Abstract
Context: Garden cress [Lepidium sativum (Brassicaceae)] has been widely used to treat a number of ailments in traditional medicine. The pharmacological and preventive potential of Lepidium sativum, such as anti-inflammatory, antipyretic, antihypertensive, anti-ashthamatic, anticancer, and anti-oxidant, are well known.
Objective: The present investigation was designed to study the protective effects of chloroform extract of Lepidium sativum seed (LSE) against oxidative stress and cytotoxicity induced by hydrogen peroxide (H2O2) in human liver cells (HepG2).
Materials and methods: Cytotoxicity of LSE and H2O2 was identified by (3-(4,5-dimethylthiazol-2-yl)-2,5-diphenyl tetrazolium bromide (MTT), neutral red uptake (NRU) assays, and morphological changes in HepG2. The cells were pre-exposed to biologically safe concentrations (5–25 μg/ml) of LSE for 24 h, and then cytotoxic (0.25 mM) concentration of H2O2 was added. After 24 h of the exposures, cell viability by MTT, NRU assays, and morphological changes in HepG2 were evaluated. Further, protective effects of LSE on reactive oxygen species (ROS) generation, mitochondrial membrane potential (MMP), lipid peroxidation (LPO), and reduced glutathione (GSH) levels induced by H2O2 were studied.
Results: Pre-exposure of LSE significantly attenuated the loss of cell viability up to 48% at 25 µg/ml concentration against H2O2 (LD50 value = 2.5 mM). Results also showed that LSE at 25 µg/ml concentration significantly inhibited the induction of ROS generation (45%) and LPO (56%), and increases the MMP (55%) and GSH levels (46%).
Discussion and conclusion: The study suggests the cytoprotective effects of LSE against H2O2-induced toxicity in HepG2. The results also demonstrate the anti-oxidative nature of LSE.
Introduction
Oxidative stress plays a significant role in the etiology of variety of human diseases (Dhalla et al., Citation2000; Rahman, Citation2005). The role of oxidative stress in liver cells induced by various toxins is also well known (Rodeiro et al., Citation2008). Reports showed that overproduction of reactive oxygen species (ROS) plays a major role in the hepatocarcinoma (Lima et al., Citation2006; Zhang et al., Citation2011), which leads to cellular damage (Lin et al., Citation2007; Zhang et al., Citation2012). A number of in vitro studies have demonstrated that oxidative stress induced by chemical oxidants, such as hydrogen peroxide (H2O2), leads to cell death (Cai et al., Citation2008; Hwang & Yen, Citation2008; Kim et al., Citation2009; Siddiqui et al., Citation2011). H2O2 has also been reported to induce apoptotic changes, which subsequently lead to death in a variety of cell systems (Jung et al., Citation2006; Kanno et al., Citation2003; Sattayasai et al., Citation2013), including human liver cells (HepG2) (Alia et al., Citation2005; Chen et al., Citation2011). Therefore, we have selected H2O2 to induce the oxidative stress-mediated cytotoxicity in the HepG2.
Garden cress [Lepidium sativum (Brassicaceae)] has been widely used to treat a number of ailments in traditional system of medicine. Lepidium sativum is a fast-growing edible herb belonging to the family Brassicaceae (Cruciferae) or mustard family, and is being cultivated as culinary vegetable in North America, Europe, and all over Asia including India (Al-Sheddi et al., Citation2013; Nadkarni, Citation1976). The pharmacological and preventive properties of L. sativum, such as anti-oxidant (Yadav et al., Citation2010), anti-inflammatory, anticoagulant (Al-Yahya et al., Citation1994), antidiabetic (Eddoaks et al, Citation2005), antidiarrheal (Manohar et al., Citation2009), antihypertensive, diuretic (Mohamed et al., Citation2003; Umang et al., Citation2009), antirheumatic (Ahsan et al., Citation1989), anti-asthmatic (Paranjape & Mehta, Citation2006), chemoprotective (Fekadu et al, Citation2002), hypercholesterolemic (Al Hamedan, Citation2010), laxative (Najeeb et al., Citation2011), and fracture healing (Abdullah & Abdullah, Citation2007) are well documented. The seeds of L. sativum are also known to be useful in leprosy, skin, dysentery, diarrhea, splenomegal, and asthma diseases (Kirtikar & Basu, Citation2006). The phytoconstituent of L. sativum include carbohydrate, protein, fatty acid, riboflavin, niacin, flavonoids, isothiocynates glycoside, essential aromatic oils, and fatty oils (Al-Sheddi et al., Citation2013).
In view of the significant lack of the mechanism(s) defining the protective effects of L. sativum on oxidative stress and ROS generation in HepG2, the present study was designed to develop a model system to investigate the protective effects of chloroform extract of L. sativum seed (LSE) against H2O2-induced cytotoxicity in HepG2. The HepG2 are involved in the metabolism (Kim et al., Citation2011), and compose a good model to assess the toxicity or detoxification of various compounds against oxidative stress inducers (Farshori et al., Citation2013; Lima et al., Citation2006; Zhang et al., Citation2012).
Materials and methods
Chemicals and consumables
DMEM culture medium, antibiotics-atimycotic solution, fetal bovine serum (FBS), and trypsin were purchased from Invitrogen, Life Sciences, Grand Island, NY. Consumables and culture wares used in the study were procured from Nunc, Roskilde, Denmark. H2O2 and all other specified chemicals and reagents were purchased from Sigma Chemical Company Pvt. Ltd. St. Louis, MO.
Preparation of extract
The L. sativum seeds used in this study were obtained from the local market of Riyadh, Saudi Arabia in October 2013. The seeds were identified by Dr. Mohammad Atiqur Rahman, taxonomist of Medicinal, Aromatic and Poisonous Plants Research Center (MAPPRC), College of Pharmacy, King Saud University, Saudi Arabia and a specimen (#15967) was submitted in the herbarium of the King Saud University. The seeds were screened manually to remove bad ones. They were then dried to constant weight in an oven at 70°C, ground using mechanical grinder, put in air-tight containers, and stored in a desiccator. For the preparation of chloroform extract, the seeds were macerated in chloroform and then filtered. The procedure was repeated several times. The solvent was then evaporated using a rotary evaporator and the residue so obtained was formed as the chloroform extract.
Cell culture
HepG2, sourced from the American Type Culture Collection (Accession HB-8065, Rockville, MD) were cultured in DMEM, supplemented with 10% fetal bovine serum, 0.2% sodium bicarbonate, and antibiotic/antimycotic solution (100×, 1 ml/100 ml of medium). Cells were grown in 5% CO2 at 37°C in high humid atmosphere. Before the experiments, the viability of cells was assessed following the protocol of Siddiqui et al. (Citation2008). HepG2 showing more than 98% cell viability and passage number between 20 and 22 were used in this study.
Experimental design
To determine the biologically safe concentrations of LSE, HepG2 were exposed to 5–500 μg/ml of LSE and to determine the LD50 value of H2O2, the cells were exposed to 0.01–2 mM of H2O2 for 24 h. Further, to evaluate the protective effects of LSE on HepG2, the cells were pre-exposed to biologically safe concentrations (5, 10, and 25 μg/ml) of LSE. Then H2O2 (0.25 mM) was added and cells were incubated at 37°C for 24 h. After the exposures, percentage cell viability, morphological changes, ROS generation, mitochondrial membrane potential (MMP), lipid peroxidation (LPO), and reduced glutathione (GSH) were evaluated.
Drug solutions
The LSE was not completely soluble in culture medium; therefore, the stock solutions of extract were prepared in dimethylsulphoxide (DMSO) and diluted in culture medium to reach the desired concentrations. The concentration of DMSO in culture medium was not more than 0.1% and this medium was used as control. Hydrogen peroxide (H2O2) was freshly diluted in culture medium before adding to the cells.
Cytotoxicity screening of LSE extract and H2O2
The biologically safe or non-cytotoxic concentration of LSE and cytotoxicity of H2O2 was evaluated using 3-(4,5-dimethylthiazol-2-yl)-2,5-diphenyl tetrazolium bromide (MTT), neutral red uptake (NRU), and cellular morphology assays. For the cytotoxicity assessment, HepG2 were exposed to various concentrations (5–500 μg/ml) of LSE and H2O2 (0.01–2 mM) for 24 h. The cellular morphological analysis was performed by phase contrast-inverted microscope.
MTT assay
Percentage cell viability was assessed using the MTT assay as described (Siddiqui et al., Citation2008). Briefly, HepG2 (1 × 104) were allowed to adhere for 24 h CO2 incubator at 37°C in 96-well culture plates. After 24 h exposure of HepG2 with increasing concentrations (5–500 μg/ml) of LSE and H2O2 (0.01–2 mM) for 24 h, MTT (5 mg/ml of stock in PBS) was added (10 μl/well in 100 μl of cell suspension), and plates were incubated for 4 h. The supernatant was discarded and 200 μl of DMSO was added to each well and mixed gently. The developed color was read at 550 nm using multiwell microplate reader (Thermo Scientific, Waltham, MA). Untreated sets were also run under identical conditions and served as control.
NRU assay
NRU assay was carried out following the protocol described by Siddiqui et al. (Citation2010). Briefly, after 24 h exposure of HepG2 with increasing concentrations (5–500 μg/ml) of LSE and H2O2 (0.01–2 mM) for 24 h, the medium was aspirated and cells were washed twice with PBS, and incubated for 3 h in a medium supplemented with neutral red (50 μg/ml). The medium was washed off rapidly with a solution containing 0.5% formaldehyde and 1% calcium chloride. Cells were subjected to further incubation of 20 min at 37°C in a mixture of acetic acid (1%) and ethanol (50%) to extract the dye. The plates were read at 540 nm using multiwell microplate reader (Thermo Scientific, Waltham, MA). The values were compared with the control sets run under identical conditions.
Morphological analysis
Morphological changes in HepG2 exposed to increasing concentrations of LSE (5–500 μg/ml) and H2O2 (0.01–2 mM) for 24 h were observed by phase contrast inverted microscope (Olympus, Center Valley, PA) attached with automatic image analysis software. Further, to observe the protective effects of LSE on cellular morphology, HepG2 were treated with biologically safe concentrations (5, 10, and 25 μg/ml) of LSE before exposure to cytotoxic concentration of H2O2 (0.25 mM) for 24 h.
ROS generation
The production of intracellular ROS was measured using 2,7-dichlorofluorescin diacetate (DCFH-DA) as described by Wang and Joseph (Citation1999) with slight modifications. In brief, after the exposure of HepG2 with LSE and H2O2, the cells were washed twice with PBS. The cells were then exposed to 20 μM of DCFH-DA fluorescent dye for 1 h at 37°C in dark. The fluorescence intensity of specific dye was measured at 485 nm excitation and 530 nm emission wavelengths using spectrofluorometer (Fluoroskan, Thermo Scientific, Waltham, MA).
Mitochondrial membrane potential
MMP was measured following the protocol of Zhang et al. (Citation2011). In brief, control and treated cells were washed twice with PBS. The cells were further treated with 10 µg/ml of Rhodamine-123 fluorescent dye for 1 h at 37°C in dark. The fluorescence intensity of Rhodamine-123 was measured at 485 nm excitation and 530 nm emission wavelengths using spectrofluorometer.
Lipid peroxidation
LPO was performed using thiobarbituric acid-reactive substances (TBARS) protocol (Buege & Aust, Citation1978). Briefly, after the exposure of HepG2 with LSE and H2O2, HepG2 were collected by centrifugation and sonicated in ice cold potassium chloride (1.15%) and centrifuged again for 10 min at 3000 × g. The resulting supernatant (1 ml) was collected and 2 ml of thiobarbituric acid (TBA) reagent (15% TCA, 0.7% TBA, and 0.25N HCl) was added. The solution was heated at a temperature of 100°C for 15 min in a boiling bath. The sample was then placed at a cold temperature and centrifuged at 1000 × g for 10 min. The absorbance of the supernatant was measured at 535 nm.
GSH level
Intracellular reduced GSH level was estimated as described by Chandra et al. (Citation2002) with some modifications. Briefly, HepG2 exposed to LSE and H2O2 were collected by centrifugation and the cellular proteins were precipitated by incubating 1 ml sonicated cell suspension with 10% TCA (1 ml) on ice for 1 h followed by centrifugation at 3000 rpm for 10 min. The supernatant was then added to 2 ml buffer (0.4 M Tris and 0.02 M EDTA; pH 8.9) and 0.01 M of 5,5′-dithionitrobenzoic acid (DTNB) to reach a final volume of 3 ml. The tubes were incubated for 10 min at 37°C in a shaking water bath. The absorbance of yellow color developed was read at 412 nm.
Statistical analysis
Results were expressed as mean ± SE of at least three independent experiments (each in triplicate). Statistical analysis was performed using one-way ANOVA using Dunnett’s post hoc test employed to compare the values between control and treated groups. The values showing p < 0.05 were considered as statistically significant.
Results
Cytotoxicity assessments of LSE and H2O2
The cytotoxicity of LSE was assessed using MTT assay, NRU assay, and cellular morphology, after exposing the HepG2 to various concentrations of LSE and H2O2. Our results showed that LSE at 5, 10, and 25 μg/ml concentrations did not cause any significant effect on cell viability of HepG2 ( and ). Therefore, the concentrations 5, 10, and 25 μg/ml of LSE were chosen to study the protective effects against H2O2-induced toxicity in HepG2. Further, based on the LD50 value obtained, 0.25 mM of H2O2 was used to induce the toxicity in further experiments ().
Figure 1. Cytotoxicity assessment by (A) MTT assay and (B) NRU assay in HepG2 following the exposure of various concentrations of LSE for 24 h. Values are mean ± SE of three independent experiments. *p < 0.05, **p < 0.01 versus control.
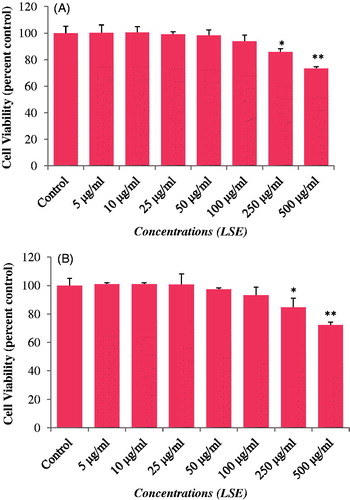
Protective effects of LSE against H2O2-induced cell death
MTT and NRU assays
The protective potential of LSE in HepG2 observed by MTT and NRU assays is presented in . A significant (p < 0.01) reduction in percentage cell viability was observed in HepG2 following the exposure of H2O2 (0.25 mM) for 24 h by MTT assay (), and NRU assay (). HepG2 pre-treated with LSE at 5, 10, and 25 μg/ml for 24 h significantly attenuated the H2O2 induced loss of cell viability in a concentration-dependent manner. An increase of 45% in the cell viability of HepG2 was recorded at 25 μg/ml of LSE (). A similar kind of concentration-dependent increase in the cell viability was also observed by NRU assay in LSE pre-exposed HepG2. An increase of 48% in cell viability of HepG2 was recorded at 25 μg/ml of LSE ().
Morphological changes
The alterations in the morphology of HepG2 on exposure of LSE and H2O2 are shown in . Cells exposed to 0.25 mM of H2O2 reduced the normal morphology of HepG2, and cell adhesion capacity as compared with control. Most of the cells exposed to H2O2 lost their typical morphology and appeared smaller in size (). HepG2 exposed to increasing concentrations of LSE for 24 h prior to H2O2 exposure significantly restore their original morphology in a concentration-dependent manner ().
Figure 5. Alterations in the morphology of HepG2. Cells were pre-exposed with LSE for 24 h, then hydrogen peroxide (H2O2) for 24 h. Image were taken using phase contrast-inverted microscope at 20× magnification. (A) Control, (B) H2O2 (0.25 mM), (C) LSE (5 μg/ml) + H2O2 (0.25 mM), (D) LSE (10 μg/ml) + H2O2 (0.25 mM), and (E) LSE (25 μg/ml) + H2O2 (0.25 mM).
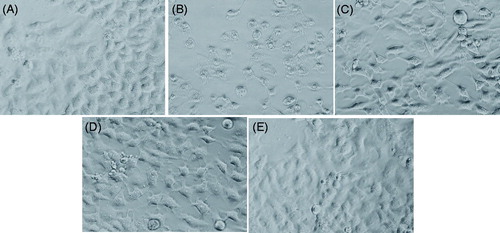
Protective effects of LSE on ROS generation induced by H2O2
The results of ROS generation in HepG2 exposed to H2O2 and various concentrations of LSE are presented in . Exposure of HepG2 with H2O2 at 0.25 mM for 24 h resulted in an increase of 154 ± 5.6% of increase (p < 0.01) in the production of ROS. Pre-treatment of cells with LSE at 5, 10, and 25 μg/ml concentration significantly reduced the levels of H2O2-induced ROS production in HepG2 ().
Figure 6. Protective potential of LSE on (A) reactive oxygen species (ROS) generation; (B) mitochondrial membrane potential (MMP); (C) lipid peroxidation (LPO); (D) glutathione (GSH) depletion in HepG2 following the exposure of different concentrations of LSE for 24 h prior to the addition of H2O2 for 24 h. All values represent the mean ± SE. *p < 0.05, **p < 0.01 versus H2O2 exposure.
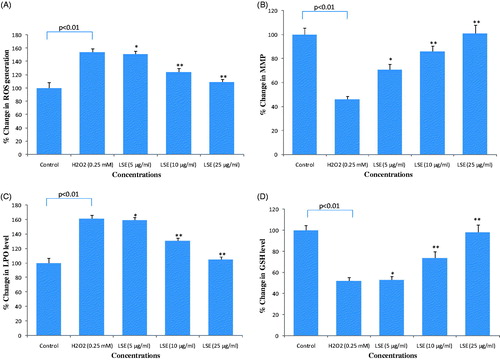
Protective effects of LSE on MMP
The intensity of mitochondrial membrane potential (MMP) in HepG2 exposed to H2O2 and various concentrations of LSE is presented in . Results showed that HepG2 exposed to H2O2 (0.25 mM) for 24 h resulted in 46 ± 2.6% reduction (p < 0.01) in the level of MMP. Pre-treatment of cells with LSE at 5, 10, and 25 μg/ml concentrations prior to H2O2 treatment significantly increased the intensity of MMP in HepG2 ().
Protective effects of LSE on LPO
Protective potential of various concentrations of LSE on H2O2 induced lipid peroxidation in HepG2 is summarized in . As shown in figure, exposure of H2O2 resulted in a significant increase of 61% (p < 0.01) in LPO as compared with control cells. HepG2 pre-treated with LSE at 5, 10, and 25 μg/ml concentration for 24 h prior to H2O2 treatment significantly (p < 0.01) reduced the LPO ().
Protective effects of LSE on GSH level
Protective potential of LSE on H2O2-induced depletion in GSH level is summarized in . As shown in figure, HepG2 exposed with 0.25 mM of H2O2 significantly reduced the GSH level by 48% (p < 0.01) as compared with control. Results also showed that LSE at 5, 10, and 25 μg/ml significantly prevented (p < 0.01) the depletion of GSH levels caused H2O2 by in HepG2 ().
Discussion
Oxidative stress has been proven to play an important role in the progression of a number of human diseases (Dhalla et al., Citation2000; Rahman, Citation2005). H2O2 is known to induce oxidative stress in a variety of cells (Kumar et al., Citation2010; Siddiqui et al., Citation2011; Sattayasai et al., Citation2013). There is a plethora of evidence documenting the essential involvement of oxidative stress-induced damages and increased levels of H2O2 in a variety of diseases (Spencer et al., Citation2009; Yamashita et al., Citation2008). Since oxidative stress seems to be involved in many diseases, the administration of anti-oxidants may be useful in the prevention and treatment of these diseases (Sekler et al., Citation2008). Currently, there is an increasing interest in naturally derived bioactive compounds with potential cytoprotective effects against oxidative stress induced cell death (Ali et al., Citation2014; Jaydeokar et al., Citation2014). Lepidium sativum has been widely used to treat a number of diseases in conventional medical system. It has been reported that seed extracts of L. sativum possess anti-asthmatic (Paranjape & Mehta, Citation2006) and bronchodilatory (Mali et al., Citation2008) activities, and are also being used in the treatment of bronchial asthma (Archana & Mehta, Citation2006). However, protective effects of L. sativum on ROS generation and oxidative stress induced by H2O2 in HepG2 have not been explored so far. Therefore, in order to explore the protective potential of LSE against H2O2-induced oxidative stress and ROS generation, the present study was designed. Our results demonstrated that LSE exhibited significant cytoprotective effects against H2O2-induced cell death in HepG2. Based on the LD50 value obtained by MTT and NRU assays, 0.25 mM of H2O2 was selected to induce oxidative stress-mediated cytotoxicity in HepG2. The results of this study are consistent with the previous reports of concentration-dependent cytotoxicity of H2O2 (Kumar et al., Citation2010; Okello et al., Citation2011; Siddiqui et al., Citation2011). Response of H2O2 in various cells has been reported in similar fashion in the literature and we believe that the same kind of oxidative stress-related events have also been induced in HepG2. Our results showed that pre-exposure of LSE significantly increased the cell viability of H2O2-exposed HepG2. These data are comparable with previous studies reporting that crude extracts from natural products exhibited significant cyto-protection against the H2O2 in PC12 and SK-N-SH cells (Kumar et al., Citation2010; Sattayasai et al., Citation2013) and in liver cells against other toxicants (Alia et al., Citation2006; Goya et al., Citation2007; Martin et al., Citation2008; Verma et al., Citation2013).
The induction of intracellular ROS generation in HepG2 indicates that H2O2 can cause oxidative stress. In this study, the restoration of the intra-cellular ROS level by pre-treatment with LSE showed the protective effects of various concentrations of LSE against H2O2-induced ROS generation in HepG2. Our results are in agreement with the previous reports showing that the administration of natural products suppress the increased level of intra-cellular ROS generation (Farshori et al., Citation2013; Molina et al., Citation2003; Sattayasai et al., Citation2013; You et al., Citation2010). It is known that apoptosis-signaling cascades can be initiated by mitochondria impairment (Taveira et al., Citation2014). It has also been suggested by some authors that the mitochondria plays an important role in cell protection and could be used as an essential therapeutic target (Swerdlow et al., Citation2010). In this study, we found that the administration of LSE can protect mitochondrial membrane dysfunction in H2O2 exposed HepG2. As shown in , the LSE revealed potential capacity by preserving the mitochondrial membrane potential (MMP).
Lipid peroxidation is one of the mechanisms of oxidative stress and cell death (Schmidtmann et al., Citation1991; Tribble et al., Citation1897). The increase in the lipid peroxidation caused by H2O2 has been reported (Siddique et al., Citation2012) and our results are in well concurrence with these findings. This increase in lipid peroxidation in HepG2 by H2O2 may be due to an enhancement in the hepatic MDA from the peroxidation of polyunsaturated fatty acids. Our results also showed that the H2O2-induced increase in the level of lipid peroxidation was significantly decreased when cells were pre-exposed to various concentrations of LSE. Glutathione (GSH) is a main non-enzymatic anti-oxidant defense system within the cells and plays an important role in the protection against oxidative stress (Scharf et al., Citation2003). As shown in , H2O2 reduced the GSH level to 48% in HepG2 as compared with untreated control. However, the pre-treatment of HepG2 with LSE (5–25 μg/ml) significantly restored the decrease in the GSH level caused by H2O2. It is also known that the glutathione peroxidase catalyses GSH oxidation to GSSG at the expense of H2O2 and glutathione reductase recycles oxidized GSH back to reduced GSH (Goya et al., Citation2007; Ursini et al., Citation1995). Therefore, it could be inferred that the LSE pre-exposed HepG2 quenches the intracellular damaging peroxide and recovers the concentration of reduced GSH. The significant revival in the level of GSH clearly indicates a positive response of LSE in cell defense system against the H2O2 oxidative insult.
Conclusion
Our results demonstrate that LSE has the ability to protect HepG2 against the H2O2-induced cytotoxicity by modulating ROS generation and lipid peroxidation, as well as reducing MMP and GSH levels. The results from this study produced more insight on the potential biological activities of LSE and suggested that LSE could be a source of an anti-oxidant. However, detailed future studies on the mechanism(s) of detoxification of H2O2 by which LSE exert cytoprotective effects in HepG2 are needed.
Declaration of interest
The authors report that they have no conflict of interest. This work was supported by NSTIP Strategic Technologies Program number (12-MED2491-02) in the Kingdom of Saudi Arabia.
References
- Abdullah H, Abdullah J. (2007). The effects of Lepidium sativum seeds on fracture-induced healing in rabbits. Med Gen Med 9:23–9
- Ahsan SK, Tariq M, Ageel M, et al. (1989). Studies on some herbal drugs used in fracture healing. Int J Crude Drug Res 27:235–9
- Al Hamedan WA. (2010). Protective effect of Lepidium sativum L. seeds powder and extract on hypercholesterolemic rats. J Am Sci 6:873–9
- Ali M, Khan SA, Chang PS, et al. (2014). Habb-e-Asgand, polyherbal Unani formulation, protects liver and antioxidative enzymes against paracetamol induced hepatotoxicity. Pharm Biol 52:506–15
- Alia M, Ramos S, Mateos R, et al. (2005). Response of the antioxidant defense system to tert-butyl hydroperoxide and hydrogen peroxide in a human hepatoma cell line (HepG2). J Biochem Mol Toxicol 19:119–28
- Alia M, Ramos S, Mateos R, et al. (2006). Quercetin protects human hepatoma cell line (HepG2) against oxidative stress induced by tertbutyl hydroperoxide. Toxicol Appl Pharm 212:110–18
- Al-Sheddi ES, Farshori NN, Al-Oqail MM, et al. (2013). Evaluation of cytotoxicity and oxidative stress induced by alcoholic extract and oil of Lepidium sativum seeds in human liver cell line HepG2. Afr J Biotech 12:3854–63
- Al-Yahya MA, Mossa JS, Ageel AM, Rafatullah S. (1994). Pharmacological and safety evaluation studies on Lepidium sativum L., seeds. Phytomedicine 1:155–9
- Archana PN, Mehta AA. (2006). A study on clinical efficacy of Lepidium sativum seeds in treatment of bronchial asthma. Iran J Pharmacol Ther 5:55–9
- Buege JA, Aust SD. (1978). Microsomal lipid peroxidation. Methods Enzymol 52:302–10
- Cai L, Wang H, Li Q, et al. (2008). Salidroside inhibits H2O2-induced apoptosis in PC12 cells by preventing cytochrome c release and inactivating of caspase cascade. Acta Biochim Biophys Sin (Shanghai) 40:796–802
- Chandra D, Ramana KV, Wang L, et al. (2002). Inhibition of fiber cell globulization and hyperglycemia-induced lens opacification by aminopeptidase inhibitor bestatin. Invest Ophthalmol Vis Sci 43:2285–92
- Chen X, Zhong Z, Xu Z, et al. (2011). No protective effect of curcumin on hydrogen peroxide-induced cytotoxicity in HepG2 cells. Pharmacol Rep 63:724–32
- Dhalla NS, Temsah RM, Netticadan T. (2000). Role of oxidative stress in cardiovascular diseases. J Hypertens 18:655–73
- Eddoaks M, Maghrani M, Zeggwagh NA, Michel JB. (2005). Study of the hypoglycemic activity of Lepidium sativum L. aqueous extract in normal and diabetic rats. J Ethnopharmacol 97:391–5
- Fekadu K, Sylvie R, Mariauhl WH, et al. (2002). Chemoprotective effects of garden cress (Lepidium sativum L.) and colonic preneoplastic 2-amino-3-methyl-imidazole (4,5-f) quinoline (IQ)-induced genotoxic effects and colonic preneoplastic lesions. Carcinogeneis 23:1155–61
- Goya L, Delgado-Andrade C, Rufian-Henares JA, et al. (2007). Effect of coffee Melanoidin on human hepatoma HepG2 cells. Protection against oxidative stress induced by tert-butylhydroperoxide. Mol Nutr Food Res 51:536–45
- Hwang SL, Yen GC. (2008). Neuroprotective effects of the citrus flavanones against H2O2-induced cytotoxicity in PC12 cells. J Agric Food Chem 56:859–64
- Jaydeokar AV, Bandawane DD, Bibave KH, Patil TV. (2014). Hepatoprotective potential of Cassia auriculata roots on ethanol and antitubercular drug-induced hepatotoxicity in experimental models. Pharm Biol 52:344–55
- Jung CH, Jun CY, Lee S, et al. (2006). Rhus verniciflua stokes extract: Radical scavenging activities and protective effects on H2O2-induced cytotoxicity in macrophage RAW 264.7 cell lines. Biol Pharm Bull 29:1603–7
- Kanno SI, Shouji A, Asou K, Ishikawa M. (2003). Effects of naringin on hydrogen peroxide-induced cytotoxicity and apoptosis in P388 cells. J Pharm Sci 92:166–70
- Kim JH, Kim D, Kim J, Hwang JK. (2011). Euchresta horsfieldii Benn. activates peroxisome proliferator-activated receptor α and regulates expression of genes involved in fatty acid metabolism in human HepG2 cells. J Ethnopharmacol 133:244–7
- Kim JS, Ha TY, Ahn J, et al. (2009). Pterostilbene from vitis coignetiae protect H2O2-induced inhibition of gap junctional intercellular communication in rat liver cell line. Food Chem Toxicol 47:404–9
- Kirtikar KR, Basu BD. (2006). Indian Medicinal Plants. Allahabad: Popular Prakashan, 174–5
- Kumar S, Seal CJ, Howes MJR, et al. (2010). In vitro protective effects of Withania somnifera (L.) dunal root extract against hydrogen peroxide and b-amyloid(1–42)-induced cytotoxicity in differentiated PC12 cells. Phytother Res 24:1567–74
- Lima CF, Fernandes-Ferreira M, Pereira-Wilson C. (2006). Phenolic compounds protect HepG2 cells from oxidative damage: Relevance of glutathione levels. Life Sci 79:2056–68
- Lin PH, Lin CH, Huang CC, et al. (2007). 2,3,7,8-Tetrachlorodibenzo-p-dioxin (TCDD) induces oxidative stress, DNA strand breaks, and poly(ADP-ribose) polymerase-1 activation in human breast carcinoma cell lines. Toxicol Lett 172:146–58
- Mali RG, Mahajan SG, Mehta AA. (2008). Studies on bronchodilatory effect of Lepidium sativum against allergen induced bronchospasm in guinea pigs. Pharmacogn Mag 4:189–92
- Manohar D, Shylaja H, Viswanatha GL, Rajesh S. (2009). Antidiarrheal activity of methanolic extracts of Lepidium sativum in rodent. J Nat Remidies 9:197–201
- Martin MA, Ramos S, Mateos R, et al. (2008). Protection of human HepG2 cells against oxidative stress by cocoa phenolic extract. J Agric Food Chem 56:7765–72
- Mohamed M, Naoufel A, Jean BM, Mohamed E. (2003). Antihypertensive effects of Lepidium sativum L. in spontaneously hypertensive rats. J Ethanophamacol 100:193–7
- Molina MF, Sanchez-Reus I, Iglesias I, Benedi J. (2003). Quercetin, a flavonoid antioxidant, prevents and protects against ethanol-induced oxidative stress in mouse liver. Biol Pharm Bull 26:1398–402
- Nadkarni KM. (1976). Indian Materia Medica. Bombay, India: Popular Prakashan, 1001
- Najeeb UR, Malik HM, Khalid MA, Anwarul HG. (2011). Prokinetic and laxative activities of Lepidium sativum seed extract with species and tissue selective gut stimulatory actions. J Ethanopharmacol 134:878–83
- Okello EJ, McDougall GJ, Kumar S, Seal CJ. (2011). In vitro protective effects of colon-available extract of Camellia sinensis (tea) against hydrogen peroxide and beta-amyloid (Aβ(1–42)) induced cytotoxicity in differentiated PC12 cells. Phytomedicine 18:691–6
- Paranjape AN, Mehta AA. (2006). A study on clinical efficacy of Lepidium sativum seeds in treatment of bronchial asthma. Iran J Pharmacol Ther 5:55–9
- Rahman I. (2005). The role of oxidative stress in the pathogenesis of COPD: Implications for therapy. Treat Respir Med 4:175–200
- Rodeiro I, Donato MT, Martínez I, et al. (2008). Potential hepatoprotective effects of new Cuban natural products in rat hepatocytes culture. Toxicol In Vitro 22:1242–9
- Sattayasai J, Chaonapan P, Arkaravichie T, et al. (2013). Protective effects of Mangosteen extract on H2O2-induced cytotoxicity in SK-N-SH cells and scopolamine-induced memory impairment in mice. PloS One 8:e85053
- Scharf G, Prustomersky S, Knasmuller S, et al. (2003). Enhancement of glutathione and glutamylcysteine synthetase, the rate limiting enzyme of glutathione synthesis, by chemoprotective plant-derived food and beverage components in the human hepatoma cell line HepG2. Nutr Cancer 45:74–83
- Schmidtmann S, Muller M, von Baehr R, Precht K. (1991). Changes of antioxidative homeostasis in patients on chronic haemodialysis. Nephrol Dial Transplant 6:71–4
- Sekler A, Jiménez JM, Rojo L, et al. (2008). Cognitive impairment and Alzheimer's disease: Links with oxidative stress and cholesterol metabolism. Neuropsychiatr Dis Treat 4:715–22
- Siddique YH, Ara G, Afzal M. (2012). Estimation of lipid peroxidation induced by hydrogen peroxide in cultured human lymphocytes. Dose–Response 10:1–10
- Siddiqui MA, Kashyap MP, Kumar V, et al. (2010). Protective potential of trans-resveratrol against 4-hydroxynonenal induced damage in PC12 cells. Toxicol In Vitro 24:1592–8
- Siddiqui MA, Kashyap MP, Kumar V, et al. (2011). Differential protection of pre-, co-and post-treatment of curcumin against hydrogen peroxide in PC12 cells. Hum Exp Toxicol 30:192–8
- Siddiqui MA, Singh G, Kashyap MP, et al. (2008). Influence of cytotoxic doses of 4-hydroxynonenal on selected neurotransmitter receptors in PC-12 cells. Toxicol In Vitro 22:1681–8
- Spencer WA, Lehmler HJ, Robertson LW, Gupta RC. (2009). Oxidative DNA adducts following Cu (2þ)-mediated activation of dihydroxy PCBs: Role of reactive oxygen species. Free Radic Biol Med 46:1346–52
- Swerdlow R, Burns J, Khan S. (2010). The Alzheimer's disease mitochondrial cascade hypothesis. J Alzheimer's Dis 20:265–79
- Taveira M, Sousa C, Valentão P, et al. (2014). Neuroprotective effect of steroidal alkaloids on glutamate-induced toxicity by preserving mitochondrial membrane potential and reducing oxidative stress. J Steroid Biochem Mol Biol 140:106–15
- Tribble DL, Tak YA, Dean P, Jones MD. (1987). The pathophysiological significance of lipid peroxidation in oxidative cell injury. Hepatology 7:77–386
- Umang P, Mukul K, Undale V, Ashok B. (2009). Evaluation of diuretic activity of aqueous and methanolic extracts of Lepidium sativum garden cress (Cruciferae) in rats. Trop J Pharm Res 8:215–19
- Ursini F, Maiorino M, Brigelius-Floh R, et al. (1995). Diversity of glutathione peroxidases. Methods Enzymol 252:38–114
- Verma S, Bahorun T, Singh RK, et al. (2013). Effect of Aegle marmelos leaf extract on N-methyl N-nitrosourea-induced hepatocarcinogensis in Balb/c mice. Pharm Biol 51:1272–81
- Wang H, Joseph JA. (1999). Quantifying cellular oxidative stress by dichlorofluorescin assay using microplate reader. Free Radic Biol Med 27:612–16
- Yadav YC, Srivastav DN, Seth AK, et al. (2010). In vivo antioxidant potential of Lepidium sativum L. seeds in albino rats using cisplatin induced nephrotoxicity. Interact J Phytomed 2:292–8
- Yamashita A, Nijo N, Pospı’sil P, et al. (2008). Quality control of photosystem II: Reactive oxygen species are responsible for the damage to photosystem II under moderate heat stress. J Biol Chem 283:28380–91
- You Y, Yoo S, Yoon HG, et al. (2010). In vitro and in vivo hepatoprotective effects of the aqueous extract from Taraxacum officinale (dandelion) root against alcohol-induced oxidative stress. Food Chem Toxicol 48:1632–7
- Zhang CL, Zeng T, Zhao XL, et al. (2012). Protective effects of garlic oil on hepatocarcinoma induced by N-nitrosodiethylamine in rats. Int J Biol Sci 8:363–74
- Zhang Y, Lijie J, Liping J, et al. (2011). Possible involvement of oxidative stress in potassium bromate-induced genotoxicity in human HepG2 cells. Chem Biol Interact 189:186–91