truncatula (Mezghani-Jarraya et al., Citation2009) and potent antitumoral activity. The most important aspect of H. scoparia relates to its apoptosis effect in adherent leukemic cells but not normal hematopoietic cells (Bourogaa et al., Citation2011).
The results presented in the current study showed that the liver tissues of rats challenged with I/R underwent a significant increase in lipid peroxidation (MDA), which coincided with a rapid decrease in the antioxidant enzyme activities as compared with the sham group. During reperfusion, the mitochondrial ROS generation increases dramatically (Harada et al., Citation2004; He et al., Citation2006) since the electrons released by the respiratory chain can be directly donated to the newly supplied oxygen (Takemura et al., Citation1992). MDA is an end-product of lipid peroxidation, and its content is used to estimate the degree of oxidative damage (Schuessel et al., Citation2005). Moreover, CAT, SOD, and GPx play important roles as free radical scavengers to counteract the deleterious effect of ROS. Therefore, antioxidant enzyme activities and MDA content are key indicators of antioxidant capacity. In fact, SOD was reported to convert superoxide radicals to hydrogen peroxide, which is subsequently converted to water by CAT and GSH-Px (Wang et al., Citation2007). Dysfunctions of CAT, SOD, and GPx may result in the loss of the protective activity exerted by those enzymes, which may explain the reduction observed in the CAT, SOD, and GPx levels after reperfusion in the ischemic groups investigated in the present work. If this was the case, then an antioxidant treatment should have prevented the loss of antioxidants activities by effectively scavenging the excess of ROS. The results indicated that the hepatoprotection by the flavonoid-enriched fraction was due to its antioxidant properties through enhancing the activity of SOD, GPx, and CAT (). As a type of phenolic compound, flavonoids have attracted increasing attention particularly for their attractive antioxidant activities (Awaad et al., Citation2012; Prommuak et al., Citation2008). They have also been reported to offer a promising source of potential antioxidants (Wang et al., Citation2014). Widely distributed in plants, these compounds have the ability to scavenge free radicals, superoxides, and hydroxyl radicals through single-electron transfer (Havsteen, Citation2002; Le et al., Citation2007; Lemanska et al., Citation2001).
The analysis of the flavonoid-enriched extract from H. scoparia by LC-UV-MS/MS indicated the presence of isorhamnetin–xylose–galactose, quercetin–xylose–rhamnose–galactose, and quercetin–glucose–rhamnose (rutin) (Bourogaa et al., Citation2011). However, the most potent compound was rutin (Bourogaa et al., Citation2011). The latter is a powerful antioxidant and anti-inflammatory polyphenol (Chen et al., Citation2000). It was suggested that the reduction of ROS production of rutin is related to the enhancement of (SOD, GPx, or CAT activity as well as of its inhibitory activity on xanthine oxidase, which is an important enzyme in the oxidative injury of tissues (Khan et al., Citation2009; Wang et al., Citation2012). Furthermore, rutin and its analogues, such as epigallocatechin-3-gallate (EGCG) and quercetin, act as efficient radical inhibitors. They have also been reported to rescue spatial memory impairment in rats with ischemia–reperfusion-induced cerebral injury (Pu et al., Citation2007). Rutin was also noted to decrease mitochondrial TBARS (Gupta et al., Citation2003) and to attenuate morphological damage and ischemic apoptosis (Khan et al., Citation2009).
Although the topic of hepatic I/R injury has been studied extensively in recent decades, the molecular mechanisms underlying this effect largely remain poorly understood. Interestingly, however, the pharmaceutical inhibition of glycogen synthase kinase (GSK)-3β was previously reported to protect the liver from I/R-induced injury (Moreno et al., Citation2007; Varela et al., Citation2010). Given the fact that the rutin found in the flavonoid-enriched fraction regulates the phosphorylation of GSK-3β (Bourogaa et al., Citation2011), this compound can presumably play a key role in GSK-3β signaling and tissue function regulation during hepatic I/R injury.
During reperfusion, the influx of a large amount of O2 is known to induce an increased electron leak in the mitochondrial respiratory electron transport chain and a neutrophil respiratory burst. These two processes lead to the generation of oxygen free radicals (Arab et al., Citation2009; Nishikawa et al., Citation2009; Ozmen et al., Citation2009). Postconditioning provides the liver with several cycles of on/off flow before reperfusion to control the generation of oxygen free radicals. This method, which is equivalent to the slow and intermittent reoxygenation in controls, consequently decreased the burst of oxygen free radicals when the liver cell was suddenly reoxygenated, thus activating the intracellular antioxidant enzymes (CAT, SOD, and GPx) and free radical scavengers to exert protective effects (Peralta et al., Citation2003; Wu et al., Citation2007). The results of the present study suggested that both pharmacological preconditioning and postconditioning reduce the liver damage marked by decreased AST, ALT, LDH, and ALP activities. The protective effects against hepatic IRI could be attributed to the role that those combinations played in reducing the level of tissue oxidative stress. The combination between the pharmacological preconditioning and postconditoning procedures could also be hypothesized to show more effective protection effects against hepatic ischemia and reperfusion injury than individual treatments. The use of ischemic preconditioning in combination with ischemic postconditioning could offer additive protection by increasing the GPx, SOD, and CAT activities and reducing the level of lipid peroxidation.
Taken together, the results of the present study demonstrate that the application of H. scoparia flavonoid as a pharmacological precondiotning procedure reduce hepatocellular injuries after I/R. The use of the flavonoidic fraction in our study showed that flavonoid could further improve the oxidation resistance. More interestingly, the combined application of pharmacological preconditioning and postconditioning could provide synergistic protection against liver ischemic reperfusion injury.
Acknowledgements
The authors would like to express their sincere gratitude to Mr. Anouar Smaoui from the English Language Unit at the Faculty of Science of Sfax for his constructive proofreading and language polishing services.
Declaration of interest
The authors report that they have no conflicts of interest. This research study did not receive any specific grant from any funding agency in the public, commercial or not-for-profit sectors.
References
- Aebi H. (1984). Catalase in vitro. Methods Enzymol 105:121–6
- Arab HA, Sasani F, Rafiee MH, et al. (2009). Histological and biochemical alterations in early-stage lobar ischemia-reperfusion in rat liver. World J Gastroenterol 15:1951–7
- Awaad AS, Maitland DJ, Donia AELR, et al. (2012). Novel flavonoids with antioxidant activity from a Chenopodiaceous plant. Pharm Biol 50:99–104
- Bourogaa E, Bertrand J, Despeaux M, et al. (2011). Hammada scoparia flavonoids and rutin kill adherent and chemoresistant leukemic cells. Leuk Res 35:1093–101
- Bourogaa E, Jarraya RM, Nciri R, et al. (2014). Protective effects of aqueous extract of Hammada scoparia against hepatotoxicity induced by ethanol in the rat. Toxicol Ind Health 30:113–22
- Bourogaa E, Nciri R, Mezghani-Jarraya R, et al. (2013). Antioxidant activity and hepatoprotective potential of Hammada scoparia against ethanol-induced liver injury in rats. J Physiol Biochem 69:227–37
- Cano CP, Bermudez VP, Atencio HE, et al. (2003). Increased serum malondialdehyde and decreased nitric oxide within 24 hours of thrombotic stroke onset. Am J Ther 10:473–6
- Chen S, Gong J, Liu F, Mohammed U. (2000). Naturally occurring polyphenolic antioxidants modulate IgE-mediated mast cell activation. Immunology 100:471–80
- DeRougemont O, Lehmann K, Clavien PA. (2009). Preconditioning, organ preservation, and postconditioning to prevent ischemia-reperfusion injury to the liver. Liver Transpl 15:1172–82
- Durak I, Yurtarslanl Z, Canbolat O, Akyol O. (1993). A methodological approach to superoxide dismutase (SOD) activity assay based on inhibition of nitro blue tetrazolium (NBT) reduction. Clin Chim Acta 214:103–4
- ElRhaffari L, Hammani K, Benlyas M, Zaid A. (2002). Traitement de la leishmaniose cutanée par la phytothérapie au Tafilalet. Biol Santé 1:45–54
- Esterbauer H, Schaur RJ, Zollner H. (1991). Chemistry and biochemistry of 4-hydroxynonenal, malonaldehyde and related aldehydes. Free Radic Biol Med 11:81–128
- Flohe L, Gunzler WA. (1984). Assays of glutathione peroxidase. Methods Enzymol 105:114–21
- Glantzounis GK, Salacinski HJ, Yang W, et al. (2005). The contemporary role of antioxidant therapy in attenuating liver ischemia-reperfusion injury: A review. Liver Transpl 11:1031–47
- Gupta R, Singh M, Sharma A. (2003). Neuroprotective effect of antioxidants on ischaemia and reperfusion-induced cerebral injury. Pharmacol Res 48:209–15
- Harada H, Hines IN, Flores S, et al. (2004). Role of NADPH oxidase-derived superoxide in reduced size liver ischemia and reperfusion injury. Arch Biochem Biophys 423:103–8
- Havsteen BH. (2002). The biochemistry and medical significance of the flavonoids. Pharmacol Ther 96:67–202
- He SQ, Zhang YH, Venugopal SK, et al. (2006). Delivery of antioxidative enzyme genes protects against ischemia/reperfusion-induced liver injury in mice. Liver Transpl 12:1869–79
- Jaeschke H. (2003a). Molecular mechanisms of hepatic ischemia-reperfusion injury and preconditioning. Am J Physiol Gastrointest Liver Physiol 284:G15–26
- Jaeschke H. (2003b). Role of reactive oxygen species in hepatic ischemia–reperfusion injury and preconditioning. J Invest Surg 16:127–40
- Jaeschke H. (2006). Mechanisms of liver injury. II. Mechanisms of neutrophil-induced liver cell injury during hepatic ischemia-reperfusion and other acute inflammatory conditions. Am J Physiol Gastrointest Liver Physiol 290:G1083–8
- Jaeschke H, Lemasters JJ. (2003). Apoptosis versus oncotic necrosis in hepatic ischemia/reperfusion injury. Gastroenterology 125:1246–57
- Kakkar V, Muppu SK, Chopra K, Kaur IP. (2013). Curcumin loaded solid lipid nanoparticles: An efficient formulation approach for cerebral ischemic reperfusion injury in rats. Eur J Pharm Biopharm 85:339–45
- Khan MM, Ahmad A, Ishrat T, et al. (2009). Rutin protects the neural damage induced by transient focal ischemia in rats. Brain Res 1292:123–35
- Kim J, Kim HY, Lee SM. (2013). Protective effects of geniposide and genipin against hepatic ischemia/reperfusion injury in mice. Biomol Ther (Seoul) 21:132–7
- Le K, Chiu F, Ng K. (2007). Identification and quantification of antioxidants in fructus lycii. Food Chem 105:353–63
- Lemanska K, Szymusiak H, Tyrakowska B, et al. (2001). The influence of pH on antioxidant properties and the mechanism of antioxidant action of hydroxyflavones. Free Radic Biol Med 31:869–81
- Liu Q, Vekemans K, Iania L, et al. (2013). Assessing warm ischemic injury of pig livers at hypothermic machine perfusion. J Surg Res 186:379–89
- Marubayashi S, Dohi K, Ochi K, Kawasaki T. (1987). Protective effects of free radical scavenger and antioxidant administration on ischemic liver cell injury. Transplant Proc 19:1327–8
- Mezghani-Jarraya R, Hammami H, Ayadi A, Damak M. (2009). Molluscicidal activity of Hammada scoparia (Pomel) Iljin leaf extracts and the principal alkaloids isolated from them against Galba truncatula. MemInst Oswaldo Cruz 104:1035–8
- Moreno AJ, Oliveira PJ, Nova CD, et al. (2007). Unaltered hepatic oxidative phosphorylation and mitochondrial permeability transition in Wistar rats treated with nimesulide: Relevance for nimesulide toxicity characterization. J Biochem Mol Toxicol 21:53–61
- Nakashima Y, Kohno H, On ELA, et al. (2003). Sequential changes of connexin32 and connexin26 in ischemia-reperfusion of the liver in rats. Hepatol Res 27:67–75
- Nishikawa M, Hashida M, Takakura Y. (2009). Catalase delivery for inhibiting ROS-mediated tissue injury and tumor metastasis. Adv Drug Deliv Rev 61:319–26
- Ozmen MM, Zulfikaroglu B, Col C, et al. (2009). Effect of increased abdominal pressure on cytokines (IL1 beta, IL6, TNFalpha), C-reactive protein (CRP), free radicals (NO, MDA), and histology. Surg Laparosc Endosc Percutan Tech 19:142–7
- Penna C, Mancardi D, Rastaldo R, Pagliaro P. (2009). Cardioprotection: A radical view free radicals in pre and postconditioning. Biochim Biophys Acta 1787:781–93
- Peralta C, Jimenez-Castro MB, Gracia-Sancho J. (2013). Hepatic ischemia and reperfusion injury: Effects on the liver sinusoidal milieu. J Hepatol 59:1094–106
- Peralta C, Serafin A, Fernandez-Zabalegui L, et al. (2003). Liver ischemic preconditioning: A new strategy for the prevention of ischemia-reperfusion injury. Transplant Proc 35:1800–2
- Prommuak C, De-Eknamkul W, Shotipruk A. (2008). Extraction of flavonoids and carotenoids from Thai silk waste and antioxidant activity of extracts. Sep Purif Technol 62:444–8
- Pu F, Mishima K, Irie K, et al. (2007). Neuroprotective effects of quercetin and rutin on spatial memory impairment in an 8-arm radial maze task and neuronal death induced by repeated cerebral ischemia in rats. J Pharmacol Sci 104:329–34
- Saidi S, Abdelkafi S, Jbahi S, et al. (2015). Temporal changes in hepatic antioxidant enzyme activities after ischemia and reperfusion in a rat liver ischemia model: Effect of dietary fish oil. Hum Exp Toxicol 34:249–59
- Schuessel K, Schafer S, Bayer TA, et al. (2005). Impaired Cu/Zn-SOD activity contributes to increased oxidative damage in APP transgenic mice. Neurobiol Dis 18:89–99
- Selzner N, Rudiger H, Graf R, Clavien PA. (2003). Protective strategies against ischemic injury of the liver. Gastroenterology 125:917–36
- Song X, Xu H, Feng Y, et al. (2012). Protective effect of grape seed proanthocyanidins against liver ischemic reperfusion injury: Particularly in diet-induced obese mice. Int J Biol Sci 8:1345–62
- Takemura G, Onodera T, Ashraf M. (1992). Hydroxyl radical production during early reperfusion after different periods of ischemia in rat hearts and its effect on myocardial function. OH in postischemic heart. Am J Cardiovasc Pathol 4:165–74
- Vardanian AJ, BusuttiL RW, Kupiec-Weglinski JW. (2008). Molecular mediators of liver ischemia and reperfusion injury: A brief review. Mol Med 14:337–45
- Varela AT, Simoes AM, Teodoro JS, et al. (2010). Indirubin-3′-oxime prevents hepatic I/R damage by inhibiting GSK-3beta and mitochondrial permeability transition. Mitochondrion 10:456–63
- Wang B, Peng L, Zhu L, Ren P. (2007). Protective effect of total flavonoids from Spirodela polyrrhiza (L.) Schleid on human umbilical vein endothelial cell damage induced by hydrogen peroxide. Colloids Surf B Biointerfaces 60:36–40
- Wang KX, Hu SY, Jiang XS, et al. (2008). Protective effects of ischaemic postconditioning on warm/cold ischaemic reperfusion injury in rat liver: A comparative study with ischaemic preconditioning. Chin Med J (Engl) 121:2004–9
- Wang SW, Wang YJ, Su YJ, et al. (2012). Rutin inhibits beta-amyloid aggregation and cytotoxicity, attenuates oxidative stress, and decreases the production of nitric oxide and proinflammatory cytokines. Neurotoxicology 33:482–90
- Wang SW, Wang YJ, Su YJ, et al. (2014). Rutin inhibits beta-amyloid aggregation and cytotoxicity, attenuates oxidative stress, and decreases the production of nitric oxide and proinflammatory cytokines. Neurotoxicology 33:482–90
- Wu BQ, Chu WW, Zhang LY, et al. (2007). Protection of preconditioning, postconditioning and combined therapy against hepatic ischemia/reperfusion injury. Chin J Traumatol 10:223–7
- Wu J, Hecker JG, Chiamvimonvat N. (2009). Antioxidant enzyme gene transfer for ischemic diseases. Adv Drug Deliv Rev 61:351–63
- Yu HC, Qin HY, He F, et al. (2011). Canonical notch pathway protects hepatocytes from ischemia/reperfusion injury in mice by repressing reactive oxygen species production through JAK2/STAT3 signaling. Hepatology 54:979–88
- Zhang WX, Yin W, Zhang L, et al. (2009). Preconditioning and postconditioning reduce hepatic ischemia-reperfusion injury in rats. Hepatobiliary Pancreat Dis Int 8:586–90
- Zhou G, Dada LA, Wu M, et al. (2009). Hypoxia-induced alveolar epithelial-mesenchymal transition requires mitochondrial ROS and hypoxia-inducible factor 1. Am J Physiol Lung Cell Mol Physiol 297:L1120–30