Abstract
Context: Antibiotic resistance in humans is a major concern. Drugs that target traditional sites and pathways are becoming obsolete; thus, compounds affecting novel targets are needed. Screening lichen metabolites for antimicrobials has yielded promising antimicrobial compounds, yet their mode of action is poorly understood. Letharia vulpina (L.) Hue (Parmeliaceae) has traditionally been used to poison predators, and treat stomach disorders; more recently L. vulpina extracts have demonstrated promising antimicrobial properties.
Objective: This study investigates the mode of action of L. vulpina acetone extract against a methicillin-resistant Staphylococcus aureus (MRSA).
Material and methods: We treated MRSA with L. vulpina extracts at 1×, 5×, and 10 × MIC values (MIC = 31.25 µg/ml) for 24 h and optical density (OD660) was measured over time to determine bacteriolytic activity; counted colony forming units (CFUs) to determine time kill dynamics; the propidium iodide (PI) assay and transmission electron microscopy were used to assess membrane-damage potential, and thin-layer chromatography was used to identify secondary compounds.
Results: Bacteriolytic assays showed that L. vulpina extracts, containing only vulpinic acid, do not cause cell lysis, even at 10 × MIC values but there was 92% reduction in bacterial CFUs when treated with increased concentrations of lichen extracts over 24 h at 4 h intervals. Our data indicate that the L. vulpina extract compromises membrane integrity of the MRSA isolate and disrupts cell division processes.
Discussion and conclusion: Based on this study, detailed examination of acetone extracts of L. vulpina as well as pure extracts of vulpinic acid as potential antibacterial compounds merit further study.
Introduction
Antibiotics are microbial-derived compounds that act specifically to disable vital cellular functions like cell wall synthesis, cell membrane integrity, and protein synthesis (Mobashery & Azucena, Citation2002; Wilson et al., Citation2011). They are produced by a wide variety of microorganisms in order to limit competition. Their potential for treating human infections was realized in the early 1940s thanks to the accidental discovery of penicillin, produced by the Penicillium fungus (Collignon, Citation2002; Wilson et al., Citation2011). The subsequent development of commercial, broad-spectrum antibiotics ushered in an era of unprecedented disease-survival rates and increased life-expectancy (Wilson et al., Citation2011). Recently, however, populations of pathogenic (disease-causing) microorganisms have evolved resistance to many commonly used antibiotics. The overuse and frequent misuse of these “miracle drugs” have strongly selected for drug-resistant strains, as well as given place to greater incidence of opportunistic and nosocomial infections.
One example of a common antibiotic-resistant human pathogen is methicillin-resistant Staphylococcus aureus (MRSA). MRSA has evolved resistance to a broad array of β-lactam antibiotics, making it difficult to treat (Taylor, Citation2013). MRSA is thought to have acquired the mecA gene through horizontal gene transfer. This gene confers a mutated version of the bacterial protein targeted by β-lactam antibiotics. Although there are still antibiotics effective against MRSA, their range of effectiveness, spectrum of side effects, and limited applicability make the need for newer therapeutic options critical (Liapikou & Torres, Citation2013; Taylor, Citation2013).
The discovery of new antibacterial drugs has diminished in recent years. The rapid development of drug-resistant isolates has severely impacted a drug's long-term potential to return a profit (Leeb, Citation2004). Hence, it is necessary to investigate and develop new classes of antimicrobials that are either opaque to pathogen resistance or express a new mode of action, thereby increasing the potential lifetime of the antibacterial agent (Kokubun et al., Citation2007).
Natural products derived from plants, fungi, marine organisms, and microorganisms have served as a rich source of novel biologically active compounds. More than 300 new natural metabolites with antimicrobial activities were reported between 2000 and 2008 (Saleem et al., Citation2010), but there are still many compounds that deserve more thorough investigation. Hence, there is a growing interest in phytomedicine, and many medicinally important plants are being investigated as potential sources of new antimicrobial agents (Gupta et al., Citation2012).
Lichens are symbiotic systems consisting of a fungus and an alga and/or a cyanobacterium engaged in a complex symbiotic relationship. Lichens represent one of the more promising potential reservoirs for low molecular weight secondary compounds with more than 1000 different chemicals reported for lichens and their cultured mycobionts (Molnar & Farkas, Citation2010). The antimicrobial properties of lichen compounds have been well documented (Pompilio et al., Citation2013; Shrestha & St. Clair, Citation2013b; Stojanovic et al., Citation2012). Several compounds with potent antibacterial effect have been isolated from various lichen species. Among them, usnic acid is the best-known antimicrobial compound (Araújo et al., Citation2015; Cocchietto et al., Citation2002). Usnic acid has also been used as a topical antibacterial agent for human skin diseases in many European countries (Odds et al., Citation2001). However, information regarding the mode of action of these compounds against pathogenic bacteria is very limited. To our knowledge, there is only one published report on the mechanism of action of lichen-derived compounds (Gupta et al., Citation2012) which reports the membrane-damaging potential of usnic acid in the treatment of MRSA.
Recently, two studies (Shrestha & St. Clair, Citation2013a; Shrestha et al., Citation2014) examined the antibacterial properties of acetone and methanol extracts from 36 species of lichens against four different pathogenic bacteria. As a follow up to these earlier studies, acetone extracts from Letharia vulpina (L.) Hue (Parmeliaceae) were tested against MRSA isolates to determine the mode of action. This lichen species was selected because it has been found to be effective in inhibiting the growth of four pathogenic bacteria in our previous study and because it contains only vulpinic acid. Letharia vulpina has traditionally been used to address a variety of issues. For example, indigenous people of Northern California used this species to treat stomach disorders (Malhotra et al., Citation2007). Likewise, some Plateau Indian tribes used it as a poultice for swelling, bruises, sores, and boils (Hunn, Citation1990). In addition, L. vulpine-laced meat was also used in Scandinavia to poison wolves and foxes (Galun, Citation1988). The authors have shown in another study that extracts of L. vulpina are also capable of inhibiting the growth of Escherichia coli, Pseudomonas aeruginosa, Staphylococcus aureus, and MRSA. In this study, we specifically examined the effect of L. vulpina extracts on bacteriolysis, time kill dynamics, and membrane damaging potential.
Materials and methods
Bacterial strain
The MRSA COL strain used in this study was kindly provided by Dr. Brian Wilkinson, University of Illinois.
Lichen material
In June of 2010, samples of L. vulpina were collected from the vicinity of Alpine Creek Trailhead in the Sawtooth National Recreational Area, Blaine County, Idaho (43 °53′53.04″ N, 114 °54′25.62″ W). A voucher specimen has been deposited in the Herbarium of Non-vascular cryptogams, at Brigham Young University, Provo, UT. An acetone extract of L. vulpina was used in this study. Procedures for the extraction and identification of lichen compounds have been previously published (Shrestha et al., Citation2014).
Minimum inhibitory concentration (MIC) assays
The MIC value for L. vulpina extracts was 31.25 µg/ml. This result was initially reported in Shrestha et al. (Citation2014). In the current study, we tested three different concentrations (1 × MIC, 5 × MIC, and 10 × MIC) of the same extract used in our previous study in an effort to determine the mode of action of the extract.
Bacteriolysis
The effects of the L. vulpina extract on cell lysis were evaluated by measuring the OD660 value of treated bacteria at various points, in accordance with the method described by Isnansetyo and Kamei (Citation2003) with a slight modification. Briefly, overnight cultures of MRSA were prepared by inoculating 10 ml of Müller Hinton Broth (Sigma Aldrich, St. Louis, MO) with a single MRSA colony, and incubating the culture at 37 °C with shaking (200 rpm). Cultures were then diluted with Müller Hinton broth to an OD660 value of approximately 0.1. The various bacterial suspensions were then treated separately with three different concentrations (1 × MIC, 5 × MIC, and 10 × MIC) of the L. vulpina extract. Immediately following the addition of the extracts, samples were taken and OD660 values were measured. Additional samples were taken and the ODs were measured at 4, 8, 12, 16, and 24 h. Corresponding dilutions of the lichen extract without the bacteria were used as blanks.
Time kill assay
The antimicrobial activity of L. vulpina extract against MRSA was evaluated by measuring the reduction in the numbers of CFU over 24 h. Cell suspensions of MRSA were prepared and treated with lichen extracts as described above. Bacterial viability was assessed at various time points (0, 8, 16, and 24 h) by spread-plating diluted bacterial suspensions onto Müller Hinton agar plates. After 24 h of incubation at 37 °C, the colonies were counted and data were presented as CFU (% of control) = (number of CFUs from lichen extract-treated sample/number of CFUs from control sample) × 100 (Andra et al., Citation2008).
Propidium iodide uptake assay
The effect of the L. vulpina extract on MRSA membrane permeability was studied by measuring propidium iodide (PI) uptake by bacterial cells. Cell suspensions of MRSA, prepared as described above, were treated separately for 24 h with various concentrations of the extract. The protocol of Nobles et al. (Citation2013) was followed with slight modifications. After the 24 h treatment, the bacterial cells were pelleted by centrifugation and washed once with 1 × phosphate buffer solution (PBS). Ten-fold dilutions of the cell suspensions were made in PBS, and PI (50 µg/ml) was added to the cells. A 200 µl aliquot of the cell suspension was transferred in triplicate to a 96-well plate, which was incubated at room temperature for 15 min in the dark. Fluorescence was measured at excitation and emission wavelengths of 535 and 625 nm, respectively, using a Synergy HT Multi-Mode Microplate reader (BioTek, Winooski, VT).
Transmission electron microscopy
In an effort to better understand the membrane damaging properties of this lichen extract, transmission electron microscopy (TEM) was performed. MRSA suspensions were treated with the extract of L. vulpina at 10 × MIC for 24 h at 37 °C. This concentration was selected in order to induce the maximum effect on the largest percentage of bacterial cells. After treatment, the cells were pelleted and embedded in 1% low melting point agarose. The cells were then fixed with 2.5% buffered glutaraldehyde for 1 h, washed with washing buffer, and then post-fixed in osmium tetroxide for 1 h before staining en bloc with 1% uranyl acetate overnight. The buffer used was 0.1 M sodium cacodylate, at pH 7.4. After staining overnight, the bacterial cells were dehydrated in a graded acetone series and then embedded in epoxy resin. Ultra-thin sections were prepared and stained with 1% uranyl acetate and lead citrate. Microscopy was performed using a Tecnai F30 transmission electron microscope (Tescan Inc., Warrendale, PA), under standard operating conditions.
Statistical analysis
All experiments were repeated three times with at least two replicates for each experiment. Results are reported as mean values ± SD of the three independent runs. One-way analysis of variance and Dunnett's multiple comparison were used to determine the significant differences between all measured parameters (p < 0.05). Statistical analyses were performed using GraphPad Prism 6 software (GraphPad, San Diego, CA).
Results
Extrolites of two lichens
To characterize the chemical compounds present in the extract of L. vulpina, we used thin-layer chromatography. Results showed that the L. vulpina extract contained exclusively vulpinic acid. The chemical structure of vulpinic acid is shown in .
Minimum inhibitory concentration
This study is a follow up to two previously published studies (Shrestha & St. Clair, Citation2013a; Shrestha et al., Citation2014), wherein acetone and methanol extracts of 36 different lichen species were screened for activity against four different pathogenic bacteria. In this study, we used acetone extracts of L. vulpina, to examine its mode of action against MRSA. The MIC value reported was 31.25 µg/ml; in this study, we used three concentrations: 31.25, 156.25, and 312.5 µg/ml which represents 1×, 5×, and 10 × the MIC value, respectively. The vehicle control (DMSO) did not have any effect on the viability of MRSA, even at the concentration equivalent to a 10 × MIC concentration of lichen extract.
Bacteriolysis assay
To assess whether the lichen extracts had a bacteriolytic effect, we measured the OD660 value of the treated bacterial cultures at various time points (). When the MRSA cultures were treated with 1 × MIC and 5 × MIC concentrations of the extract, there was an increase in the OD660 values. However, the increase in OD660 values in the treated cultures (1 × and 5 × MIC) was lower than the controls, and the OD660 value for the 10 × MIC-treated culture remained unchanged, even after 24 h ().
Figure 2. Bacteriolytic activity of lichen extract against MRSA. Cultures were incubated without extract (control) and with three different concentrations of the L. vulpina extract. The OD660 value was measured at various time points. Error bars indicate the standard deviation of the mean of three independent experiments with two replicates each (n = 6).
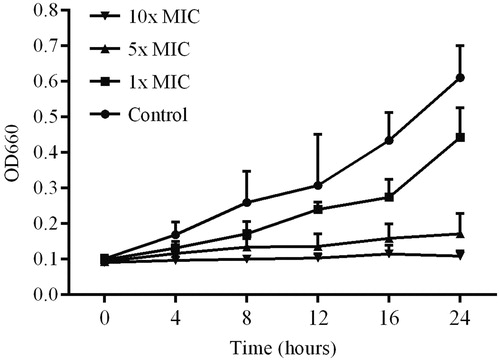
Time kill assay
Bacterial cell killing kinetics by the lichen extract was assessed by plating out treated bacterial suspensions and counting the number of viable cells at different time points. Treatment of MRSA cultures with the L. vulpina extract showed a reduction in CFU when compared with the control (). However, the bacterial cell killing kinetics were relatively slow. After 24 h, we observed a 34, 77, and 91% reduction in CFU when cultures were treated with 1×, 5×, and 10 × MIC of the L. vulpina extract, respectively.
Figure 3. Bacterial kill kinetics data for the lichen extract applied to MRSA cultures. Cultures incubated without the extract served as the control. Three different concentrations of the L. vulpina extract were tested. The viability was assessed by plating samples at various time periods and is presented as CFU (expressed as % of control).
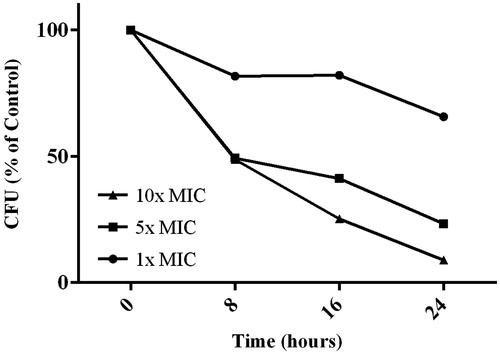
PI uptake assay
To test whether the lichen extract caused membrane instability, we added PI to the treated cultures after 24 h. PI, when associated with DNA, fluoresces intensely, and its use in this context indicates whether or not cell membranes are more permeable to PI after treatment (Arndt-Jovin & Jovin, Citation1989). Our data showed that there was a significant increase (p < 0.05) in the fluorescence values when MRSA cultures were treated with 1×, 5×, and 10 × MIC of the lichen extract (). We also observed a steady increase in fluorescence level with increasing extract concentrations.
Figure 4. Data showing propidium iodide uptake by MRSA cultures treated with increasing concentrations of the lichen extract. Cultures were incubated without extract (control) and with three different concentrations of the L. vulpina extract. Error bars indicate the standard deviation of the means of two independent experiments with three replicates each (n = 6). **p < 0.01 and ****p < 0.0001.
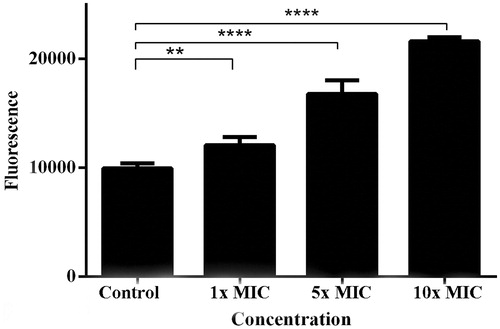
Transmission Electron Microscopy
To further document the suspected extract-related damage to bacterial cell membranes, and to explore possible additional mechanisms of action, we used TEM to examine thin sections of MRSA cells which had been treated with 10 × MIC concentrations of L. vulpina extract for 24 h. Untreated cells are shown in . The L. vulpina extract adversely affected MRSA cell membranes causing abnormal morphology (). In addition, we also found that treatment with the L. vulpina extract interrupted the normal cell division process ().
Discussion
MRSA isolates have evolved resistance to a range of β-lactam antibiotics, thus limiting effective treatment options. This condition has resulted in a concerted effort to identify and develop new potential therapies for treatment of these strains (Stapleton & Taylor, Citation2002). The antimicrobial activity of some lichen compounds indicates promise as a potential solution to this problem. Since the first report of lichen antimicrobial properties (Burkholder et al., Citation1944), several studies have documented the activities of various lichen extracts/chemicals against different pathogenic bacterial strains. Research (Boustie et al., Citation2011; Cocchietto et al., Citation2002; Francolini et al., Citation2004; Lauterwein et al., Citation1995; Molnar & Farkas, Citation2010; Pompilio et al., Citation2013; Ramos et al., Citation2014; Ranković et al., Citation2014) has shown that lichen-derived compounds show promise as potentially effective antibacterial agents. Most of these studies have focused on screening various crude extracts or isolated lichen compounds against different bacterial strains. However, our research provides some of the first data describing possible mechanisms of action for lichen extracts against pathogenic bacterial strains. Specifically, this study provides mechanism of action-related data for a crude extract from L. vulpina against a MRSA isolate.
Many antimicrobial agents containing polyphenolics cause gross membrane damage and whole-cell lysis (Sikkema et al., Citation1995). Our study showed that there was no significant reduction in the OD660 value, even at the highest concentration (10 × MIC) of the extract after 24 h. This suggests that the primary mode of action of the lichen-derived polyphenolic compound is not gross cell membrane damage. We also found steady increases in the absorbance at OD660 value with 1 × MIC and 5 × MIC, however, the absorbance was still lower than the control. Gupta et al. (Citation2012) also found increases in the absorbance after a 24 h treatment of MRSA cultures with various concentrations of usnic acid.
Sub-lethal injury of microbes may alter the permeability of cell membranes and affect the membrane's capacity to osmoregulate or to exclude toxic materials (Gilbert, Citation1984). It appears that treatment of MRSA cells with the L. vulpina extracts was fatal, but the killing kinetics was slow. The time kill assay showed that there was a significant reduction in the number of CFUs after 24 h of exposure. The PI uptake assay also revealed that there was a dose-dependent increase in the uptake of PI by treated cells. Many polyphenolic compounds, derived either from lichens or higher plants, have given similar results (Alwash et al., Citation2013; Carson et al., Citation2002; Gupta et al., Citation2012). We found viable bacterial cells after 24 h of treatment, even at the highest extract concentrations (10 × MIC), indicating a slow kill rate. Similar results were also reported by Gupta et al. (Citation2012).
Examination of lichen extract-treated MRSA cells using electron microscopy further documented the inability of this extract to lyse MRSA cells, in spite of clear evidence of damage to cell membranes. The membrane damaging activity of usnic acid has also been previously demonstrated (Gupta et al., Citation2012). Our data also provide preliminary documentation suggesting that the L. vulpina extract may also affect cell division processes. Many polyphenolic compounds have been shown to affect cell division processes in different strains of pathogenic bacteria. FtsZ, a tubulin-like GTPase, plays a central role in bacterial cell division. Inhibition of FtsZ polymerization prevents cells from dividing, leading ultimately to cell death (Wang et al., Citation2003). Crysophaentin A, a natural polyphenolic compound isolated from a marine alga, has been shown to inhibit FtsZ (Plaza et al., Citation2010). Similarly, another polyphenolic compound, viriditoxin, produced by a species of the fungus Aspergillus, also inhibits FtsZ. It is important to mention here that lichens are symbiotic associations between a fungus and an alga, and they produce an impressive variety of polyphenolic compounds. Therefore, the results of this study demonstrate the merits of further biochemical investigations of lichen extracts, as a possible source for new antibacterial compounds that specifically target cell division processes in MRSA.
Our thin layer chromatography results have shown that L. vulpina contain only vulpinic acid as its secondary compound. Other published reports also suggest that vulpinic acid is the major compound present in L. vulpina (Abo-Khatwa et al., Citation1996; Lawrey, Citation1983). So, it can be further inferred that the mode of action we observed in to the crude extract of L. vulpina was due to vulpinic acid.
Conclusion
A study by Gupta et al. (Citation2012) demonstrated that usnic acid, a commonly occurring polyphenolic compound in many species of lichens, can destabilize the membrane integrity of MRSA. Similar to the Gupta study, our results also show that L. vulpina extracts disrupt the integrity of MRSA membranes. In addition, our data document that L. vulpina extracts also impact MRSA cell division processes. However, we cannot eliminate the possibility of other modes of action in addition to destabilizing membranes and disrupting cell division processes, mediated by this L. vulpina extract. As indicated in the “Introduction” section, there are many studies showing promising results for the antimicrobial properties of lichen metabolites, but studies exploring modes of action are very limited. Our study opens the door for future mechanistic research, and L. vulpina merits further investigation.
Declaration of interest
The authors report that they have no conflicts of interest.
References
- Abo-Khatwa AN, Al-Robai AA, Al-Jawhari DA. (1996). Lichen acids as uncouplers of oxidative phosphorylation of mouse-liver mitochondria. Nat Toxins 4:96–102
- Alwash MS, Ibrahim N, Ahmad WY. (2013). Identification and mode of action of antibacterial components from Melastoma malabathricum Linn. leaves. Am J Infect Dis 9:46–58
- Andra J, Jakovkin I, Grotzinger J, et al. (2008). Structure and mode of action of the antimicrobial peptide arenicin. Biochem J 410:113–22
- Araújo AAS, de Melo MGD, Rabelo TK, et al. (2015). Review of the biological properties and toxicity of usnic acid. Nat Prod Res. [Epub head of print]. doi:10.1080/14786419.2015.1007455
- Arndt-Jovin DJ, Jovin TM. (1989). Fluorescence labeling and microscopy of DNA. Method Cell Biol 30:417–48
- Boustie J, Tomasi S, Grube M. (2011). Bioactive lichen metabolites: Alpine habitats as an untapped source. Phytochem Rev 10:287–307
- Burkholder PR, Evans AW, Mcveigh I, Thornton HK. (1944). Antibiotic activity of lichens. Proc Natl Acad Sci USA 30:250–5
- Carson CF, Mee BJ, Riley TV. (2002). Mechanism of action of Melaleuca alternifolia (tea tree) oil on Staphylococcus aureus determined by time-kill, lysis, leakage, and salt tolerance assays and electron microscopy. Antimicro Agents Ch 46:1914–20
- Cocchietto M, Skert N, Nimis P, Sava G. (2002). A review on usnic acid, an interesting natural compound. Naturwissenschaften 89:137–46
- Collignon PJ. (2002). Antibiotic resistance. Med J Aust 177:325–9
- Francolini I, Norris P, Piozzi A, et al. (2004). Usnic acid, a natural antimicrobial agent able to inhibit bacterial biofilm formation on polymer surfaces. Antimicro Agents Ch 48:4360–5
- Galun M. (1988). CRC Handbook of Lichenology (Vol II). Boca Raton (FL): CRC Press, Inc
- Gilbert P. (1984). The revival of micro-organisms sublethally injured by chemical inhibitors. Soc Appl Bacteriol Symp Ser 12:175–97
- Gupta V, Verma S, Gupta S, et al. (2012). Membrane-damaging potential of natural l-(−)-usnic acid in Staphylococcus aureus. Eur J Clin Microbiol 31:3375–83
- Hunn ES. (1990). Nch'i-Wana, “The Big River”: Mid-Columbia Indians and Their Land. Seattle: University of Washington Press
- Isnansetyo A, Kamei Y. (2003). MC21-A, a bactericidal antibiotic produced by a new marine bacterium, Pseudoalteromonas phenolica sp. nov. O-BC30T, against methicillin-resistant Staphylococcus aureus. Antimicro Agents Ch 47:480–8
- Kokubun T, Shiu WK, Gibbons S. (2007). Inhibitory activities of lichen-derived compounds against methicillin-and multidrug-resistant Staphylococcus aureus. Planta Med 73:176–9
- Lauterwein M, Oethinger M, Belsner K, et al. (1995). In vitro activities of the lichen secondary metabolites vulpinic acid,(+)-usnic acid, and (−)-usnic acid against aerobic and anaerobic microorganisms. Antimicro Agents Ch 39:2541–3
- Lawrey JD. (1983). Vulpinic and pinastric acids as lichen antiherbivore compounds: Contrary evidence. Bryologist 86:365–9
- Leeb M. (2004). Antibiotics: A shot in the arm. Nature 431:892–3
- Liapikou A, Torres A. (2013). Emerging drugs on methicillin-resistant Staphylococcus aureus. Expert Opin Emerg Dr 18:291–305
- Malhotra S, Subban R, Singh A. (2007). Lichens – Role in traditional medicine and drug discovery. Int J Alter Med 2:1–9
- Mobashery S, Azucena E. (2002). Bacterial antibiotic resistance. Encycl Life Sci 2:472–7
- Molnar K, Farkas E. (2010). Current results on biological activities of lichen secondary metabolites: A review. Z Naturforsch(C) 65:157–73
- Nobles CL, Green SI, Maresso AW. (2013). A product of heme catabolism modulates bacterial function and survival. PLoS Pathog 9:e1003507
- Odds FC, Rinaldi MG, Sheehan DJ, Warnock DW. (2001). Antifungal susceptibility testing: Practical aspects and current challenges. Clin Microbiol Rev 14:643–58
- Plaza A, Keffer JL, Bifulco G, et al. (2010). Chrysophaentins A-H, antibacterial bisdiarylbutene macrocycles that inhibit the bacterial cell division protein FtsZ. J Am Chem Soc 132:9069–77
- Pompilio A, Pomponio S, Di Vincenzo V, et al. (2013). Antimicrobial and antibiofilm activity of secondary metabolites of lichens against methicillin-resistant Staphylococcus aureus strains from cystic fibrosis patients. Future Microbiol 8:281–92
- Ramos DDBM, Gomes FS, Napoleão TH, et al. (2014). Antimicrobial activity of Cladonia verticillaris lichen preparations on bacteria and fungi of medical importance. Chin J Biol. [Epub ahead of print]. https://doi.org/http://dx.doi.org/10.1155/2014/219392
- Ranković B, Kosanić M, Manojlović N, et al. (2014). Chemical composition of Hypogymnia physodes lichen and biological activities of some its major metabolites. Med Chem Res 23:408–16
- Saleem M, Nazir M, Ali MS, et al. (2010). Antimicrobial natural products: An update on future antibiotic drug candidates. Nat Prod Rep 27:238–54
- Shrestha G, Raphael J, Leavitt SD, St. Clair LL. (2014). In vitro evaluation of the antibacterial activity of extracts from thirty-four species of North American lichens. Pharm Biol 52:1262–6
- Shrestha G, St. Clair LL. (2013a). Antimicrobial activity of extracts from two lichens Ramalina menziesii and Usnea lapponica. Bull California Lichen Soc 20:5–10
- Shrestha G, St. Clair LL. (2013b). Lichens: A promising source of antibiotic and anticancer drugs. Phytochem Rev 12:229–44
- Sikkema J, De Bont J, Poolman B. (1995). Mechanisms of membrane toxicity of hydrocarbons. Microbiol Rev 59:201–22
- Stapleton PD, Taylor PW. (2002). Methicillin resistance in Staphylococcus aureus: Mechanisms and modulation. Sci Prog 85:57–72
- Stojanovic G, Stojanovic I, Smelcerovic A. (2012). Lichen depsidones as potential novel pharmacologically active compounds. Mini-Rev Org Chem 9:178–84
- Taylor AR. (2013). Methicillin-resistant Staphylococcus aureus infections. Primary Care 40:637–54
- Wang J, Galgoci A, Kodali S, et al. (2003). Discovery of a small molecule that inhibits cell division by blocking FtsZ, a novel therapeutic target of antibiotics. J Biol Chem 278:44424–8
- Wilson BA, Salyers AA, Whitt DD, Winkler ME. (2011). Bacterial Pathogenesis: A Molecular Approach. Washington (DC): ASM Press