Abstract
Context: Dracocephalum polychaetum Bornm. (Lamiaceae) is used in folk medicine and contains antioxidant agents.
Objectives: The objective of this study is to investigate the antidiabetic, antioxidant, and antilipid peroxidative properties of methanol extract of D. polychaetum aerial parts.
Materials and methods: The effect of extract (200, 300, and 400 mg/kg, b.w.) on oral glucose tolerance test (OGTT) was investigated. Also, extract (300 mg/kg) administered orally in diabetic rats for 14 d then, serum levels of some biochemical factors were evaluated. Pancreas samples were used for the determination of malondealdehyde (MDA) level, glutathione (GSH) content, superoxide dismutase, and catalase enzyme activities. Red blood cells (RBCs) and plasma were used for MDA estimation. Pancreatic α-amylase inhibition, DPPH (1,1-diphenyl-2-picrylhydrazyl), and FRAP (ferric reducing antioxidant power) assays were done. The total flavonoid content of the extract was determined by spectrophotometry.
Results: Extract (300 mg/kg) decreased serum glucose level (27.1%) significantly at 120 min in OGTT. Serum levels of creatinine, triglycerides, cholesterol, alanine amino transferase and MDA levels in plasma, RBCs, and pancreas significantly decreased in treated (300 mg/kg) diabetic rats, while pancreatic GSH content, superoxide dismutase, and catalase enzymatic activities increased (p < 0.05). The IC50 values for the extract and butyl hydroxyanisole were 5.6 and 1.15 mg/mL in DPPH and 0.155 and 0.062 mg/mL in the FRAP methods, respectively. The extract had no inhibitory effect on α-amylase activity. The total amount of flavonoids of the extract was estimated to be 1.8% (g/g) on the basis of quercetin content.
Conclusion: Dracocephalum polychaetum shoot extract has antioxidant, antihyperlipidemic, and antilipid peroxidative properties.
Introduction
Type 1 diabetes mellitus is a metabolic disorder characterized by pancreas failure to secrete sufficient insulin, leading to disturbance in glucose homeostasis. During hyperglycemia, free radicals are significantly produced, which causes failure in antioxidant defense mechanism and cell damage. Increased oxidative stress is an important factor, which plays role in the development and the progression of diabetes and its complications. Natural antioxidants in medicinal plants are potential candidates for the prevention of and protection against oxidative stress resulting to cell injury (Griesmacher et al., Citation1995). Thus, the study of plant species is still a feasible research strategy in search for new antidiabetic agents. The genus Dracocephalum L. (Lamiaceae) is represented by eight species in Iran. Results of different studies about medicinal properties of different species of Dracocephalum have shown antinociceptive effect of D. kotschyi Boiss. (Golshani et al., Citation2004), as well as antioxidant (Povilaityte et al., Citation2001) and cardioprotective effects of D. moldavica L. (Najafi et al., Citation2009). It has been reported that the essential oils of two Dracocephalum species from Iran (D. polychaetum Bornm. and D. surmandinum Rech.f.) have antibacterial activity against both Gram-positive and Gram-negative bacteria (Sonboli et al., Citation2012). Also, essential oils of D. surmandinum are shown to have antioxidant and radical scavenging activities and inhibit cell growth in human breast adenocarcinoma (MCF-7) and erythromyeloblastoid leukemia cell lines (K562) (Sonboli et al., Citation2010). Dracocephalum polychaetum is a perennial woody herb and one of the endemic species that grows only in southeast of Iran in the mountainous regions of Kerman province. In folk medicine, the plant, known as Mofaroo, is used to treat abdominal pain. Also, it is a local spice for dairy products. It has been reported that flavonoids, apigenin, and luteolin are the main components of D. polychaetum methanol extract (Mehrabani et al., Citation2005). The extract is known to possess antihyperglycemic (Matsuda et al., Citation1995) and antioxidant effects (Romanova et al., Citation2001). Also, analgesic, and not the anxiolytic, activity of aqueous extract of D. polychaetum has been reported (Khodami et al., Citation2011). Since no detailed investigation has been carried out to define the antioxidant and antidiabetic properties of D. polychaetum, the current investigation was carried out to determine this.
Materials and methods
Plant material
Fresh aerial parts of D. polychaetum were collected in June 2012 from the Hezar Mountain in Kerman province, southeast of Iran, and were taxonomically identified by Dr. S.M. Mirtadzadini in the Botany Section of the Department of Biology, Shahid Bahonar University of Kerman, and a voucher specimen was deposited in the herbarium of this department.
Preparation of methanol extract of D. polychaetum
The aerial parts of the plant were air-dried in the ambient temperature, powdered, and extracted with 80% methanol by percolation as described previously (Chattopadhyay, Citation1999). After extraction, methanol was evaporated by rotary evaporator at 40 °C and the remainder was dried at 35–40 °C. The final extraction yield was about 10%. This extract was stored at 4 °C.
Determination of the main flavonoids of the extract
From stock solution of different flavonoids of rutin, quercetin, kaempferol, and catechin, an amount of 2.5 mg of rutin was dissolved in 10 mL of methanol, shaken, and filtered. An amount of 3 g of the powdered plant extract plus 30 mL of methanol was boiled for 2 min, cooled, and filtered. The filtrate was used for thin-layer chromatography (TLC). A volume of 10 µL of each sample was spotted near the bottom of a glass plate coated with silica gel GF254. The TLC plate was developed in a suit solvent system. The spotted plate was developed at the room temperature to a distance of 12 cm. The front was marked and the solvent was allowed to evaporate off at the room temperature. To detect the flavonoids on the plate, the plate was examined under UV light and also was sprayed with the reagent of the natural product.
Assay of the flavonoid content of the extract
Quercetin was used as a standard for the determination of the plant flavonoids on the basis of TLC detection. The dried extract (0.1 g) was added to acetone (20 mL). Then, the methenamine solution (1 mL) and 2 mL of HCl (25%) were added. The mixture was boiled for 30 min and filtered through cotton. The filtrate was decanted with 20 mL distilled water and 15 mL ethyl acetate. A volume of 1 mL of the AlCl3 solution was added to 10 mL of extract and diluted to 25 mL with acetic acid 5% in methanol. After 30 min, the absorbance of the samples was determined at 360 nm using a UV–visible spectrophotometer (Lambda 25, Perkin Elmer, Waltham, MA). The amount of flavonol O-glycoside was determined based on the quercetin amount as the reference (IHP, Citation2002).
Assay validation: Validation parameters of the assay were ascertained. Linearity was approved by the correlation between absorbance and concentration. The intra- and inter-day precision values were determined by measuring the absorbance in the replicate standard samples at three concentration levels (40, 80, and 120 mg/L). There were five repetitions at each concentration of samples which were assayed simultaneously within a day for verification of the intra-day assay precision. Relative standard deviation percentage (RSD%) <5 was regarded as the criterion for acceptable precision. The accuracy was measured by calculation of the error percentage.
Animals
Adult male Wistar rats, weighing 200–250 g, were used in this study. The animals were kept in the animal house of the Department of Biology in specific cages in 12 h light/dark cycle. The room temperature was controlled at 23 ± 2 °C. The animals were maintained with free access to food and tap water. The experimental procedure was confirmed by the Institutional Animal Ethical Committee of Shahid Bahonar University of Kerman.
Oral glucose tolerance test (OGTT)
The OGTT was performed for overnight-fasted normal rats to determine effective hypoglycemic dose of the extract. To this end, 30 rats were divided into five groups (six animals in each). Among the groups, one group received the drinking water, three groups received the extract at the doses of 200, 300, and 400 mg/kg; and one group received glibenclamide (20 mg/kg). Thirty min after administration of the extract, the animals received glucose (2 g/kg). Blood samples were drawn before the treatments (time zero) as well as 30, 60, and 120 min after administration of the extract. Glucose levels were estimated using a glucometer.
Experimental design
To carry out more detailed investigation on properties of the extract, according to another protocol, 36 rats were divided into six groups; six rats in each group, as follows:
Group 1 (control): normal untreated rats.
Group 2 (diabetic): diabetes was induced in rats by intraperitoneal injection of streptozotocin (STZ) (Sigma, St. Louis, MI) at the dose of 60 mg/kg b.w. dissolved in citrate buffer (0.1 M, pH = 4) to raise the blood glucose level above 300 mg/dL; considered to be diabetic after 7 d.
The other four groups: three groups of diabetic rats treated daily by oral administration of methanolic extract of D. polychaetum (200, 300, and 400 mg/kg, b.w.) and one group of diabetic rats was treated by daily administration of glibenclamide (600 µg/kg, b.w.) orally for 14 d.
After 14 d, anesthetized fasted animals sacrificed by decapitation and blood samples were collected immediately into tubes and centrifuged at 3000g for 10 min. The serum samples were stored at −20 °C for biochemical assays. An adequate volume of blood was used for the evaluation of lipid peroxidation in RBCs of normal, diabetics, and treated animals with the extract dose that showed the highest hypoglycemia (300 mg/kg). The pancreas samples were kept at −70 °C.
Pancreatic α-amylase inhibition assay (in vitro)
The methodology described by Giancarlo et al. (Citation2006) was adapted and used. Different concentrations of the extract (0.1, 0.3, 0.5, and 1 mg/mL) were prepared in DMSO. The color reagent included DNSA (Sigma, St. Louis, MO) (96 mM), and sodium and potassium tartrate (5.31 M) in sodium hydroxide (2 M). The solution of porcine pancreatic α-amylase (ppA) (Sigma, St. Louis, MO) (0.5 U/mL) was prepared in sodium phosphate buffer (20 mM, pH = 6.9) containing sodium chloride (6.7 mM). A mixture of the plant extract and enzyme solution was incubated at 25 °C for 30 min. Then, starch solution (1%) was added to a volume of the mixture and incubated at 25 °C for 3 min. The reaction was terminated by adding the color reagent and placed into a water bath at 85 °C for 15 min. The reaction mixture was then cooled and was diluted with distilled water and its absorbance value determined at 540 nm. The control tube did not contain the extract and showed 100% enzyme activity. The known porcine pancreatic α-amylase inhibitor, acarbose (Exir Co., Tehran, Iran) (0.08, 0.1, 0.3, 0.5, and 1 mg/mL) was used as the positive control. Individual blanks were used to correct the background absorbance. The inhibition percentage of α-amylase determined according to the following formula:
Evaluation of antioxidant properties of the extract
In vitro studies
(I) DPPH activity: Hydrogen atom- or electron-donating ability of the corresponding extracts was measured according to bleaching of the purple-colored methanol solution of DPPH (Merck, Darmstadt, Germany). This spectrophotometric assay uses stable DPPH radical as the reagent. Of various concentrations of the samples in methanol, 50 µL was added to 5 mL of a 0.004% methanol solution of DPPH. After a 30-min incubation period at the room temperature, the optical density (OD) of the samples was measured at 517 nm against a blank. The percentage inhibition (I%) was calculated in the following way:
Ablank shows the absorbance of the control (containing all reagents except the test compound) and Asample is the absorbance of the test compound. The sample concentration (mg/mL) that required scavenging 50% of DPPH (IC50) was calculated from the graph plotting inhibition percentage against the extract concentration (Burits et al., Citation2001).
(II) FRAP assay: The ferric reducing antioxidant power (FRAP) of D. polychaetum was determined at different concentrations based on the method of Benzie and Strain (Citation1996) with some modifications. The FRAP reagent contained 25 mL of 300 mM acetate buffer (pH = 3.6) and a volume of 2.5 mL of 2,4,6-tripyridyl-s-triazine (TPTZ) (10 mM) solution (Merck, Darmstadt, Germany) in 40 mM HCl, and 2.5 mL of 20 mM FeCl3. Then, 50 µL of the sample (the extract) was mixed with 1.5 mL FRAP reagent. The absorbance at the wavelength of 593 nm was measured after 30 min incubation.
Determination of the acute toxicity of extract
For the estimation of the lethal dose of extract (LD50), acute toxicity test was performed on normal healthy rats and animals’ behavior was observed for 48 h after the administration of different doses of the extract (500, 1000, and 1500 mg/kg b.w.). Also, blood sampling was done daily for 2 weeks to follow up health biochemical indices.
In vivo studies
Pancreas samples were homogenized in phosphate buffer (0.05 M, pH = 7) and centrifuged at 7000g at 4 °C for 2 min. The supernatant was used for assessments. The protein content of the sample was determined by the Bradford (Citation1976) method. Catalase activity measurement was performed as described by Aebi (Citation1984). The catalase activity in tissue homogenate was determined based on its ability to decompose H2O2 (30 mM) and measured at 240 nm during 1 min at 15 s intervals.
Superoxide dismutase activity was measured by the method of Giannopolitis and Ries (Citation1977). According to this method, tissue homogenate was added to the reaction solution containing potassium phosphate buffer (50 mM, pH = 7), Na–EDTA (0.1 mM), methionine (13 mM), riboflavin (75 µM), and NBT (0.075 µM). The mixture was exposed to fluorescent light for 15 min. Then, the absorbance was determined at 560 nm. Cellular GSH in pancreas tissue was determined according to the Sedlak and Lindsay’s (Citation1968) method.
Determination of antilipid peroxidation property of the extract
Blood samples were collected from normal and diabetic rats in bottles containing EDTA. Blood was centrifuged at 3000g for 10 min. Plasma was separated, buffy coat was carefully removed, and separated erythrocytes were washed three times with 0.01 M saline phosphate buffer at pH 7.4 (containing 0.15 M NaCl). After washing with 0.01 saline phosphate buffer, the method of Stocks and Dormandy (Citation1971) was used to determine lipid peroxidation in RBC samples.
Also, the method of Ohkawa et al. (Citation1979) modified by Jamall and Smith (Citation1985) was used for the determination of MDA in pancreas tissue samples. Moreover, the plasma MDA level was determined according to the method by Kurtel et al. (Citation1992).
Statistical analysis
All the data were expressed as mean ± SEM. Statistical analysis was carried out using the one-way ANOVA followed by Tukey’s post hoc test and a value of p < 0.05 was considered as a statistical significance level.
Results
Acute toxicity and the LD50 value of extract
The LD50 value of the extract should be above 1500 mg/kg b.w., because it did not result in any symptoms of toxicity or mortality up to this amount. Therefore, the methanol extract of D. polychaetum should be relatively non-toxic and safe.
Effect of the extract on OGTT
The effects of D. polychaetum extract (200, 300, and 400 mg/kg) and glibenclamide (20 mg/kg) as a reference drug on glucose tolerance of normal rats are shown in . Only 90 min after feeding the rats with glucose or 120 min after administration of the extract (300 mg/kg), the blood glucose concentration was decreased significantly by 27.1% when compared with the control rats ().
Figure 1. Effect of D. polychaetum extract and glibenclamide on glucose tolerance in glucose loaded rats. Extract administered at zero time and 2 g/kg b.w. glucose loaded 30 min after it. Blood glucose levels were analyzed at 30, 60, and 120 min in rats. Each value represents mean ± SEM, n = 6 rats per group. *p < 0.05, **p < 0.01 when compared with 2 g glucose/kg b.w. group.
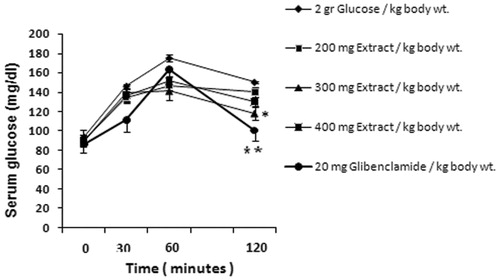
Effect of the extract on serum levels of biochemical factors (glucose, triglycerides, total cholesterol, HDL-C, creatinine, AST, and ALT)
Administration of different doses of D. polychaetum extract for 14 d did not significantly change serum glucose level when compared with control diabetic rats. Administration of the extract significantly decreased the levels of serum triglycerides (p < 0.01), total cholesterol (p < 0.01), creatinine (p < 0.01), and ALT (p < 0.05) at all doses. However, AST serum levels decreased only at the extract dose of 400 mg/kg (p < 0.05) when compared with the control diabetic rats ().
Table 1. Mean values of serum glucose, triglycerides, total cholesterol, HDL-C, creatinine, AST, and ALT for all groups.
α-Amylase inhibitory potency of the extract
Different concentrations of D. polychaetum extract (0.08–1 mg/mL) showed no inhibitory effect on α-amylase enzyme activity in vitro when compared with acarbose as a reference drug with 95.5 ± 0.5% inhibitory effect.
Antioxidant property of the extract (in vitro and in vivo)
The result of scavenging effect of plant extract on DPPH radical is given in . The results indicate that the D. polychaetum extract has notably reduced the stable free radical DPPH to the yellow-colored diphenyl-picrylhydrazyl with an IC50 value of 5.6 mg/mL in comparison with butylated hydroxyanisole (BHA) (IC50 value = 1.15 mg/mL). Also, the extract has notably reduced ferric tripyridyltriazine (Fe III TPTZ) complex to the ferrous form, which has an intense blue color, with an IC50 value of 0.156 mg/mL in comparison with BHA (IC50 value = 0.062 mg/mL; ).
Table 2. DPPH radical-scavenging activity of methanolic extract of D. polychaetum and BHA (as standard).
Table 3. The Ferric reducing antioxidant power (FRAP) of the methanolic extract of D. polychaetum and BHA (as standard).
In diabetic rats, the activities of superoxide dismutase (SOD) and catalase (CAT), and the glutathione (GSH) content in the pancreas tissue decreased significantly but in rats treated with the extract (300 mg/kg), activities of these enzymes and GSH content increased significantly ().
Table 4. SOD and CAT activities and GSH content in tissue homogenate of pancreas in different experimental groups.
Antilipid peroxidation properties of the extract
The MDA content was significantly elevated in RBCs of non-treated diabetic rats versus the control group, while the MDA level in RBCs of the extract-treated group was significantly reduced (p < 0.05). Also, diabetic rats showed a significant increase in the plasma and pancreas MDA levels while in the extract-treated rats, MDA levels significantly decreased in both plasma and pancreas (p < 0.05; ).
Table 5. MDA levels in the plasma, erythrocyte lysate, and tissue homogenate of pancreas in different experimental groups.
Assay of the total flavonoid content of the extract
The assay of the total amount of flavonoids in the methanolic extract of D. polychaetum was conducted using the method described in Iranian Plants Pharmacopeia (IHP, Citation2002). Based on the quercetin content, the amount of flavonoids was estimated to be 1.8% (g/g) in this extract.
Discussion
In the present study, experimental results indicated that diabetes causes hyperglycemia, dyslipidemia (increased cholesterol and triglycerides and decreased HDL serum levels), nephropathy (increased serum creatinine level), and hepatopathy (increased serum ALT and AST levels). Diabetes also induces oxidative stress, presented as increased plasma and pancreas MDA levels, along with decreased GSH, SOD, and CAT enzymatic activities in pancreas. The islet cells of pancreas are susceptible to oxidative damage due to the low levels of antioxidants such as SOD, glutathione, and catalase (Lenzen et al., Citation1996). Streptozotocin (STZ) is an agent commonly used for the induction of diabetes due to selective destruction of pancreas beta cells. Oxygen-free radicals have been suggested to be involved in the toxic action of STZ. The evidence for oxidative damage in diabetes has been reported by Sato et al. (Citation1979). SOD is a metalloprotein and is the first enzyme involved in the antioxidant defense by lowering the O2- level. CAT is localized in peroxisomes, which catalyzes the decomposition of H2O2 to oxygen and water, thus, prevents oxidative damage. Several studies evaluated the enzymatic antioxidant defense systems in diabetes with different results. GSH is essential for detoxification of reactive free radicals and lipid hydroperoxides and is present in large amounts in cells. Since GSH is a substrate and cofactor for glutathione peroxidase (GSH-Px), low GSH content indicates low GSH-Px activity. The formation of lipid hydroperoxides is important because they can alter membrane function and produce structural damage. Significant decline in pancreatic SOD and GSH content and increase in lipid peroxide level in this tissue have been reported in diabetic rats, which indicate increased production of ROS in this organ (Akinola et al., Citation2010; Budin et al., Citation2011; Gupta & Gupta, Citation2011). Also, high plasma MDA has been reported in diabetes (Mohora et al., Citation2006). Our findings are in accordance with these results. Diabetes develops oxidative stress in the liver (Manzari-Tavakoli et al., Citation2013) and hepatopathy is potentially considered as the result of diabetes. Elevations in serum biomarkers of liver function including ALT and AST reflect hepatocyte injury in diabetes (Harris, Citation2005). In diabetic rats, the abnormal high concentration of serum lipids has been reported, which is explained by inhibition of hormone-sensitive lipase production by insulin. Oxidative stress caused by diabetes could play a crucial role in the development and the progression of diabetic nephropathy. Elevation of important markers such as serum creatinine is related to renal dysfunction (Almadal & Vilstrup, Citation1988). The results obtained in the current study are in line with the findings of the above-mentioned studies.
In addition, in the present study, D. polychaetum extract was tested for its ability to neutralize free radicals such as DPPH. The extract exhibited free radical scavenging activity that was as good as BHA. The plant extract also showed reductive capability on reducing Fe 3+ to Fe 2+ in the FRAP assay.
Our results provided evidence that administration of D. polychaetum methanol extract in diabetic rats produced mild hypoglycemic effect in OGTT, and significantly reduced serum levels of triglycerides, cholesterol, creatinine, and ALT. Also, the extract decreased MDA and increased GSH, SOD, and CAT enzymatic activities in pancreas of treated diabetic animals. Antioxidant therapy acts as a protection against oxidative stress. Herbal products can improve antioxidant status, so, improve complications of diabetes. Recent studies have shown that plants contain many antioxidant agents like flavonoids; exert a protection against beta cell impairment. Phytochemical analysis of D. polychaetum methanol extract revealed the presence of apigenin and luteolin as two flavone aglycones (Mehrabani et al., Citation2005). The flavonoids, the most prominent plant antioxidants, are a large class of phenolic compounds, acting as free radical scavengers (Janbaz et al., Citation2002) and antihyperglycemic agents (Babu et al., Citation2007). Luteolin, a plant-derived flavonoid, possesses direct antioxidant activity and has shown the highest degree of free radical scavenging activity. This effect may be useful in the treatment of many chronic diseases such as diabetes associated with oxidative stress. Various biological actions of luteolin are mediated by inhibiting oxidative stress (Lopez-Lazaro, Citation2009). Administration of luteolin in diabetes provides protection against diabetic nephropathy or delays its development. Apigenin is a flavone that is the aglycone of several glycosides and is found mainly in plants. Due to its ability to decrease oxidative stress, apigenin plays direct roles in free radical scavenging (Pauff & Hille, Citation2009). In rats treated with apigenin, serum ALT and AST and hepatic MDA levels decreased but GSH content increased significantly (Yang et al., Citation2013). It has been reported that apigenin significantly decreases the creatinine level (Huang et al., Citation2011).
Regulation of postprandial hyperglycemia is important. In this regard, retardation of glucose absorption in small intestine is a therapeutic protocol to achieve this goal. α-Amylase is an enzyme that catalyzes starch breakdown to maltose and then glucose, which is absorbed in gut. Based on the researches available, hypoglycemic medicinal plants may act through this mechanism. However, in the current study, D. polychaetum extract showed no α-amylase inhibitory activity.
As was obtained in the current work, D. polychaetum contains antioxidant ingredients such as luteolin and apigenin and improves many diabetic disturbances. So, this plant could be a potential candidate to be further investigated in treatment of diabetes.
Acknowledgements
The authors are grateful to Dr. S.M. Mirtadzadini and Mr. Saeed Soltaninejad for their invaluable help in collection and identification of D. polychaetum.
Declaration of interest
The authors report that they have no conflicts of interest.
References
- Aebi H. (1984). Catalase in vitro. Methods Enzymol 105:121–6
- Akinola OB, Caxton-martins EA, Dini L. (2010). Chronic treatment with ethanolic extract of the leaves of Azadirachta indica ameliorates lesions of pancreatic islets in streptozotocin diabetes. Int J Morphol 28:291–302
- Almadal TP, Vilstrup H. (1988). Strict insulin therapy normalizes organ nitrogen contents and the capacity of urea nitrogen synthesis in experimental diabetes in rats. Diabetologia 31:114–18
- Babu PV, Sabitha KE, Srinivasan P, Shyamaladevi CS. (2007). Green tea attenuates diabetes induced maillard-type fluorescence and collagen cross-linking in the heart of streptozotocin diabetic rats. Pharmacol Res 55:433–40
- Benzie IF, Strain JJ. (1996). The Ferric Reducing Ability of Plasma (FRAP) as a measure of “antioxidant power”. The FRAP assay. Anal Biochem 239:70–6
- Bradford MM. (1976). A rapid and sensitive method for the quantitation of microgram quantities of protein utilizing the principle of protein-dye binding. Anal Biochem 72:248–54
- Budin SB, Hanis MM, Hamid ZA, Mohamed J. (2011). Tocotrienol-rich fraction of palm oil reduced pancreatic damage and oxidative stress in streptozotocin-induced diabetic rats. Aust J Basic Appl Sci 5:2367–74
- Burits M, Asres K, Bucar F. (2001). The antioxidant activity of the essential oils of Artemisia afra, Artemisia abyssinica and Juniperus procera. Phytother Res 15:103–8
- Chattopadhyay RR. (1999). Possible mechanism of antihyperglycemic effect of Azadirachta indica leaf extract. J Ethnopharmacol 67:373–6
- Giancarlo S, Rosa LM, Nadjafi F, Francesco M. (2006). Hypoglycaemic activity of two species extracts: Rhus coriaria L. and Bunium persicum Boiss. Nat Prod Res 20:882–6
- Giannopolitis CN, Ries SK. (1977). Superoxide dismutases. I. Occurrence in higher plants. Plant Physiol 59:309–14
- Golshani S, Karamkhani F, Monsef-esfehani HR, Abdollahi M. (2004). Antinociceptive effects of the essential oil of Dracocephalum kotschyi in the mouse writhing test. J Pharm Pharm Sci 7:76–9
- Griesmacher A, Kindhauser M, Andert SE, et al. (1995). Enhanced serum levels of thiobarbituric acid-reactive substances in diabetes mellitus. Am J Med 98:469–75
- Gupta R, Gupta RS. (2011). Antidiabetic efficacy of Mangifera indica seed kernels in rats: A comparative study with glibenclamide. Diabetol Croat 40:107–12
- Harris EH. (2005). Elevated liver function tests in type 2 diabetes. Clin Diabetes 23:115–19
- Huang J, Wang S, Zhu M, et al. (2011). Effects of genistein, apigenin, quercetin, rutin and astilbin on serum uric acid levels and xanthin oxidase activities in normal and hyperuricemic mice. Food Chem Toxicol 49:1943–7
- Iranian Herbal Pharmacopoeia (IHP). 2002. Tehran: Ministry of Health, Treatment and Medical Education Pub. p. 272
- Jamall IS, Smith JC. (1985). Effects of cadmium on glutathione peroxidase, superoxide dismutase, and lipid peroxidation in the rat heart: A possible mechanism of cadmium cardiotoxicity. Toxicol Appl Pharmacol 80:33–42
- Janbaz KH, Saeed SA, Gilani AH. (2002). Protective effect of rutin on paracetamol and CCL4-induced hepatotoxicity in rodents. Fitoterapia 73:557–63
- Khodami M, Abbasnejad M, Sheibani V, et al. (2011). Evaluation of the analgesic and anxiolytic effects of Dracocephalum polychaetum. Physiol Pharmacol 15:444–54
- Kurtel H, Granger DN, Tso P, Grisham MB. (1992). Vulnerability of intestinal interstitial fluid oxidant stress. Am J Physiol 263:G573–8
- Lenzen S, Drinkgern J, Tiedge M. (1996). Low antioxidant enzyme gene expression in pancreatic islets compared with various other mouse tissues. Free Radic Biol Med 20:463–6
- Lopez-Lazaro M. (2009). Distribution and biological activities of the flavonoid luteolin. Mini Rev Med Chem 9:31–59
- Manzari-Tavakoli A, Pouraboli I, Yaghoobi MM, et al. (2013). Antihyperglycemic, antilipid peroxidation and insulin secretory activities of Otostegia persica shoot extract in streptozotocin-induced diabetic rats and in vitro C187 pancreatic β-cells. Pharm Biol 51:253–9
- Matsuda H, Cai H, Kubo M, et al. (1995). Study on anti-cataract drugs from natural sources: II. Effects of Buddlejae Flos on in vitro aldose reductase activity. Biol Pharm Bull 18:463–6
- Mehrabani M, Roholahi S, Foroumadi A. (2005). Phytochemical studies of Dracocephalum polychaetum Bornm. J Mel Plants 4:36–42
- Mohora M, Virgolici B, Paveliu F, et al. (2006). Free radical activity in obese patients with type 2 diabetes mellitus. Rom J Intern Med 44:69–78
- Najafi M, Ghasemian E, Fathizad F, Garjani A. (2009). Effects of total extract of Dracocephalum moldavica on ischemia/reperfusion induced arrhythmias and infarct size in the isolated rat heart. Iran J Basic Med Sci 4:229–35
- Ohkawa H, Ohishi N, Yagi K. (1979). Assay for lipid peroxides in animal tissues by thiobarbituric acid reaction. Anal Biochem 95:351–8
- Pauff JM, Hille R. (2009). Inhibition studies of Bovine xanthine oxidase by luteolin, silibinin, quercetin, and curcumin. J Nat Prod 72:725–31
- Povilaityte V, Cuvelier ME, Berset C. (2001). Antioxidant properties of Moldavian dragonhead (Dracocephalum moldavica L.). J Food Lipids 8:45–64
- Romanova D, Vachalková A, Čipák L, et al. (2001). Study of antioxidant effect of apigenin, luteolin and quercetin by DNA protective method. Neoplasma 48:104–7
- Sato Y, Hotta N, Sakamoto N, et al. (1979). Lipid peroxide level in plasma of diabetic patients. Biochem Med 21:104–7
- Sedlak J, Lindsay RH. (1968). Estimation of total, protein-bound, and nonprotein sulfhydryl group in tissue with Ellmann,s reagent. Anal Biochem 25:192–205
- Sonboli A, Esmaeili MA, Gholipour A, Kanani MR. (2010). Composition, cytotoxicity and antioxidant activity of the essential oil of Dracocephalum surmandinum from Iran. Nat Prod Commun 2:341–4
- Sonboli A, Gholipour A, Yousefzadi M. (2012). Antibacterial activity of the essential oil and main components of two Dracocephalum species from Iran. Nat Prod Res 26:2121–5
- Stocks J, Dormandy TL. (1971). The autoxidation of human red cell lipids induced by hydrogen peroxide. Br J Haematol 20:95–111
- Yang J, Wang XY, Xue J, et al. (2013). Protective effect of apigenin on mouse acute liver injury induced by acetaminophen is associated with increment of hepatic glutathione reductase activity. Food Funct 4:939–43