Abstract
Context: Organic anion-transporting polypeptide 2B1 (OATP2B1) which is highly expressed in enterocytes and hepatocytes could be a key determinant for the intestinal absorption and hepatic uptake of its substrate drugs. Natural products are commonly used in traditional Chinese medicine, foods, and beverages.
Objective: The objective of this study is to determine the OATP2B1-mediated drug interactions that could occur between natural products and OATP2B1 substrate drugs.
Materials and methods: Human OATP2B1 was transiently expressed in human embryonic kidney (HEK293) cells and characterized by immunofluorescence, Western blot, and uptake assay. Liquid chromatography–tandem mass spectrometry (LC–MS/MS) methods for detecting OATP2B1 substrates estrone-3-sulfate (E3S) and three statins had been developed and were employed to investigate the effects of 27 frequently used natural products on the function of OATP2B1. Uptake of 5 μM E3S and 1 μM statins in the absence or presence of natural products was measured at 37 °C for 2 min with empty vector- and OATP2B1-transfected HEK293 cells. The IC50 values of inhibitors for OATP2B1-mediated 5 μM E3S uptake were determined.
Results: Our results showed that mulberrin, scutellarin, quercetin, and glycyrrhetinic acid were strong inhibitors of OATP2B1-mediate E3S uptake with IC50 values being 1.8, 2.0, 7.5, and 13.0 μM, which were comparable with their plasma concentrations in clinical trials. They also inhibited OATP-mediated uptake of atorvastatin, fluvastatin, and rosuvastatin. These results indicated that clinically relevant drug interactions could occur between these natural compounds and OATP2B1 substrate drugs.
Discussion and conclusion: The information obtained from this study might be helpful to predict and to avoid potential OATP2B1-mediated drug interactions.
Introduction
Pharmacokinetic drug–drug interaction is a serious concern when treating patients with multiple drugs at the same time. In general, drug–drug interactions result in the alteration of drug absorption, distribution, metabolism, and/or excretion of another co-administered drug, thereby leading to changes in drug efficacy and safety (Koenen et al., Citation2011). Uptake transporters belonging to the superfamily of solute carriers (SLC) are increasingly recognized as important factors in the absorption and disposition of drugs in the body, and modification of transporter functions by drug competition is an important mechanism underlying drug–drug interactions (Hagenbuch & Meier, Citation2004; Hediger et al., Citation2004; König et al., Citation2006). Drug transporters expressed in the small intestine, liver, and kidney are important determinants of the pharmacokinetic profiles of drugs (Muller & Fromm, Citation2011). Among them, the organic anion-transporting polypeptides (OATPs) have been shown to interact with a variety of drugs in clinical use (Koenen et al., Citation2011).
OATP2B1, which is localized in the apical membrane of enterocytes (Kobayashi et al., Citation2003) and sinusoidal membrane of hepatocytes (Kullak-Ublick et al., Citation2001) as well as in various other tissues (Bronger et al., Citation2005; Grube et al., Citation2006b; St-Pierre et al., Citation2002), could be a key determinant for the intestinal absorption and hepatic uptake of its substrates. OATP2B1 transports bromosulfophthalein (BSP), estrone-3-sulfate (E3S), dehydroepiandroserone-3-sulfate (DHEAS), taurocholate, and some drugs such as aliskiren, fexofenadine, glibenclamide, and statins (Hagenbuch & Gui, Citation2008; Roth et al., Citation2012). Inhibition of OATP2B1-mediated drug absorption in the intestine and drug uptake into the liver could lead to clinically relevant drug–drug and drug–food interactions. It was reported that grapefruit and orange juices significantly inhibited OATP2B1-mediated uptake of E3S and glibenclamide (Satoh et al., Citation2005; Shirasaka et al., Citation2013a) while apple and orange juices induced a remarkable decrease in OATP2B1-mediated fexofenadine uptake (Imanaga et al., Citation2011; Shirasaka et al., Citation2013b), indicating that citrus juices might inhibit the intestinal absorption of anionic drugs. In vivo studies showed that grapefruit, orange, and apple juices greatly reduced the plasma concentration of aliskiren, probably by inhibiting its OATP2B1-mediated influx in the small intestine (Tapaninen et al., Citation2010, Citation2011). Fuchikami et al. (Citation2006) showed that some herbal extracts used in dietary supplements significantly inhibited the function of OATP2B1, suggesting that co-administration of some dietary supplements might decrease the absorption of orally administered substrates of OATP2B1. Roth et al. (Citation2011) reported that green tea catechins epicatechin gallate and epigallocatechin gallate significantly inhibited OATP2B1-mediated E3S uptake. Several flavonoids, silymarin flavonolignans, and antidiabetic drugs have also been reported to inhibit OATP2B1 in vitro (Bachmakov et al., Citation2008; Klatt et al., Citation2013; Kock et al., Citation2013; Li et al., Citation2014; Mandery et al., Citation2010). These reports demonstrated that OATP2B1 could interact with a wide range of natural products.
Plant natural products are commonly used in traditional Chinese medicine, foods, beverages, and dietary supplements. It is quite common that a patient taking drugs is in concomitant consumption of fruit juices, teas, nutritives, etc. To identify more OATP2B1 modulators from natural products, in this study the effects of 27 frequently used natural products on OATP2B1-mediated E3S uptake have been investigated using LC-MS/MS. These natural products were isolated from various plants including Astragalus membranaceus (Fisch.) Bunge (Leguminosae), Chrysanthemum morifolium Ramat. (Compositae), Citrus aurantium L. (Rutaceae), Dioscorea nipponica Makino (Dioscoreaceae), Erigeron breviscapus (Vant.) Hand.-Mazz. (Compositae), Glycine max (L.) Merr. (Leguminosae), Glycyrrhiza glabra L. (Leguminosae), Hypericum perforatum L. (Guttiferae), Morus alba L. (Moraceae), Paeonia veitchii Lynch (Paeoniaceae), Pueraria lobate (Willd.) Ohwi (Leguminosae), Stevia rebaudiana Bertoni (Compositae), and Taxus chinensis (Pilger) Rehd. (Taxaceae). Several natural products that showed significant effect on E3S uptake have been further checked for their effects on OATP2B1-mediated uptake for three statins, namely atorvastatin, fluvastatin, and rosuvastatin, to see whether their effects are substrate dependent. The information obtained from this study might be helpful to predict and to avoid potential OATP2B1-mediated drug–drug and drug–food interactions.
Materials and methods
Chemicals and reagents
Atorvastatin, betamethasone, BSP, E3S, fluvastatin, isoquercitrin, and rosuvastatin were purchased from Sigma-Aldrich (St. Louis, MO). Diosgenin and rutin were obtained from Adamas Reagent Co., Ltd. (Shanghai, China). Quercetin was obtained from Sinopharm Chemical Reagent Co., Ltd. (Shanghai, China) and chlorogenic acid was from the National Institute for the Control of Pharmaceutical and Biological Products (Beijing, China). Scutellarin (scutellarein-7-O-glucuronide) was purchased from Shanghai Standard Biotech Co., Ltd. (Shanghai, China) and steviol glucuronide (SV-G) was from PharmaResources (Shanghai) Co., Ltd. (Shanghai, China). Astragaloside A, daidzin, dioscin, glycyrrhetinic acid, glycyrrhizic acid, hyperoside, liquiritin, paeoniflorin, puerarin, quercitrin, and vitexin were purchased from Nanjing Spring & Autumn Biological Engineering Co., Ltd. (Nanjing, Jiangsu, China). Cephalomannine, mulberrin, mulberroside A, taxachitriene A, taxuspine W, chrysanthemum stem-leaf flavonoids, and other natural product extracts were gifts from the Laboratory of Natural Medicinal Chemistry of Soochow University (Suzhou, Jiangsu, China). The content of total flavonoids in chrysanthemum stem-leaf extract was ∼85% (UV). The purity of cephalomannine, isoquercitrin, taxachitriene A, and taxuspine W was ≥90% and that of betamethasone was ≥95% (HPLC). All other listed chemicals had a purity of ≥98% (HPLC). Fetal bovine serum (FBS), Dulbecco's Modified Eagle's medium (DMEM), and trypsin were from Hyclone (Logan, UT). Lipofectamine 2000 and Opti-MEM were purchased from Invitrogen (Carlsbad, CA). Poly-d-lysine was purchased from Sigma-Aldrich (St. Louis, MO). The BCA protein assay kit was from Pierce Chemical (Rockford, IL). Antibodies for detecting the six-His tag and the Na+/K+-ATPase α subunit were purchased from Tiangen (Beijing, China) and Epitomics (Burlingame, CA). Fluorescein- and horseradish peroxidase-conjugated secondary antibodies were purchased from ProSci (Poway, CA) and ProteinTech (Chicago, IL). Immobilon Western blot detection kit was from Millipore (Billerica, MA).
OATP2B1 expression
Human embryonic kidney (HEK293) cells were cultured and transfected as described previously (Gui & Hagenbuch, Citation2008). In brief, HEK293 cells were grown at 37 °C in a humidified 5% CO2 atmosphere in DMEM medium supplemented with 10% FBS. HEK293 cells were transiently transfected with OATP2B1*3 (Nozawa et al., Citation2002) subcloned into pcDNA5/FRT or pcDNA5/FRT vector alone using Lipofectamine 2000 (Invitrogen, Carlsbad, CA) according to the instruction of the manufacturer. A six-His tag was introduced at the C-terminal end of the open-reading frame of OATP2B1*3. Transfected cells were incubated for 24 h at 37 °C and then used for immunofluorescence, surface biotinylation, and transport assay.
Immunofluorescence
For immunofluorescence, 100 000 cells were plated on culture slides and transfected on the second day. After 24 h transfection, cells were fixed in 2% paraformaldehyde and 1% Triton X-100 in phosphate-buffered saline (PBS) for 15 min at room temperature. After washing, the cells were incubated with 5% bovine serum albumin (BSA) in PBS at room temperature for 1 h. After another wash with PBS, cells were incubated with a mouse anti-His antibody (Tiangen, Beijing, China; 1:1000) in 1% BSA in PBS. Cells were then washed with PBS and incubated with fluorescein-conjugated goat anti-mouse IgG (H+L; ProSci, Poway, CA; 1:100) for 1 h at room temperature. After a final wash with PBS, slides were mounted with VECTASHIELD-mounting medium (Vector Laboratories, Burlingame, CA) and used for fluorescence microscopy analysis.
Cell surface biotinylation and immunoblot analysis
HEK293 cells were grown in poly-d-lysine coated six-well plates and transfected as described above. Biotinylation experiments were conducted 24 h post-transfection. Cells were washed twice with 2 mL of ice-cold PBS and then treated with 1 mL of sulfo-N-hydroxysuccinimide-SS-biotin (1 mg/mL in PBS) for 1 h at 4 °C. Then, cells were washed three times with 2 mL of ice-cold PBS containing 100 mM glycine and incubated for 10 min at 4 °C with the same buffer. After being washed with PBS three times, cells were lysed with 700 μL of lysis buffer (10 mM Tris, 150 mM NaCl, 1 mM EDTA, 0.1% SDS, and 1% Triton X-100, pH 7.4, containing protease inhibitors) for 1 h at 4 °C with shaking. Lysates were centrifuged at 10 000 × g for 2 min. Supernatants were incubated with 140 μL of streptavidin–agarose beads for 1 h at room temperature with constant agitation. Beads were then pelleted at 850 × g for 1 min and washed three times with ice-cold lysis buffer. Cell surface proteins were recovered from the resin by incubation of the beads with 150 μL of 2 × Laemmli buffer containing 100 mM dithiothreitol at room temperature for 30 min. Cell membrane proteins were then subjected to SDS-polyacrylamide gel electrophoresis and immunoblot analysis. OATP2B1 was detected with a mouse anti-His antibody (Tiangen, Beijing, China; 1:1000), followed by HRP-conjugated goat anti-mouse IgG (H+L); ProteinTech, Chicago, IL) (1:2000). Plasma membrane marker Na+/K+-ATPase was detected with a rabbit anti-Na+/K+-ATPase α subunit antibody (Epitomics, Burlingame, CA) (1:2500), followed by HRP-conjugated mouse anti-rabbit IgG (ProSci, Poway, CA) (1:5000). Immunoblots were developed with Immobilon Western chemiluminescence kit (Millipore, Billerica, MA) and detected with X-ray film.
Uptake assay
HEK293 cells were seeded in poly-d-lysine coated 24-well plates and transfected with Lipofectamine 2000 (Invitrogen, Carlsbad, CA). Transport assays were performed 24 h post-transfection. Cells were washed three times with 1 mL of pre-warmed uptake buffer (100 mM NaCl, 2 mM KCl, 1 mM CaCl2, 1 mM MgCl2, 10 mM Hepes, pH adjusted to 7.4 with Trizma base) and uptake was started by adding 200 μL of uptake buffer containing substrate and compounds to be tested. After incubation, uptake was ceased by removing the uptake solution and washing the cells four times with 1 mL of ice-cold uptake buffer. The cells were lysed with 200 μL of 0.2 N NaOH at 4 °C for 60 min and then 200 μL of 0.2 N HCl were added to neutralize the solution. Then 100 μL of neutralized lysate were transferred into a new Eppendorf tube and 100 μL of acetonitrile containing internal standards (IS) (100 ng/mL betamethasone for E3S, 100 nM verapamil for atorvastatin, 100 nM atorvastatin for fluvastatin and rosuvastatin) were added with thorough mixing. The mixture was then centrifuged at 13 800 × g for 10 min. Each supernatant was transferred to an auto sampler vial and 10 μL was injected into the LC-MS/MS system for analysis. The total protein concentration for each well was measured using a bicinchoninic acid (BCA) protein assay kit (Pierce, Rockford, IL) and the uptake in each well was normalized to its total protein concentration. Cells transfected with empty vector served as the background control. Transporter-specific uptake was calculated by subtracting the background uptake of vector transfected cells from uptake of OATP2B1-transfected cells.
LC-MS/MS analyses of E3S and statins
Instrumentation
Chromatography was performed using a Shimadzu HPLC system consisting of a DGU-20A3 degasser, two LC-20AD pumps, a SIL-20A auto-sampler, a CTO-20A column temperature oven, and a CBM-20A communications bus module (Shimadzu Corporation, Kyoto, Japan). Mass spectrometric detection was achieved with an AB SCIEX 4000 Qtrap system (Applied Biosystems, Foster City, CA) equipped with a Turbo IonSpray (ESI) ionization source. The data acquisition and processing were performed using the Analyst 1.5.2 software (Applied Biosystems, Foster City, CA).
Chromatography
Chromatographic separation was achieved with an Agela Venusil C18 column (2.1 mm × 50 mm, 5 μm) (Agela Technologies Inc., Tianjin, China) and the column was maintained at 40 °C. The flow rate was set at 0.3 mL/min. Optimal mobile phase conditions with a stepwise gradient elution were figured out for each substrate. For E3S, the mobile phase consisted of 5 mM ammonium acetate aqueous solution (A) and methanol (B) with the following gradient: 0–0.5 min, 40% B; 3 min, 95% B; 3.0–4.0 min, 95% B; and 4.5–6.0 min, 40% B. The total run time was 6 min. For atorvastatin, fluvastatin and rosuvastatin, the mobile phase consisted of 0.1% formic acid aqueous solution (A) and 0.1% formic acid methanol solution (B). The total run time for atorvastatin was 5.5 min with the following gradient: 0–0.5 min, 20% B; 2 min, 95% B; 2–3.5 min, 95% B; 4–5.5 min, 25% B. The total run time for fluvastatin was 6 min with the following gradient: 0–0.5 min, 25% B; 1.5 min, 90% B; 1.5–4.5 min, 90% B; 4.6–6 min, 25% B. The total run time for rosuvastatin was 6 min with the following gradient: 0–0.5 min, 15% B; 2.5 min, 95% B; 2.5–4.5 min, 95% B; 4.6–6 min, 15% B.
Mass spectrometry
The mass spectrometer was operated in the negative ionization mode for E3S and fluvastatin and positive ionization mode for atorvastatin and rosuvastatin under ESI condition, and quantitation was performed by multiple reaction monitoring (MRM) with a dwell time set to be 100 ms. The ion transitions were selected and other MS parameters were optimized for the best sensitivity for each analyte as listed in .
Table 1. Selected ion transitions and MS parameters.
Data analysis
Uptake experiments were performed in triplicate and repeated two to three times. Data with error bars represent the mean ± SD. To analyze whether the groups were different from the control, one-way ANOVA was performed followed by Dunnett's test with Prism 5 (GraphPad Software, Inc., La Jolla, CA). The p value for statistical significance was set to be <0.05. The IC50 values were calculated by non-linear least squares regression.
Results
Characterization of OATP2B1-expressing cells
Human OATP2B1 was transiently expressed in HEK293 cells. The expression of OATP2B1 was checked by immunofluorescence, surface biotinylation, and Western blot analysis. Immunofluorescence showed that OATP2B1 had been successfully expressed in HEK293 cells (). To confirm that OATP2B1 was properly located at the plasma membrane, we performed Western blot analysis with an anti-His antibody after purifying membrane proteins by surface biotinylation. We used the α subunit of Na+/K+-ATPase as a plasma membrane marker and the anti-His tag antibody detected a band at ∼85 kDa for OATP2B1 (), which is consistent with the reported molecular mass of OATP2B1 (Grube et al., Citation2006b; Kullak-Ublick et al., Citation2001; St-Pierre et al., Citation2002). The surface expression level of OATP2B1 was ∼4.5-folds of that of endogenous Na+/K+-ATPase. To functionally characterize the transiently expressed OATP2B1 and measure some basic kinetic constants, the OATP2B1 model substrate E3S was used. OATP2B1-mediated uptake of 1 and 50 μM E3S was linear for 3 min (data not shown). Therefore, subsequent transport assays were performed at 2 min for OATP2B1. showed an example of saturation kinetics for OATP2B1-mediated E3S uptake. Uptake was saturable with apparent Km and Vmax values of 13.8 ± 2.5 μM and 518 ± 30 pmol/mg/min, respectively. The Km value is comparable with the values published in the literature (Grube et al., Citation2006a; Nozawa et al., Citation2004; Tamai et al., Citation2001).
Figure 1. Characterization of OATP2B1-expressing HEK293 cells with immunofluorescence, surface biotinylation and Western blot analysis, and kinetic study. (A) Immunofluorescence of pcDNA5/FRT empty vector (upper panel) and pcDNA5/FTR-OATP2B1 (lower panel) transfected HEK293 cells. Cells were grown and transfected on culture slide and stained with an anti-His antibody. (B) Western blot analysis of surface biotinylated proteins detected with an anti-His antibody. The plasma membrane marker Na+/K+-ATPase α subunit was used as a positive control. (C) Kinetic study of OATP2B1-mediated E3S uptake. Uptake of increasing concentrations of E3S was measured at 37 °C for 2 min with empty vector- and OATP2B1-transfected HEK293 cells. After subtracting the values obtained with empty vector-transfected cells, net OATP2B1-mediated uptake was fitted to the Michaelis–Menten equation to obtain Km and Vmax values. Means ± SD of triplicate determinations were given.
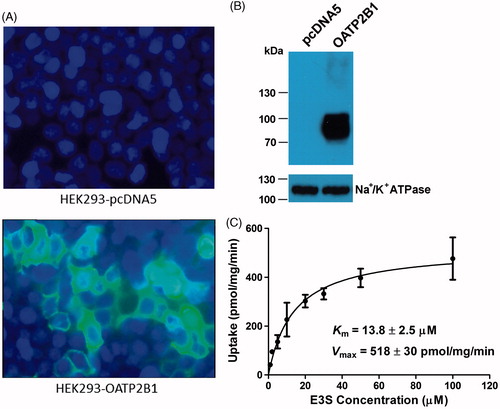
Effects of natural products on OATP2B1-mediated E3S transport
E3S is one of the typical and well-characterized OATP2B1 substrates which are amphipathic organic compounds containing hydrophobic and negatively charged groups. To identify potential modulators of OATP2B1 from natural products, uptake experiments were performed for E3S with 27 natural compounds and extracts which originated from various plants including A. membranaceus, C. morifolium, C. aurantium, D. nipponica, E. breviscapus, G. max, G. glabra, H. perforatum, M. alba, P. veitchii, P. lobate, S. rebaudiana, and T. chinensis. Uptake of 5 μM E3S in the absence and presence of natural products with the empty vector- and OATP2B1-transfected HEK293 cells was measured. The results are summarized in . The absolute amount of E3S transported by OATP2B1 into cells in the absence of inhibitor in a 24-well plate was ∼70 pmol/well. BSP, a known OATP2B1 inhibitor (Kis et al., Citation2010; Lan et al., Citation2009), was used as a positive control and it strongly inhibited OATP2B1-mediated E3S uptake by approximately 90%. Chrysanthemum stem-leaf flavonoids, glycyrrhetinic acid, mulberrin, quercetin, and scutellarin were strong inhibitors of OATP2B1 with more than 80% inhibition. Astragaloside A, hyperoside, isoquercitrin, 40% and 90% ethanol eluates of mulberry bark, and rutin were moderate inhibitors with 50–80% inhibition, while daidzin, dioscin, glycyrrhizic acid, hesperidin, liquiritin, paeoniflorin, quercitrin, taxuspine W, and vitexin were weak inhibitors with 20–50% inhibition of OATP2B1-mediated E3S uptake. Diosgenin, mulberroside A, puerarin, steviol glucuronide, and taxachitriene A had no significant effect on E3S uptake. On the contrary, cephalomannine and chlorogenic acid showed a stimulating effect on OATP2B1-mediated E3S uptake.
Figure 2. Effects of tested natural products on OATP2B1-mediated E3S uptake. Uptake of 5 μM E3S was measured at 37 °C for 2 min with empty vector- and OATP2B1-transfected HEK293 cells in the absence or presence of the indicated natural products. Values obtained with empty vector-transfected cells were subtracted from values obtained with OATP2B1-transfected cells and were given as percent of control. Means ± SD of triplicate determinations were given; *p < 0.05.
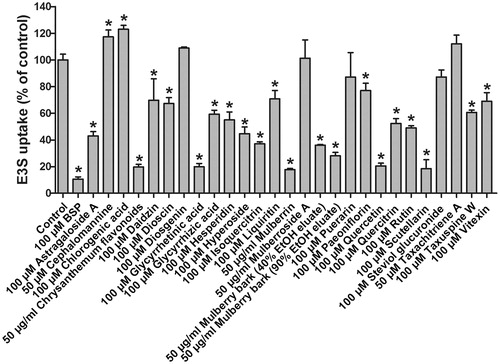
IC50 values of natural products on OATP2B1-mediated E3S transport
Twelve natural products which showed more than 40% inhibition of OATP2B1-mediated E3S transport were selected to carry out concentration-dependent inhibition experiments to determine their IC50 values (). The IC50 value for astragaloside A could not be determined due to its low solubility. Among the characterized compounds, mulberrin and scutellarin were the strongest inhibitors with IC50 values being 1.8 and 2.0 μM, respectively. Glycyrrhizic acid and quercitrin were the weakest inhibitors with IC50 values being 125.7 and 100.3 μM, respectively.
Table 2. Inhibition rates and IC50 values of tested natural products on OATP2B1-mediated E3S uptake.
Effects of several modulators on OATP2B1-mediated statins transport
Several modulators which showed significant effects on E3S uptake had been further checked for their effects on OATP2B1-mediated uptake for three statins, namely atorvastatin, fluvastatin, and rosuvastatin, to see whether their effects are substrate-dependent. These modulators included five strongest inhibitors which were chrysanthemum flavonoids, glycyrrhetinic acid, mulberrin, quercetin, and scutellarin, and a stimulator chlorogenic acid (). Uptake of 1 μM atorvastatin, fluvastatin, and rosuvastatin in the absence and presence of natural products with the empty vector- and OATP2B1-transfected HEK293 cells was measured. The absolute amounts of atorvastatin, fluvastatin, and rosuvastatin transported by OATP2B1 into cells in the absence of inhibitor in a 24-well plate were about 6, 38, and 0.9 pmol/well, respectively. As shown in , all five inhibitors for E3S also showed significant inhibition for OATP2B1-mediated transport of atorvastatin, fluvastatin, and rosuvastatin with the strongest inhibition for rosuvastatin. However, the weak stimulator chlorogenic acid for E3S exhibited inhibitory effect on OATP2B1-mediated statins transport.
Figure 3. Effects of several modulators on OATP2B1-mediated uptake of (A) atorvastatin, (B) fluvastatin, and (C) rosuvastatin. Uptake of 1 μM atorvastatin, fluvastatin, and rosuvastatin was measured at 37 °C for 2 min with empty vector- and OATP2B1-transfected HEK293 cells in the absence or presence of the indicated natural products. Values obtained with empty vector-transfected cells were subtracted from values obtained with OATP2B1-transfected cells and were given as percent of control. Means ± SD of triplicate determinations were given; *p < 0.05.
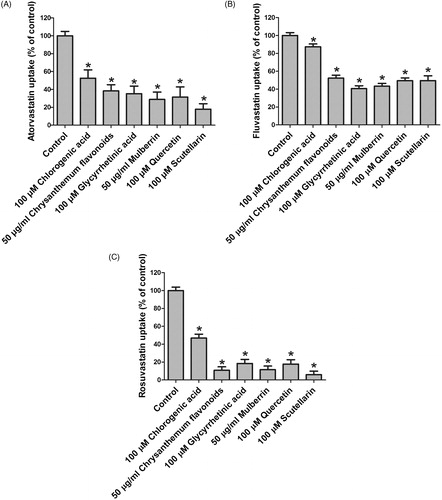
Discussion
OATP2B1, which is expressed in the liver (Kullak-Ublick et al., Citation2001) and small intestine (Kobayashi et al., Citation2003) as well as other organs (Roth et al., Citation2012), transports BSP, E3S, DHEAS, taurocholate, and some drugs such as aliskiren, fexofenadine, statins, and glibenclamide (Hagenbuch & Gui, Citation2008; Roth et al., Citation2012). The liver and the small intestine are the places where the likelihood of drug–drug or drug–food interactions is the greatest. Inhibition or stimulation of OATP2B1's function by drugs or foods could alter the pharmacokinetics of OATP2B1 substrates and potentially lead to adverse effects. Quite a few interactions between OATP2B1 and natural products have been reported in the literature (Fuchikami et al., Citation2006; Imanaga et al., Citation2011; Kock et al., Citation2013; Li et al., Citation2014; Mandery et al., Citation2010; Satoh et al., Citation2005; Shirasaka et al., Citation2013a,Citationb; Tapaninen et al., Citation2010, Citation2011; Roth et al., Citation2011), suggesting that OATP2B1 could interact with a wide range of natural products. To identify more OATP2B1 modulators from natural products and to avoid potential adverse drug–drug and drug–food interactions, in this work the effects of 27 natural compounds and extracts on the function of OATP2B1 have been investigated. These natural products were from various plants including A. membranaceus, C. morifolium, C. aurantium, D. nipponica, E. breviscapus, G. max, G. glabra, H. perforatum, M. alba, P. veitchii, P. lobate, S. rebaudiana, and T. chinensis, which are frequently consumed as Chinese herbal medicine, fruits, vegetables, and drinks. Our results showed that chrysanthemum stem–leaf flavonoids, glycyrrhetinic acid, mulberrin, quercetin, and scutellarin were strong inhibitors of OATP2B1 with inhibition rates greater than 80% and IC50 values less than 15 μM ( and ). They also showed inhibitory effect on OATP2B1-mediated transport for lipid-lowering drugs atorvastatin, fluvastatin, and rosuvastatin ().
Quercetin (3,3′,4′,5,7-pentahydroxyflavone), a flavonol, is widely distributed in plants, e.g., in fruits, vegetables, leaves, and grains (Beecher, Citation2003; Shirasaka et al., Citation2013a; Woo & Kim, Citation2013). In Western populations, the estimated daily intake of flavonols is in the range of 20–50 mg/d. Of this, about 13.8 mg/d is in the form of quercetin-type flavonols (Cao et al., Citation2010). Several studies showed that quercetin had an inhibitory effect on OATP2B1's function (Mandery et al., Citation2010; Satoh et al., Citation2005; Shirasaka et al., Citation2013a). Our results were consistent with these reports ( and , and ). Most of the dietary intake of quercetin-type flavonols is as quercetin glycosides (Kelly, Citation2011). However, little is known about the effect of quercetin glycosides on OATP2B1's function. The only two reports were about the effect of rutin (quercetin-3-O-rutinoside) on OATP2B1, but their conclusions were controversial. Fuchikami et al. (Citation2006) showed that rutin had an inhibitory effect on OATP2B1-mediated uptake of E3S, whereas Ogura et al. (Citation2014) showed that rutin stimulated uptake of E3S by OATP2B1. Therefore, in this study, we checked the effects of four quercetin glycosides on OATP2B1's function, namely quercitrin (quercetin-3-O-rhamnoside), isoquercitrin (quercetin-3-O-glucoside), hyperoside (quercetin-3-O-galactoside), and rutin. The chemical structures of quercetin and its four glycosides are shown in . Our results showed that rutin was an inhibitor of OATP2B1 with an inhibition rate of 51% and an IC50 value of 60.7 μM ( and ). The inhibition activity of quercetin was higher than its glycoside forms. The IC50 values for quercetin, hyperoside, isoquercitrin, rutin, and quercitrin were 7.5, 29.4, 30.6, 60.7, and 100.3 μM, respectively (). A potential explanation for this phenomenon is that the binding site of quercetin is not large enough to accommodate quercetin glycosides very well. The only difference of the structures of hyperoside and isoquercitrin is the configuration of a hydroxyl group in their sugar moieties. Therefore, the activities of hyperoside and isoquercitrin were comparable (). However, when their hydroxymethyl group was replaced by a methyl group as in quercitrin, their activities decreased by 3-fold with IC50 values increasing from ∼30 μM to 100 μM, indicating that the hydroxyl group was important for their binding. Liquiritin and puerarin, a 4′-O-glucoside of a flavanone and an 8-C-glucoside of an isoflavone (), also showed weak inhibitory effects with inhibition rates of 30% and 13%, respectively ().
Two other flavones, mulberrin and scutellarin, also exhibited strong inhibition of OATP2B1-mediate E3S transport with IC50 values being 1.8 and 2.0 μM, respectively ( and ). In addition, they showed strong inhibition for OATP2B1-mediated statins transport (). Mulberrin is a flavone with two isopentenyl groups at positions 3 and 8, and its scaffold is close to the structure of quercetin (). The isopentenyl group is smaller and more flexible than the glycosyl group, and thus mulberrin could probably be much easier to fit into its binding site than quercetin glycosides. Scutellarin (scutellarein-7-O-glucuronide) is a flavone with a glucuronosyl group at position 7. The carboxyl group which makes scutellarin an organic anion could contribute to its binding with OATP2B1. It was reported that OATP2B1 played a predominant role in the hepatic uptake of scutellarin (Gao et al., Citation2012). Therefore, it seems that scutellarin is not only an inhibitor but also a substrate of OATP2B1. Scutellarin has been widely used for the clinical treatment of cardiovascular and cerebrovascular diseases in China for many years. It is the main effective constituent of breviscapine, a flavonoid mixture isolated from the dried whole plant of E. breviscapus (Cui et al., Citation2013). An animal study showed that the plasma concentration of scutellarin could reach ∼4 mg/L (8.6 μM) after oral administration of 400 mg/kg scutellarin in both male and female rats (Xing et al., Citation2011). A clinical study showed that the peak plasma concentration of scutellarin aglycone was 0.9 mg/L (3.1 μM) in healthy volunteers with a single oral dose of 120 mg breviscapine tablets (Ju et al., Citation2005). The blood concentration of scutellarin is higher than its IC50 value for OATP2B1 inhibition, and thus a potential drug–drug interaction could occur when an OATP2B1 substrate drug is co-administered with scutellarin.
Chrysanthemum stem–leaf flavonoids are a flavonoid mixture isolated from the stems and leaves of C. morifolium, which also showed strong inhibition on OATP2B1-mediated transports for E3S and three statins with an IC50 value of 2.3 mg/L for E3S ( and , and ). Chrysanthemum tea is popular in China. However, due to its strong inhibitory effect on OATP2B1, it is better to avoid drinking chrysanthemum tea when patients take OATP2B1 substrate drugs to prevent potential drug–food interaction.
Licorice root has been frequently used in traditional Chinese medicine and due to its sweet taste it has also been constantly used as flavoring and sweetening agents in foods, beverages, candies, and dietary supplements (Kao et al., Citation2013; Lin et al., Citation2009). The main bioactive components of licorice root are a pentacyclic triterpenoid glycoside glycyrrhizic acid, and its aglycone and major metabolite glycyrrhetinic acid (; Nafisi et al., Citation2012). Our results showed that glycyrrhetinic acid and glycyrrhizic acid were inhibitors for OATP2B1-mediated E3S transport with IC50 values of 13 and 126 μM, respectively ( and ). Therefore, glycyrrhetinic acid has almost 10-fold stronger inhibitory activity on OATP2B1 than glycyrrhizic acid. Glycyrrhetinic acid also showed strong inhibition for OATP2B1-mediated statins transport (). Glycyrrhizic acid is a large molecule with a disaccharide attached to its aglycone and probably it is too big to fit into the binding pocket. A clinical study showed that the peak plasma concentration of glycyrrhetinic acid could reach 4.5–9.0 mg/L (9.6–19.2 μM) after the administration of 500–1500 mg oral doses to healthy volunteers (Krahenbuhl et al., Citation1994). The plasma concentration of glycyrrhetinic acid is in the same range as its IC50 value for OATP2B1 inhibition, indicating glycyrrhetinic acid could cause an OATP2B1-mediated drug interaction.
Conclusion
OATP2B1, which is expressed in significant amounts in the small intestine and liver, is involved in drug absorption and disposition, and thus its functional change could lead to clinically significant drug–drug interactions. Natural products are widely used in herbal remedies, foods, beverages, and dietary supplements. To identify potential OATP2B1 modulators from natural products, in the present study, the effects of 27 frequently used natural compounds and extracts on the function of OATP2B1 have been investigated. Our results showed that flavonoids usually had stronger inhibitory effects than their glycoside forms, suggesting that larger molecular volume might be not beneficial for their binding. However, flavonoid monoglucuronides such as scutellarin showed strong inhibition of OATP2B1, probably due to the carboxyl group which makes the compound an organic anion. The IC50 values of several natural products such as scutellarin and glycyrrhetinic acid were comparable with their plasma concentrations in clinic, indicating potential drug interactions could occur when OATP2B1 substrate drugs are co-administered with these natural products. In conclusion, our study might provide some information to predict and to avoid potential OATP2B1-mediated adverse drug–drug and drug–food interactions.
Acknowledgements
We thank Dr. Jiangyun Liu for his kind gift of some natural compounds.
Declaration of interest
The authors report that they have no conflicts of interest. This work is financially supported by grant from National Natural Science Foundation of China (31200623).
References
- Bachmakov I, Glaeser H, Fromm MF, Konig J. (2008). Interaction of oral antidiabetic drugs with hepatic uptake transporters: Focus on organic anion transporting polypeptides and organic cation transporter 1. Diabetes 57:1463–9
- Beecher GR. (2003). Overview of dietary flavonoids: Nomenclature, occurrence and intake. J Nutr 133:3248S–54
- Bronger H, Konig J, Kopplow K, et al. (2005). ABCC drug efflux pumps and organic anion uptake transporters in human gliomas and the blood–tumor barrier. Cancer Res 65:11419–28
- Cao J, Zhang Y, Chen W, Zhao X. (2010). The relationship between fasting plasma concentrations of selected flavonoids and their ordinary dietary intake. Br J Nutr 103:249–55
- Cui MY, Tian CC, Ju AX, et al. (2013). Pharmacokinetic interaction between scutellarin and valsartan in rats. Acta Pharm Sin 48:541–6
- Fuchikami H, Satoh H, Tsujimoto M, et al. (2006). Effects of herbal extracts on the function of human organic anion-transporting polypeptide OATP-B. Drug Metab Dispos 34:577–82
- Gao C, Zhang H, Guo Z, et al. (2012). Mechanistic studies on the absorption and disposition of scutellarin in humans: Selective OATP2B1-mediated hepatic uptake is a likely key determinant for its unique pharmacokinetic characteristics. Drug Metab Dispos 40:2009–20
- Grube M, Kock K, Karner S, et al. (2006a). Modification of OATP2B1-mediated transport by steroid hormones. Mol Pharmacol 70:1735–41
- Grube M, Kock K, Oswald S, et al. (2006b). Organic anion transporting polypeptide 2B1 is a high-affinity transporter for atorvastatin and is expressed in the human heart. Clin Pharmacol Ther 80:607–20
- Gui C, Hagenbuch B. (2008). Amino acid residues in transmembrane domain 10 of organic anion transporting polypeptide 1B3 are critical for cholecystokinin octapeptide transport. Biochemistry 47:9090–7
- Hagenbuch B, Gui C. (2008). Xenobiotic transporters of the human organic anion transporting polypeptides (OATP) family. Xenobiotica 38:778–801
- Hagenbuch B, Meier PJ. (2004). Organic anion transporting polypeptides of the OATP/SLC21 family: Phylogenetic classification as OATP/SLCO superfamily, new nomenclature and molecular/functional properties. Pflugers Arch 447:653–65
- Hediger MA, Romero MF, Peng JB, et al. (2004). The ABCs of solute carriers: Physiological, pathological and therapeutic implications of human membrane transport proteins. Introduction. Pflugers Arch 447:465–8
- Imanaga J, Kotegawa T, Imai H, et al. (2011). The effects of the SLCO2B1 c.1457C>T polymorphism and apple juice on the pharmacokinetics of fexofenadine and midazolam in humans. Pharmacogenet Genomics 21:84–93
- Ju WZ, Zhang J, Tan HS, et al. (2005). Determination of scutellarin in human plasma by LC-MS method and its clinical pharmacokinetics in Chinese healthy volunteers. Chin J Clin Pharmacol Ther 10:298–301
- König J, Seithel A, Gradhand U, Fromm MF. (2006). Pharmacogenomics of human OATP transporters. Naunyn Schmiedebergs Arch Pharmacol 372:432–43
- Kao TC, Wu CH, Yen GC. (2013). Glycyrrhizic acid and 18beta-glycyrrhetinic acid recover glucocorticoid resistance via PI3K-induced AP1, CRE and NFAT activation. Phytomedicine 20:295–302
- Kelly GS. (2011). Quercetin. Monograph. Altern Med Rev 16:172–94
- Kis O, Zastre JA, Ramaswamy M, Bendayan R. (2010). pH dependence of organic anion-transporting polypeptide 2B1 in Caco-2 cells: Potential role in antiretroviral drug oral bioavailability and drug–drug interactions. J Pharmacol Exp Ther 334:1009–22
- Klatt S, Fromm MF, Konig J. (2013). The influence of oral antidiabetic drugs on cellular drug uptake mediated by hepatic OATP family members. Basic Clin Pharmacol Toxicol 112:244–50
- Kobayashi D, Nozawa T, Imai K, et al. (2003). Involvement of human organic anion transporting polypeptide OATP-B (SLC21A9) in pH-dependent transport across intestinal apical membrane. J Pharmacol Exp Ther 306:703–8
- Kock K, Xie Y, Hawke RL, et al. (2013). Interaction of silymarin flavonolignans with organic anion-transporting polypeptides. Drug Metab Dispos 41:958–65
- Koenen A, Kroemer HK, Grube M, Meyer zu Schwabedissen HE. (2011). Current understanding of hepatic and intestinal OATP-mediated drug–drug interactions. Expert Rev Clin Pharmacol 4:729–42
- Krahenbuhl S, Hasler F, Frey BM, et al. (1994). Kinetics and dynamics of orally administered 18 beta-glycyrrhetinic acid in humans. J Clin Endocrinol Metab 78:581–5
- Kullak-Ublick GA, Ismair MG, Stieger B, et al. (2001). Organic anion-transporting polypeptide B (OATP-B) and its functional comparison with three other OATPs of human liver. Gastroenterology 120:525–33
- Lan T, Rao A, Haywood J, et al. (2009). Interaction of macrolide antibiotics with intestinally expressed human and rat organic anion-transporting polypeptides. Drug Metab Dispos 37:2375–82
- Li Z, Cheung FS, Zheng J, et al. (2014). Interaction of the bioactive flavonol, icariin, with the essential human solute carrier transporters. J Biochem Mol Toxicol 28:91–7
- Lin SP, Tsai SY, Hou YC, Chao PD. (2009). Glycyrrhizin and licorice significantly affect the pharmacokinetics of methotrexate in rats. J Agric Food Chem 57:1854–9
- Mandery K, Bujok K, Schmidt I, et al. (2010). Influence of the flavonoids apigenin, kaempferol, and quercetin on the function of organic anion transporting polypeptides 1A2 and 2B1. Biochem Pharmacol 80:1746–53
- Muller F, Fromm MF. (2011). Transporter-mediated drug–drug interactions. Pharmacogenomics 12:1017–37
- Nafisi S, Manouchehri F, Bonsaii M. (2012). Study on the interaction of glycyrrhizin and glycyrrhetinic acid with RNA. J Photochem Photobiol B 111:27–34
- Nozawa T, Imai K, Nezu J, et al. (2004). Functional characterization of pH-sensitive organic anion transporting polypeptide OATP-B in human. J Pharmacol Exp Ther 308:438–45
- Nozawa T, Nakajima M, Tamai I, et al. (2002). Genetic polymorphisms of human organic anion transporters OATP-C (SLC21A6) and OATP-B (SLC21A9): Allele frequencies in the Japanese population and functional analysis. J Pharmacol Exp Ther 302:804–13
- Ogura J, Koizumi T, Segawa M, et al. (2014). Quercetin-3-rhamnoglucoside (rutin) stimulates transport of organic anion compounds mediated by organic anion transporting polypeptide 2B1. Biopharm Drug Dispos 35:173–82
- Roth M, Obaidat A, Hagenbuch B. (2012). OATPs, OATs and OCTs: The organic anion and cation transporters of the SLCO and SLC22A gene superfamilies. Br J Pharmacol 165:1260–87
- Roth M, Timmermann BN, Hagenbuch B. (2011). Interactions of green tea catechins with organic anion-transporting polypeptides. Drug Metab Dispos 39:920–6
- Satoh H, Yamashita F, Tsujimoto M, et al. (2005). Citrus juices inhibit the function of human organic anion-transporting polypeptide OATP-B. Drug Metab Dispos 33:518–23
- Shirasaka Y, Shichiri M, Mori T, et al. (2013a). Major active components in grapefruit, orange, and apple juices responsible for OATP2B1-mediated drug interactions. J Pharm Sci 102:280–8
- Shirasaka Y, Shichiri M, Murata Y, et al. (2013b). Long-lasting inhibitory effect of apple and orange juices, but not grapefruit juice, on OATP2B1-mediated drug absorption. Drug Metab Dispos 41:615–21
- St-Pierre MV, Hagenbuch B, Ugele B, et al. (2002). Characterization of an organic anion-transporting polypeptide (OATP-B) in human placenta. J Clin Endocrinol Metab 87:1856–63
- Tamai I, Nozawa T, Koshida M, et al. (2001). Functional characterization of human organic anion transporting polypeptide B (OATP-B) in comparison with liver-specific OATP-C. Pharm Res 18:1262–9
- Tapaninen T, Neuvonen PJ, Niemi M. (2010). Grapefruit juice greatly reduces the plasma concentrations of the OATP2B1 and CYP3A4 substrate aliskiren. Clin Pharmacol Ther 88:339–42
- Tapaninen T, Neuvonen PJ, Niemi M. (2011). Orange and apple juice greatly reduce the plasma concentrations of the OATP2B1 substrate aliskiren. Br J Clin Pharmacol 71:718–26
- Woo HD, Kim J. (2013). Dietary flavonoid intake and risk of stomach and colorectal cancer. World J Gastroenterol 19:1011–19
- Xing JF, You HS, Dong YL, et al. (2011). Metabolic and pharmacokinetic studies of scutellarin in rat plasma, urine, and feces. Acta Pharmacol Sin 32:655–63