Abstract
Context: Despite phytochemical studies of Agrimonia pilosa Ledeb. (Rosaceae), the antidiabetic effects of this plant are unknown.
Objective: This study characterizes the isolated compounds from the aerial parts of A. pilosa and evaluates their PTP1B and α-glucosidase inhibitory properties.
Materials and methods: Ethanol extract of A. pilosa was found to inhibit 64% PTP1B activity at 30 μg/mL. The ethanol extract was partitioned with methylene chloride, ethyl acetate, n-butanol, and water fractions. Among these, the ethyl acetate fraction displayed the most potent PTP1B activity. The ethyl acetate extract was separated by chromatographic methods to obtain flavonoids and triterpenoids (1–11); which were evaluated for their inhibitory effects on PTP1B activity with p-nitrophenyl phosphate (p-NPP) as a substrate, and also α-glucosidase enzyme.
Results: Compounds 1–11 were identified as apigenin-7-O-β-d-glucuronide-6″-methyl ester, triliroside, quercetin-7-O-β-d-glycoside, quercetin-3-O-β-d-glycoside, kaempferol, kaempferol-3-O-α-l-rhamnoside, β-sitosterol, ursolic acid, tormentic acid, methyl 2-hydroxyl tricosanoate, and palmitic acid. Compounds 8, 9, and 11 displayed inhibitory effects on PTP1B activity with IC50 values of 3.47 ± 0.02, 0.50 ± 0.06, and 0.10 ± 0.03 μM, respectively. Compounds 3, 4, 6, and 9 exhibited inhibition of the α-glucosidase activity with IC50 values of 11.2 ± 0.2, 29.6 ± 0.9, 28.5 ± 0.1, and 23.8 ± 0.4 μM, respectively.
Discussion and conclusion: As major ingredients of A. pilosa, compounds 1, 6, 8, and 9 showed the greatest inhibitory potency on PTP1B activity. Compounds 3, 6, 8, and 9 also showed potent inhibitory effects on α-glucosidase enzyme. This result suggested the potential of these compounds for developing antidiabetic agents.
Introduction
Diabetes mellitus is now a major and growing public health problem throughout the world. According to the World Health Organization (WHO), 346 million people globally suffer from diabetes since 2011 and its prevalence is projected to double by 2030 (Danaei et al., Citation2011). Among the diseases, type 2 diabetes is characterized by chronic hyperglycemia associated with abnormalities in carbohydrate, fat, and protein metabolism. The American Diabetes Association reported that about 22.3 million people in the United States have diabetes, accounting for 7% of the population (Erratum, Citation2013). Thus, great efforts are being made in the search for new therapeutic agents to stem its progress (Kumar et al., Citation2011).
Protein tyrosine phosphatases (PTPs) are involved in the down-regulation of cellular signal transduction mediated by receptor tyrosine kinases such as insulin receptor and epidermal growth factor receptor (Burke & Zhang, Citation1998). Protein tyrosine phosphatases 1B (PTP1B), a member of the PTP family, is thought to function as a negative regulator of insulin signal transduction. PTP1B directly interacts with activated insulin receptor or insulin receptor substrate-1 (IRS-1) to dephosphorylate phosphotyrosine residues, resulting in down-regulation of insulin action (Goldstein et al., Citation2000). PTP1B knock-down mice show enhanced insulin sensitivity in glucose and insulin tolerance tests, indicating that PTP1B is a major player in the modulation of insulin sensitivity (Elchebly et al., Citation1999; Klaman et al., Citation2000). Increased expression of PTP1B in adipose tissue and muscle of obese humans and rodents is thought to be related to insulin resistance (Wu et al., Citation2001), whereas the increased insulin sensitivity from weight loss is accompanied by reduced PTP1B activity (Ahmad et al., Citation1997a,Citationb). PTP1B overexpression in rat primary adipose tissues and 3T3/L1 adipocytes has been shown to decrease insulin-sensitive Glut4 translocation (Chen et al., Citation1999) and insulin receptor and IRS-1 phosphorylation (Venable et al., Citation2000), respectively. As with the insulin signaling pathway, the leptin signaling pathway can be attenuated by PTPs and there is evidence that PTP1B is also involved in this process. Therefore, it has been suggested that compounds that reduce PTP1B activity or expression levels can not only be used for treating type 2 diabetes but also obesity (Moller, Citation2001; Zhang & Lee, Citation2003). Although there have been a number of reports on the design and development of these PTP1B inhibitors, including difluoromethylene phosphonates, 2-carbomethoxybenzoic acids, 2-oxalylaminobenzoic acids, and several lipophilic compounds (Andersen et al., Citation2000; Bialy & Waldmann, Citation2003; Burke et al., Citation1994; Liljebris et al., Citation2002; Zhang & Zhang, Citation2007). However, new types of PTP1B inhibitors with suitable pharmacological properties remain to be discovered.
Agrimonia pilosa Ledeb. (Rosaceae) is a perennial herb which grown throughout Korea, China, and Japan. Its erect stem grows about 30–100 cm in height. The leaves are green, oval, and hairy: they are 1–4 cm long and 0.5–2.5 cm wide. Biological studies have revealed that this plant possessed numerous effects. Ethyl acetate extract showed anti-acetylcholinesterase activity (Jung & Park, Citation2007), methanol extract acted as antitumor activity (Miyamoto et al., Citation1987), ethanol extract showed anti-inflammatory (Jung, Citation2010) and anti-viral activities (Shin et al., Citation2010), and water extract showed analgesic (Gong et al., Citation2006) and hemostasis activities (Hong et al., Citation2008). As part of an ongoing investigation for discovering new antidiabetic agents from medicinal plants, the ethanol extract of the aerial parts of A. pilosa showed an inhibitory effect of 64% on PTP1B activity at a concentration of 30 µg/mL. Thus, assay-guided separation of this extract has led to the isolation of six flavonoid glycosides (1–6), two triterpenoids (7–9), and two long chain fatty acids (10 and 11). Furthermore, the inhibitory effects of these isolates on the PTP1B and α-glucosidase enzyme activities were evaluated in vitro and a kinetic study was performed to investigate their inhibition modes. In this report, the purification, structural determination, and antidiabetic properties of these isolates are discussed.
Materials and methods
General experimental procedures
The specific rotations were determined on a JASCO DIP-1000 digital polarimeter (JASCO Corp., Tokyo, Japan) using a 100-mm glass microcell. The UV spectra were recorded in MeOH using a Shimadzu UV spectrometer (Shimadzu Corp., Kyoto, Japan). The IR spectra (KBr) were recorded on a Nicolet 6700 FT-IR spectrometer (Thermo Electron Corp., Tokyo, Japan). The NMR spectra were recorded in methanol-d4 (CD3OD) on Varian Oxford-AS 400 MHz instrument (Varian, Palo Alto, CA), with TMS as the internal standard, at the Department of Pharmacy, Catholic University of Daegu, Korea. All mass spectrometric experiments were performed on a Quattro II mass spectrometer at Korea Basic Science Institute Daegu Center (KBSI, Daegu, Korea). Silica gel (63–200 µm particle size) and RP-C18 (40–63 µm particle size) for column chromatography were from Merck (Darmstadt, Germany). Thin-layer chromatography (TLC) was carried out on precoated Merck silica gel 60 F254 and RP-C18 F254 plates from Merck (Darmstadt, Germany). HPLC was carried out using a Gilson System (Middleton, WI) with a UV detector and an Optima Pak C18 column (10 × 250 mm, 10 µm particle size, RS Tech Corporation, Seoul, Korea). HPLC solvents were obtained from Fisher Scientific Korea Ltd. (Seoul, Korea). All other reagents were of the highest analytical grade.
Plant material
The aerial parts of A. pilosa were collected from the herbal garden of the Catholic University of Daegu, Korea, in September 2011. The plant material was authenticated by one of the author (B. S. M.). A voucher specimen (20100923) was deposited in the herbarium of College of Pharmacy, Catholic University of Daegu, Korea.
Extraction and isolation
The aerial parts of A. pilosa (10.8 kg) were dried and then extracted with 95% ethanol using sonication at 50 °C for 10 h. The ethanol extract was evaporated under reduced pressure to give a crude extract (2.52 kg). A 2.5 kg aliquot was then suspended in H2O (2.0 L) and partitioned successively with CH2Cl2 (2 L × 4, 1130 g), EtOAc (2 L × 4, 165 g), n-BuOH (2 L × 4, 500 g), and H2O (700 g). The CH2Cl2, EtOAc, n-BuOH, and H2O-soluble layers were investigated for their PTP1B inhibitory activities. Among these, the EtOAc fraction demonstrated the most potent activity. Thus, this fraction was subjected to several column chromatographies to yield compounds 1–11 (). The chemical structures of the isolated compounds were determined by extensive analysis of the spectroscopic and physicochemical data, as well as the comparison with published literature ().
PTP1B inhibitory assay
Protein tyrosine phosphatase 1B (human recombinant) was purchased from Biomol International LP, Plymouth Meeting, PA, and the inhibitory activities of the tested samples were evaluated using p-nitrophenyl phosphate (p-NPP) as a substrate (Nguyen et al., Citation2011). Briefly, to each well (final volume 110 μL) were added 2 mM p-NPP and PTP1B in a buffer containing 50 mM citrate (pH 6.0), 0.1 M NaCl, 1 mM EDTA, and 1 mM dithiotheritol (DTT), with or without sample. The plate was preincubated at 37 °C for 10 min, and then 50 μL of p-NPP in buffer was added. Following incubation at 20 °C for 20 min, the reaction was terminated with the addition of 10 M NaOH. The amount of p-nitrophenyl produced after enzymatic dephosphorylation was estimated by measuring the absorbance at 405 nm using a VERSA Max microplate reader (Molecular Devices, Sunnyvale, CA). The non-enzymatic hydrolysis of 2 mM p-NPP was corrected by measuring the increase in absorbance at 405 nm obtained in the absence of PTP1B enzyme. The inhibition (%) was calculated as (Ac − As)/Ac × 100%, where Ac is the absorbance of the control and As is the absorbance of the sample. RK-682 was used as positive control.
Determination of the inhibition mode of active compounds
The Lineweaver–Burk plot and Dixon plot experiments were carried out in the presence and absence of the inhibitors with various concentrations of p-NPP as the substrate. The inhibition modes of the tested compounds were assessed on the basis of their inhibitory effects on the Km (dissociation constant) and Vmax (maximum reaction velocity) of the enzyme, which were determined by Lineweaver–Burk plot experiment. The Lineweaver–Burk plot is the double reciprocal plot of the enzyme reaction velocity (V) versus the substrate (p-NPP) concentration (1/V versus 1/[p-NPP]) (Nguyen et al., Citation2010).
α-Glucosidase inhibitory assay
The α-glucosidase enzyme assay was carried out using the reported procedure (Li et al., Citation2005) with some modifications. Briefly, to each well (final volume 80 µL) were added 20 µL 100 mM phosphate buffer (pH 6.8), 20 µL 2.5 mM p-NPG, 20 µL tested sample (dissolved in DMSO), and 20 µL α-glucosidase enzyme [0.2 U/mL in 10 mM phosphate buffer (pH 6.8)]. The plate was incubated at 37 °C for 15 min. The reaction was then terminated with the addition of 80 µL 0.2 M Na2CO3. The amount of p-nitrophenyl produced after enzymatic dephosphorylation was estimated by measuring the absorbance at 405 nm using a VERSA Max microplate reader (Molecular Devices, Sunnyvale, CA). The inhibition (%) was calculated as (Ac − As)/Ac × 100%, where Ac is the absorbance of the control and As is the absorbance of the sample. Acarbose was used as a positive control.
Statistical analysis
Data are represented as means ± SD of at least three independent experiments performed in triplicated assays and determined by regression analysis. For statistical analysis of the data for single comparison, the significance between means was determined by the Student t test. Sigma Plot program version 11.0 (SPSS Inc., Chicago, IL) was used for analysis of the kinetic data.
Results and discussion
In the continuing program to search for new PTP1B inhibitors from medicinal plants, the ethanol extract of the aerial parts of A. pilosa was found to inhibit PTP1B activity in vitro (>60% inhibition at 30 µg/mL). Thus, bioassay-guided isolation of this extract using chromatographic methods led to the isolation of six flavonoid glycosides (1–6), three triterpenes (7–9), and two long-chain fatty acid derivatives (10 and 11) (). Their chemical structures were determined by extensive analysis of the physicochemical and spectroscopic data, as well as by comparing with reported literatures. Apigenin-7-O-β-d-glucuronide-6″-methyl ester (1) was isolated as a yellow amorphous powder from A. pilosa for the first time. It was first identified in 1985 by Lin et al. (Citation2003) before isolated from the aqueous ethanolic extract of the aerial parts of Origanum vulgare L. (Lamiaceae). Triliroside (2) was first isolated from the seeds of Eremocarpus setigerus (Bajaj et al., Citation1986). It was reported to possess antidiabetic and anti-obese properties by enhancing adiponectin secretion and GLUT4 translocation in 3T3-L1 cells (Song et al., Citation2013). Quercetin-7-O-β-d-glycoside (3) was also isolated from A. pilosa for the first time (Mohamed et al., Citation1997). Quercetin-3-O-β-d-glycoside (4), kaempferol (5), and kaempferol-3-O-α-l-rhamnoside (6) were isolated previously from the aerial parts of A. pilosa by Liu et al. (Citation2008) and from the Agrimoniae Herba by Fang et al. (Citation2012). In addition, compounds 7–9 were isolated previously from A. pilosa by An et al. (Citation2005); their chemical structures were identified as β-sitosterol (7), ursolic acid (8), and tormentic acid (9). Compound 10 was isolated, as white powder, and established as methyl 2-hydroxyl tricosanoate (10) (Yamada et al., Citation2001). This is the first time that methyl 2-hydroxyl tricosanoate has been isolated from A. pilosa. The long-chain fatty acid, compound 11, was identified as palmitic acid (Liu et al., Citation2008). It has been isolated previously from A. pilosa by Fang et al. (Citation2012).
The PTP1B inhibitory effects of the isolated compounds (1–11) were evaluated using an in vitro assay as described previously (Nguyen et al., Citation2011). Except for compounds 2 and 3, all isolates exhibited potent inhibitory effects against PTP1B enzyme with IC50 values ranging from 0.10 ± 0.03 to 49.78 ± 1.42 μM. The positive control, RK-682 (Cui et al., Citation2010; Hamaguchi et al., Citation1995), displayed an IC50 value of 4.47 ± 0.03 μM. Of the isolates, compounds 9 and 11 exhibited the most potency with IC50 values of 0.50 ± 0.06 and 0.10 ± 0.03 μM, respectively. The ursane-type triterpene 9 and long-chain fatty acid (11) displayed the IC50 values smaller than RK-682 about 10 and 30 times, respectively. As a triterpene, ursolic acid (8) exhibited equivalent activity (an IC50 value of 3.47 ± 0.02 μM) to the positive control, RK-682 (IC50 value = 4.47 ± 0.03 μM). Furthermore, compounds 4 (IC50 value = 27.73 ± 1.54 μM) and 6 (IC50 value = 12.16 ± 0.02 μM), with an O-β-d-glucoside attached at C-3 position, displayed stronger activity than simple flavonol 5 (IC50 value = 42.93 ± 2.36 μM). The attachment of a complex molecule to C-3 and/or the substitution of a glucose unit at C-7 may be responsible for the suppression of the activity of flavonols 2 and 3 (an IC50 value of >50 μM). Compound 1, as a flavone with an O-β-d-glucuronide-6″-methyl ester attached at C-7, displayed strong inhibitory effect with an IC50 value of 14.35 ± 0.76 μM. The above-obtained data reveal that the hydrophobic compounds (8–11), especially the ursane-type triterpenoids 8 and 9, have stronger inhibitory effects than the hydrophilic compounds (1–6) against PTP1B enzyme activity.
To determine the inhibition modes of the active compounds, the Lineweaver–Burk plot and Dixon plot experiments were carried out in the presence and absence of the inhibitors with various concentrations of p-NPP as the substrate (Nguyen et al., Citation2010). The Sigma Plot program (SPCC Inc., Chicago, IL) was used to analyze both the double reciprocal Lineweaver–Burk plot () and the Dixon plot (). lists the Ki values for the active compounds 1, 4, 6, 8, 9, and 11, which were determined from the x-axis values where the lines intersected. A kinetic study showed that the inhibition modes of compounds 1, 8, and 9, as determined using the Lineweaver–Burk () and Dixon plots () were mixtures of inhibition types. However, both experiments showed that compound 6 exhibited non-competitive inhibition because the Vmax values decreased with concentration without changing the Km for the substrate, and the lines intersected at a value of 1/[S] under zero on the x-axis (at 1/(intensity/min) = 0) (). In contrast, increasing the substrate concentrations resulted in a series of lines that did not intersect on the y-axis in the Lineweaver–Burk plot () and the x-axis in Dixon plots (), but paralleled with each other, confirming that compound 4 shows uncompetitive inhibition. In addition, the pattern of straight lines with intersecting y intercepts () is characteristic of competitive inhibitor, indicating that compound 11 shows competitive inhibition.
Figure 3. Graphical determination of inhibition type for the isolated compounds 1, 4, 6, 8, 9, and 11. Lineweaver–Burk plots for the inhibition of compounds 1, 4, 6, 8, 9, and 11 on the PTP1B-catalyzed hydrolysis of p-NPP. Data are expressed as the mean reciprocal of initial velocity for n = 3 replicates at each substrate concentration.
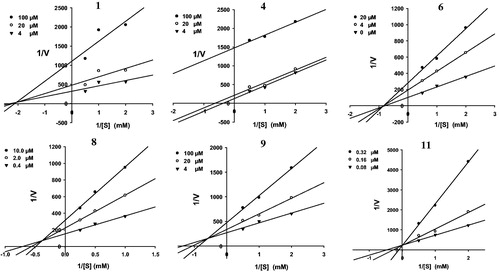
Figure 4. Graphical determination of inhibition type for the isolated compounds 1, 4, 6, 8, 9, and 11. Dixon plots for compounds 1, 4, 6, 8, 9, and 11 used for determining the inhibition constant Ki. Ki values were determined from the negative x-axis value at the point of the intersection of the three lines.
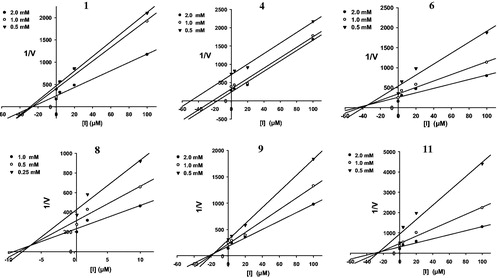
Table 1. Inhibitory effects of isolated compounds (1–11) on the PTP1B and α-glucosidase enzyme activities.
Postprandial hyperglycemia plays an important role in the development of type 2 diabetes and its complications. One therapeutic approach to treat postprandial hyperglycemia is to retard the cleavage of glucose from disaccharide via inhibition of α-glucosidase in the digestive organs (Tewari et al., Citation2003). In this regard, we measured the inhibitory effects of compounds 1–11 on the α-glucosidase enzyme. Acarbose was used as a positive control in this assay and showed significant activity with an IC50 value of 124.2 ± 0.4 μM. Of these isolates, compounds 3, 4, 6, and 9 showed the greatest potencies with IC50 values of 11.2 ± 0.2, 29.6 ± 0.9, 28.5 ± 0.1, and 23.8 ± 0.4 μM, respectively, While compounds 2, 8, and 11 exhibited potent inhibitory effects with IC50 values of 44.8 ± 0.9, 42.4 ± 0.7, and 45.5 ± 1.5 μM, respectively. Compounds 1 and 10 exhibited equivalent activity to the positive control with IC50 values of 103.3 ± 1.1 and 112.8 ± 1.7 μM, respectively.
Conclusions
Agrimonia pilosa is a perennial herbaceous flowering plant, which is distributed primarily in the temperate regions of the Northern Hemisphere (Yamada et al., Citation2001). The aerial parts of A. pilosa have been used as traditional medicine for the treatment of abdominal pain, sore throat, headaches, and heat stroke (Kato et al., Citation2010). Several triterpenoids and phenolics have been isolated from this plant, showing anticancer, antioxidant, anti-inflammatory, and hepatoprotective activities (Miyamoto et al., Citation1985; Park et al., Citation2004; Zhu et al., Citation2009). However, chemical components which are thought to be responsible for antidiabetic effect of the A. pilosa extract have not been investigated. In this study, compounds 8, 9, and 11, as major triterpenes, showed the strongest inhibitory potencies on the PTP1B activity with IC50 values of 3.47 ± 0.02, 0.50 ± 0.06, and 0.10 ± 0.03 μM, respectively. As major flavonoids, compounds 1 and 6 exhibited significant effects. Furthermore, compound 3 possessed the most inhibitory potency against α-glucosidase activity with an IC50 value of 11.2 ± 0.2 μM. In addition, compounds 4, 6, 8, and 9 showed potent effects IC50 values ranging from 23.8 ± 0.4 to 29.6 ± 0.9 μM, while acarbose, a positive control used in this assay, showed an IC50 value of 124.2 ± 0.4 μM. These results suggested the potential of compounds 1, 4, 6, 8, 9, and 11 as lead compounds for the developing antidiabetic agents; their antidiabetic properties may be related to their inhibitory effects on the PTP1B and α-glucosidase activities. Furthermore, the result shows that triterpenes and flavonoids may be the compounds responsible for the antidiabetic properties of A. pilosa.
Acknowledgements
The authors are grateful to the Korea Basic Science Institute (KBSI) for mass spectrometric measurements.
Declaration of interest
The authors declare no competing financial interest. This research was supported by the research grants, funded by the Catholic University of Daegu, in 2013.
References
- Ahmad F, Azevedo JJ, Cortright R, et al. (1997a). Alterations in skeleton muscle protein tyrosine phosphatase activity and expression in insulin-resistant human obesity and diabetes. J Clin Invest 100:449–58
- Ahmad F, Considine RV, Bauer TL, et al. (1997b). Improved sensitivity to insulin in obese subjects following weight loss is accompanied by reduced protein tyrosine phosphatase in adipose tissue. Metabolism 46:1140–5
- An RB, Kim HC, Jeong GS, et al. (2005). Constituents of the aerial parts of Agrimonia pilosa. Nat Prod Sci 11:196–8
- Andersen HS, Iversen LF, Jeppensen CB, et al. (2000). 2-(Oxalylamino) benzoic acid is a general competitive inhibitor of protein tyrosine phosphatases. J Biol Chem 275:7101–8
- Bajaj R, Chang CJ, McLaughlin JL, et al. (1986). Tiliroside from the seeds of Eremocarpus setigerus. J Nat Prod 49:1174–5
- Bialy L, Waldmann H. (2003). Inhibitors of protein PTP1B inhibitors as potential therapeutics in the treatment of type 2 diabetes and obesity. Expert Opin Investig Drugs 12:223–33
- Burke TR, Kole HK, Roller PP. (1994). Potent inhibition of insulin receptor dephosphorylation by a hexamer peptide containing the phosphotyrosyl mimetic F2Pmp. Biochem Biophys Res Commun 204:129–34
- Burke TR, Zhang ZY. (1998). Protein–tyrosine phosphatases: Structure, mechanism, and inhibitor discovery. Biopolymers 47:225–41
- Chen H, Cong LN, Li Y, et al. (1999). A phosphotyrosyl mimetic peptide reverses impairment of insulin-stimulated translocation of GLUT4 caused by overexpression of PTP-1B in rat adipose cells. Biochemistry 38:384–9
- Cui L, Lee HS, Ndinteh DT, et al. (2010). New prenylated flavanones from stem bark of Erythrina abyssinica with PTP1B inhibitory activity. Planta Med 76:713–18
- Danaei G, Finucane MM, Lu Y, et al. (2011). National, regional, and global trends in fasting plasma glucose and diabetes prevalence since 1980: Systematic analysis of health examination surveys and epidemiological studies with 370 country-years and 2.7 million participants. Lancet 378:31–40
- Elchebly M, Payette P, Michaliszyn E, et al. (1999). Increased insulin sensitivity and obesity resistance in mice lacking the protein tyrosine phophatase-1B gene. Science 283:1544–8
- Erratum. (2013). Economic costs of diabetes in the U.S. in 2012. Diabetes Care 36:1033–46
- Fang L, Xiaoyu B, Yongzhi H. (2012). Chemical constituents of Herba Agrimoniae. Zhongcaoyao 43:851–5
- Goldstein BJ, Bitter-Kowalczyk A, White MF, Harbeck M. (2000). Tyrosine dephosphorylation and deactivation of insulin receptor substrate-1 by protein tyrosine phosphatase 1B. Possible facilitation by the formation of a ternary complex with the GRB2 adaptor protein. J Biol Chem 275:4283–9
- Gong CG, Zhang GG, Wang XI, Lei YH. (2006). Experimental studies of anti-inflammatory and analgesic effects of extract from Herba Agrimoniae. J Pharm Pract 6:339–41
- Hamaguchi T, Sudo T, Osada H. (1995). RK-682, a potent inhibitor of tyrosine phosphatase, arrested the mammalian cell cycle progression at G1phase. FEBS Lett 372:54–8
- Hong G, Dai YH, Liu PX, et al. (2008). Advances in research on chemical constituents and pharmacological activities of Agrimonia pilosa. Pharm Care Res 8:362–6
- Jung CH. (2010). Inhibitory effect of Agrimonia pilosa Ledeb. on inflammation by suppression of iNOS and ROS production. Immunol Invest 39:159–70
- Jung M, Park M. (2007). Acetylcholinesterase inhibition by flavonoids from Agrimonia pilosa. Molecules 12:2130–9
- Kato H, Li W, Koike M, et al. (2010). Phenolic glycosides from Agrimonia pilosa. Phytochemistry 71:1925–9
- Klaman LD, Boss O, Peroni OD, et al. (2000). Increased energy expenditure, decreased adiposity, and tissue-specific insulin sensitivity in protein tyrosine phosphatase 1B-deficient mice. Mol Cell Biol 20:5479–89
- Kumar S, Narwal S, Kumar V, Prakash O. (2011). α-Glucosidase inhibitors from plants: A natural approach to treat diabetes. Pharmacogn Rev 5:19–29
- Li T, Zhang XD, Song YW, Liu JW. (2005). A microplate-based screening method for alpha-glucosidase inhibitors. Chin J Clin Pharmacol Ther 10:1128–34
- Liljebris C, Larsen SD, Ogg D, et al. (2002). Investigation of potential bioisosteric replacements for the carboxyl groups of peptidomimetic inhibitors of protein tyrosine phosphatase 1B: Identification of a tetrazole-containing inhibitor with cellular activity. J Med Chem 45:1785–98
- Lin YL, Wang CN, Shiao YJ, et al. (2003). Benzolignanoid and polyphenols from Origanum vulgare. J Chin Chem Soc 50:1079–83
- Liu PY, Yulei Z, Liqin D, et al. (2008). Studies on isolation and identification of flavonoids in herbs of Agrimonia pilosa. Zhongguo Zhongyao Zazhi 33:2925–8
- Miyamoto K, Koshiura R, Ikeya Y, Taguchi H. (1985). Isolation of agrimoniin, an anti-tumour constituent, from the roots of Agrimonia pilosa LEDEB. Chem Pharm Bull 33:3977–81
- Miyamoto K, Kishi N, Koshiura R. (1987). Antitumor effect of agrimoniin, a tannin of Agrimonia pilosa Ledeb. on transplantable rodent tumors. Jpn J Pharmacol 43:187–95
- Mohamed S, Mohamed AEA, Nabiel AMS. (1997). Flavonoids of four Cleome and three Capparis species. Biochem Syst Ecol 25:161–6
- Moller DE. (2001). New drug targets for type 2 diabetes and the metabolic syndrome. Nature 414:821–7
- Nguyen PH, Dao TT, Kim JY, et al. (2011). New 5-deoxyflavonoids and their inhibitory effects on protein tyrosine phosphatase 1B (PTP1B) activity. Bioorg Med Chem 19:3378–83
- Nguyen PH, Nguyen TNA, Kang KW, et al. (2010). Prenylated pterocarpans as bacterial neuraminidase inhibitors. Bioorg Med Chem 18:3335–44
- Park EJ, Oh H, Kang TH, et al. (2004). An isocoumarin with hepatoprotective activity in HepG2 and primary hepatocytes from Agrimonia pilosa. Arch Pharm Res 27:944–6
- Shin W, Lee K, Park M, Seong B. (2010). Broad-spectrum antiviral effect of Agrimonia pilosa extract on influenza viruses. Microbiol Immunol 54:11–19
- Song LL, Li D, Zhang MX, et al. (2013). Triliroside isolated from Agrimonia pilosa Ledeb. enhanced adiponectin secretion and GLUT4 translocation in 3T3-L1 cells. Lat Am J Pharm 32:524–30
- Tewari N, Tiwari VK, Mishra RC, et al. (2003). Synthesis and bioevaluation of glycosyl ureas as alpha-glucosidase inhibitors and their effect on mycobacterium. Bioorg Med Chem 11:2911–22
- Venable CL, Frevert EU, Kim YB, et al. (2000). Overexpression of protein tyrosine phosphatase 1B in adipocytes inhibits insulin-stimulated phosphoinositide 3-kinase activity without altering glucose transport or Akt/protein kinase B activation. J Biol Chem 275:18318–26
- Wu X, Hoffstedt J, Deeb W, et al. (2001). Depot-specific variation in protein tyrosine phosphatases activities in human omental and subcutaneous adipose tissue: A potential contribution to differential insulin sensitivity. J Clin Endocrinol Metab 86:5973–80
- Yamada K, Matsubara R, Kaneko M, et al. (2001). Constituents of Holothuroidea. 10. Isolation and structure of a biologically active ganglioside molecular species from the sea cucumber Holothuria leucospilota. Chem Pharm Bull 49:447–52
- Zhang S, Zhang ZY. (2007). PTP1B as a drug target: Recent developments in PTP1B inhibitor discovery. Drug Discov Today 12:373–81
- Zhang ZY, Lee SY. (2003). PTP1B inhibitors as potential therapeutics in the treatment of type 2 diabetes and obesity. Expert Opin Investig Drugs 12:223–33
- Zhu L, Tan J, Wang B, et al. (2009). Antioxidant activities of aqueous extract from Agrimonia pilosa LEDEB. and its fractions. Chem Biodivers 6:1716–26