Abstract
Context: Viola tianshanica Maxim. (Violaceae) is a perennial herb distributed in Central Asia, especially in the Xinjiang Uygur Autonomous Region (XUAR) of China. Preliminary study showed that the ethanol extract of the herb exhibited the anti-complement activity against the classical pathway, but the active components responsible for this capacity remain unknown and are yet to be studied.
Objective: The objective of this study was the isolation and identification of the anti-complement constituents of V. tianshanica.
Materials and methods: The ethyl acetate and n-butanol fractions from the ethanol extract of V. tianshanica were purified. The structures of the isolates were identified by spectroscopic methods, and comparing their spectral data with those reported in the literature. All the isolates (0.02–2.50 mg/mL) were evaluated for their anti-complement activity against the classical and alternative pathways.
Results: Twenty-one phenolic compounds including 15 flavonol O-glycosides (1–15), one flavone 6,8-di-C-glycoside (16), one flavone aglycone (17), and four phenolic acid derivatives (18–21) were isolated and identified. Bioassay showed that 11 compounds inhibited the classical pathway and the alternative pathway with CH50 and AP50 values of 0.113–1.210 mM and 0.120–1.579 mM, respectively. Preliminary mechanistic study using complement-depleted sera demonstrated that 1 acted on C1q, C2, C4, and C9 components, 16 on C1q, C4, and C5, and 21 on C1q, C3, C4, and C9.
Conclusion: All isolated compounds except 1 and 10 were reported for the first time from V. tianshanica. Compound 16 is the first flavone C-glycoside isolated from the herb. Flavonol O-glycosides and phenolic acids contributed the anti-complement activity of the herb.
Introduction
The human complement system is an essential component of innate immunity and plays an important role in modulating adaptive immunity as well. It comprises over 30 plasma and membrane-bound proteins and can be activated through three different pathways: the classical pathway (CP), the alternative pathway (AP), and the mannan-binding lectin pathway (MBL-P) (Kirschfink, Citation1997). Once activated inappropriately, the complement system is involved in the pathogenesis of several severe diseases such as systemic lupus erythematosus (SLE), rheumatoid arthritis (RA), and acute respiratory distress syndrome (ARDS) (Lustep & Clark, Citation2001; Makridea, Citation1998; Peiris et al., Citation2003). Therefore, inhibition of excessive complement activation might be a therapeutic strategy for the treatment of these diseases.
Viola tianshanica Maxim. (Violaceae) is a perennial herb distributed in Central Asia, especially in the Xinjiang Uygur Autonomous Region (XUAR) of China (ECCMM, Citation2005). The whole plants including roots have been used in traditional Uygur medicines as a heat-clearing and detoxicating drug for the treatment of fever, headache, pharyngalgia, and acute pyogenic infections such as boils, furuncles, and carbuncles (Shen et al., Citation2009a). Previous pharmacological studies have shown that the extracts of V. tianshanica have anti-inflammatory (Yang et al., Citation2011), antibacterial (Ma et al., Citation2004; Shen et al., Citation2009b), and antioxidative activities (Shen & Xie, Citation2009). It is used in XUAR as a substitute for Viola yedoensis Makino (Violaceae). However, very little is known about the phytochemical composition of V. tianshanica, with the exception of several flavonol O-glycosides (Yu et al., Citation2009), cyclotides (Xiang et al., Citation2010), and lignans (Qin et al., Citation2013). Our preliminary investigation showed that the ethanol extract of V. tianshanica had reproducible anti-complement activity against the CP. As part of our continued investigation of the components and bio-activities of V. yedoensis and its substituents (Cao et al., Citation2013, Citation2014; Li et al., Citation2012; Qin et al., Citation2013), the aim of the present study was to (1) isolate and identify the potential anti-complement components from V. tiansnaica; (2) evaluate the anti-complement activities of the isolates through the CP and AP; and (3) identify the targets of the active compounds in the complement activation cascade.
Materials and methods
General experimental procedures
Melting points (m.p.) were determined using an X-5 micro-melting point apparatus (Yuhua Instrument Ltd., Gongyi, China). NMR spectra were recorded on a Bruker DRX-400 or 600 spectrometer (Bruker, Fallanden, Switzerland) with TMS as an internal standard. ESI-MS spectra were obtained on an Agilent SL G1946D single quadrupole mass spectrometer (Agilent, Foster, CA). HR-ESI-MS spectra were measured on a Waters Xevo G2 QTOF-MS spectrometer (Waters, Milford, MA). UV spectra were measured by an Agilent 1200 HPLC-DAD system (Agilent, Palo Alto, CA). Column chromatography was carried out on silica gel (200–300 mesh, Qingdao Marine Chemical Factory, Qingdao, China), Sephadex LH-20 (Pharmacia, Uppsala, Sweden), and macroporous resin (Diaion HP-20, Mitsubishi Chemical Corp., Tokyo, Japan). Semi-preparative HPLC separation was performed on an LC3000 system equipped with a P3000 pump, a UV3000 detector (Beijing Chuangxintongheng Science & Technology Co. Ltd., Beijing, China), and a Luna RP-18 column (250 mm × 10 mm, 10 μm, Phenomenex, Torrance, CA).
Plant material
The whole plant of V. tianshanica was collected from the Xinjiang Uygur Autonomous Region, China, in June 2012, and authenticated by Dr. Zhihong Cheng. A voucher specimen (No. 201206TSJC) is deposited at the Department of Pharmacognosy, School of Pharmacy, Fudan University, Shanghai, China.
Animals and reagents for the anti-complement assay
Guinea pigs and New Zealand rabbits were purchased from Laboratory Animals Research Institute of Fudan University (Shanghai, China). Sheep erythrocytes were collected in Alsevers' solution. Anti-sheep erythrocyte antibody was from Prof. Yunyi Zhang (Department of Pharmacology, Fudan University, Shanghai, China). Normal human serum (NHS) was obtained from three healthy adult donors (age 25–30). Suramin sodium salt (the positive control) was purchased from Sigma (St. Louis, MO). Anti-C1q, human (goat), anti-C2, human (goat), anti-C5, human (rabbit), and anti-C9, human (Goat) were obtained from Merck Biosciences (Shanghai, China). Anti-C3, human (goat) and anti-C4, human (Goat) were purchased from Shanghai Sun Biotech Co., Ltd. (Shanghai, China). Buffers: veronal-buffered saline (VBS) containing 0.5 mM Mg2+ and 0.15 mM Ca2+ (GVB2+) for the CP, and VBS containing 2.5 mM Mg2+ and 8 mM EGTA (GVB-Mg-EGTA) for the AP were prepared.
Extraction and isolation
The dried whole plant of V. tianshanica (20 kg) was extracted three times with 70% aqueous ethanol (20 L) at room temperature for 4 h. The ethanol extracts were combined and evaporated to dryness under reduced pressure, yielding a dark green residue (1.2 kg). The residue was suspended in water (2 L) and partitioned successively with petroleum ether (60–90 °C), ethyl acetate, and n-butanol (2 L each) to yield three corresponding extracts. The ethyl acetate extract (280 g) was fractionated on a silica gel column and eluted with increasing polarities of mixture of CH2Cl2 and MeOH to afford six fractions A–F. Fraction A eluted with CH2Cl2–MeOH (50:1) was purified repeatedly on a Sephadex LH-20 column with CH2Cl2–MeOH (1:1) to yield compounds 5 (15 mg) and 17 (30 mg). Fraction B eluted with CH2Cl2–MeOH (30:1) was recrystallized from MeOH to yield 21 (30 mg). Fraction D eluted with CH2Cl2–MeOH (10:1) was also recrystallized from MeOH to obtain a light yellow solid 1 (25 mg), the residue of which was further separated by a Sephadex LH-20 column eluting with CH2Cl2–MeOH (1:1), followed by purification on a semi-preparative HPLC eluted with 19% aqueous MeOH to afford 4 (25 mg), 18 (5 mg), 19 (6 mg), and 20 (10 mg). Fraction E eluted with CH2Cl2–MeOH (5:1) was recrystallized from MeOH to obtain 2 (100 mg). Concentration of the filtrate and purification of the residue by the semi-preparative HPLC with 15% acetonitrile led to the isolation of 3 (10 mg) and 11 (20 mg). Fraction F eluted with CH2Cl2–MeOH (5:1) was repeatedly purified by semi-preparative HPLC with 8% acetontrile to afford 6 (50 mg), 7 (20 mg), 12 (7 mg), 13 (6 mg), and 15 (30 mg), and with 32% MeOH to afford 8 (20 mg) and 9 (13 mg).
The n-butanol extract (200 g) was fractionated by passage through a macroporous resin column with a linear gradient of 0–60% ethanol in water, to yield four fractions. Fraction 2 eluted with EtOH–H2O (20:80) was chromatographed on Sephadex LH-20 (CH2Cl2–MeOH, 1:1) to yield 14 (7 mg). Fraction D eluted with EtOH–H2O (60:40) was purified by the semi-preparative HPLC using 38% methanol as eluent, to yield 10 (50 mg) and 16 (15 mg).
Anti-complement estimation through the classical pathway and the alternative pathway
The anti-complement activity of the isolates against the CP and AP was measured as previously described in detail by our laboratory (Song et al., Citation2014).
Identification of targets on the complement activation cascade
The identification of the targets on the complement activation cascade was also conducted according to the method previously described in our laboratory (Song et al., Citation2014). The concentrations of compounds 1, 16 and 21 were prepared as 0.42, 0.33, and 0.15 mg/mL, respectively, the minimal concentrations to completely inhibit the hemolysis of 1:10 diluted NHS through the CP.
Results and discussion
Repeated column chromatography of the ethyl acetate and n-butanol fractions of the ethanol extract of V. tianshanica led to the isolation of 21 phenolic compounds including 15 flavonol O-glycosides (1–15), one flavone 6,8-di-C-glycoside (16), one flavone aglycone (17), and four phenolic acid derivatives (18–21). All these compounds except 1 and 10 were reported for the first time from V. tianshanica, and compound 16 is the first flavone C-glycoside isolated from the herb. It is reported that the genus Viola L. is a rich source of flavone C-glycosides (Vukics et al., Citation2008; Xie et al., Citation2003). The chemical structures of these isolates are presented in .
Spectral data of isolated compounds
The structural identification of these compounds was mainly achieved by spectroscopic methods (including 1H-, 13C-NMR, MS, and HR-ESI-MS), and comparing their spectral data with those reported in literatures. The 13C-NMR data of 1–15 are shown in .
Table 1. 13C NMR data of compounds 1–15 (400 MHz, DMSO-d6).
Kaempferol 3-O-β-d-glucopyranoside (1): light yellow needles (MeOH); m.p. 173–175 °C; UV (λmax): 265, 343 nm; ESI-MS m/z 449 [M+H]+; HR-ESI-MS m/z 447.0925 [M-H]−; 1H-NMR (400 MHz, DMSO-d6): 12.62 (1H, s, 5-OH), 10.31 (1H, s, 7-OH), 6.21 (1H, d, J = 2.0 Hz, H-6), 6.44 (1H, d, J = 2.0 Hz, H-8), 8.05 (2H, d, J = 8.9 Hz, H-2′, 6′), 6.91 (2H, d, J = 8.9 Hz, H-3′, 5′), 5.48 (1H, d, J = 7.6, H-1′′) (Mei et al., Citation2000).
Kaempferol 3,7-di-O-β-d-glucopyranoside (2): light yellow powders; m.p. 202–205 °C; UV (λmax): 265, 346 nm; ESI-MS m/z 611 [M+H]+; HR-ESI-MS m/z 609.1470 [M−H]−; 1H-NMR (400 MHz, DMSO-d6): 12.60 (1H, s, 5-OH), 10.29 (1H, s, 4′-OH), 6.42 (1H, d, J = 1.8 Hz, H-6), 6.78 (1H d, J = 1.8 Hz, H-8), 8.04 (2H, d, J = 8.7 Hz, H-2′, 6′), 6.88 (2H, d, J = 8.7 Hz, H-3′, 5′), 5.47 (1H, d, J = 7.0 Hz, H-1′′), 5.06 (1H, d, J = 7.2 Hz, H-1′′′) (Xu & Wu, Citation2009).
Kaempferol 3-O-α-l-rhamnopyranosyl(1→6)-β-d-glucopyranoside (3): light yellow powders; m.p. 186–189 °C; UV (λmax): 265, 345 nm; ESI-MS m/z 595 [M+H]+; HR-ESI-MS m/z 593.1508 [M−H]−; 1H-NMR (400 MHz, CD3OD): 6.22 (1H, d, J = 1.4 Hz, H-6), 6.41 (1H, br. s, H-8), 8.08 (2H, d, J = 8.8 Hz, H-2′, 6′), 6.91 (2H, d, J = 8.8 Hz, H-3′, 5′), 5.14 (1H, d, J = 7.4 Hz, H-1′′), 4.54 (1H, s, H-1′′′), 1.14 (3H, d, J = 6.2 Hz, H-6′′′) (Wang et al., Citation2008).
Kaempferol 3,7-di-O-α-l-rhamnopyranoside (4): light yellow powders; m.p. 165–170 °C; UV (λmax): 266, 340 nm; ESI-MS m/z 579 [M+H]+; HR-ESI-MS m/z 577.1555 [M−H]−; 1H-NMR (400 MHz, DMSO-d6): 6.43 (1H, br. s, H-6), 6.76 (1H, br. s, H-8), 7.78 (2H, d, J = 8.5 Hz, H-2′, 6′), 6.90 (2H, d, J = 8.5 Hz, H-3′, 5′), 5.53 (1H, br. s, H-1′′), 1.11 (3H, d, J = 6.1 Hz, H-6′′), 5.27 (1H, br. s, H-1′′′), 0.78 (3H, d, J = 5.9 Hz, H-6′′′) (Gohar & Elmazar, Citation1997).
Kaempferol 3-O-(4′′-O-acetyl-α-l-rhamnopyranosyl)-7-O-α-l-rhamnopyranoside (5): light yellow powders; ESI-MS m/z 621 [M+H]+, 643 [M+Na]+; HR-ESI-MS m/z 619.1696 [M − H]−; 1H-NMR (400 MHz, DMSO-d6): 6.48 (1H, d, J = 2.0 Hz, H-6), 6.80 (1H, d, J = 2.0 Hz, H-8), 7.78 (2H, d, J = 8.8 Hz, H-2′, 6′), 6.95 (2H, d, J = 8.8 Hz, H-3′, 5′), 5.54 (1H, br. s, H-1′′), 0.80 (3H, d, J = 6.0 Hz, H-6′′), 5.58 (1H, br. s, H-1′′′), 1.28 (3H, d, J = 6.0 Hz, H-6′′′), 2.06 (3H, s, OAc) (Wu et al., Citation2012).
Kaempferol 3-O-α-l-rhamnopyranosyl (1→6)-β-d-glucopyranosyl-7-O-β-d-glucopyranoside (6): light yellow powders; UV (λmax): 265, 346 nm; ESI-MS m/z 757 [M+H]+; HR-ESI-MS m/z 755.2066 [M−H]−; 1H-NMR (400 MHz, DMSO-d6): 12.58 (1H, s, 5-OH), 10.21 (1H, s, 4′-OH), 6.45 (1H, d, J = 2.0 Hz, H-6), 6.76 (1H, d, J = 2.0 Hz, H-8), 8.02 (2H, d, J = 8.8 Hz, H-2′, 6′), 6.89 (2H, d, J = 8.8 Hz, H-3′, 5′), 5.36 (1H, d, J = 7.3 Hz, H-1′′), 4.38 (1H, d, J = 1.6 Hz, H-1′′′), 0.96 (3H, d, J = 6.2 Hz, H-6′′′), 5.10 (1H, d, J = 7.8 Hz, H-1′′′′) (Wan et al., Citation2011).
Kaempferol 3-O-β-d-glucopyranosyl(1→2)-β-d-glucopyranosyl-7-O-β-d-glucopyranoside (7): light yellow powders; m.p. 196–198 °C; UV (λmax): 264, 342 nm; ESI-MS m/z 773 [M+H]+; HR-ESI-MS m/z 771.1987 [M−H]−; 1H-NMR (400 MHz, DMSO-d6): 6.44 (1H, d, J = 1.7 Hz, H-6), 6.81 (1H, d, J = 1.7 Hz, H-8), 8.08 (2H, d, J = 8.8 Hz, H-2′, 6′), 6.94 (2H, d, J = 8.8 Hz, H-3′, 5′), 5.71 (1H, d, J = 6.9 Hz, H-1′′), 4.63 (1H, d, J = 7.8 Hz, H-1′′′), 5.09 (1H, d, J = 7.1 Hz, H-1′′′′) (Kim et al., Citation2002).
Kaempferol 3-O-[β-d-glucopyranosyl(1→2)]-α-l-rhamnopyranosyl(1→6)-β-d-glucopyranoside (8): light yellow powders; m.p. 183–184 °C; UV (λmax): 265, 345 nm; ESI-MS m/z 757 [M+H]+; HR-ESI-MS m/z 755.2039 [M−H]−; 1H-NMR (400 MHz, DMSO-d6): 12.63 (1H, s, 5-OH), 6.18 (1H, d, J = 1.6 Hz, H-6), 6.38 (1H, d, J = 1.6 Hz, H-8), 7.99 (2H, d, J = 8.8 Hz, H-2′, 6′), 6.91 (2H, d, J = 8.8 Hz, H-3′, 5′), 5.54 (1H, d, J = 7.0 Hz, H-1′′), 4.59 (1H, d, J = 7.7 Hz, H-1′′′), 4.32 (1H, br. s, H-1′′′′), 0.95 (3H, d, J = 6.0 Hz, H-6′′′′) (Tang et al., Citation2001).
Kaempferol-3-O-[α-l-rhamnopyranosyl(1→2)]-α-l-rhamnopyranosyl(1→6)]-β-d-glucopyranoside (9): light yellow powders; UV (λmax): 265, 345 nm; ESI-MS m/z 741 [M+H]+; HR-ESI-MS m/z 739.2102 [M−H]−; 1H-NMR (400 MHz, DMSO-d6): 12.65 (1H, s, 5-OH), 10.18 (1H, s, 4′-OH), 6.20 (1H, d, J = 1.2 Hz, H-6), 6.41 (1H, d, J = 1.2 Hz, H-8), 7.96 (2H, d, J = 8.7 Hz, H-2′, 6′), 6.88 (2H, d, J = 8.7 Hz, H-3′, 5′), 5.49 (1H, d, J = 7.0 Hz, H-1′′), 5.06 (1H, br. s, H-1′′′), 1.00 (3H, d, J = 6.1 Hz, H-6′′′), 4.33 (1H, br. s, H-1′′′′), 0.81 (3H, d, J = 6.1 Hz, H-6′′′′) (Lu et al., Citation2000).
Isorhamnetin 3-O-β-d-glucopyranoside (10): light yellow powders; m.p. 105–108 °C; UV (λmax): 254, 352 nm; ESI-MS m/z 479 [M+H]+; HR-ESI-MS m/z 477.1031 [M−H]−; 1H-NMR (400 MHz, DMSO-d6): 12.71 (1H, s, 5-OH), 6.22 (1H, s, H-6), 6.46 (1H, d, J = 2.0 Hz, H-8), 7.95 (1H, d, J = 2.0 Hz, H-2′), 6.93 (1H, d, J = 8.5 Hz, H-5′), 7.50 (1H, dd, J = 8.5, 2.0 Hz, H-6′), 5.57 (1H, d, J = 7.1 Hz, H-1′′), 3.86 (3H, s, OCH3) (Feng et al., Citation2008).
Isorhamnetin 3-O-α-l-rhamnopyranosyl(1→6)-β-d-glucopyranoside (11): light yellow powders; m.p. 169–174 °C; UV (λmax): 254, 353 nm; ESI-MS m/z 625 [M+H]+; HR-ESI-MS m/z 623.1615 [M−H]−; 1H-NMR (400 MHz, DMSO-d6): 6.20 (1H, d, J = 1.8 Hz, H-6), 6.40 (1H, s, H-8), 7.95 (1H, d, J = 1.8 Hz, H-2′), 6.92 (1H, d, J = 8.5 Hz, H-5′), 7.63 (1H, dd, J = 8.5, 1.8 Hz, H-6′), 5.23 (1H, d, J = 7.4 Hz, H-1′′), 4.53 (1H, d, J = 0.9 Hz, H-1′′′), 1.11 (3H, d, J = 6.2 Hz, H-6′′′), 3.95 (3H, s, OCH3) (Wu et al., Citation2011).
Isorhamnetin 3-O-α-l-rhamnopyranosyl(1→6)-β-d-glucopyranosyl-7-O-β-d-glucopyranoside (12): light yellow powders; m.p. 144–147 °C; UV (λmax): 254, 353 nm; ESI-MS m/z 787 [M+H]+; HR-ESI-MS m/z 785.2151 [M−H]−; 1H-NMR (400 MHz, DMSO-d6): 6.46 (1H, d, J = 2.0 Hz, H-6), 6.79 (1H, d, J = 1.9 Hz, H-8), 7.88 (1H, d, J = 1.9 Hz, H-2′), 6.93 (1H, d, J = 8.4 Hz, H-5′), 7.56 (1H, dd, J = 8.4, 1.9 Hz, H-6′), 5.47 (1H, d, J = 7.3 Hz, H-1′′), 4.42 (1H, d, J = 0.9 Hz, H-1′′′), 0.98 (3H, d, J = 6.2 Hz, H-6′′′), 5.08 (1H, d, J = 7.4 Hz, H-1′′′′), 3.85 (3H, s, OCH3) (Aquino et al., Citation1987).
Isorhamnetin 3-O-β-d-glucopyranosyl(1→2)-β-d-glucopyranosyl-7-O-β-d-glucopyranoside (13): light yellow powders; UV (λmax): 254, 352 nm; ESI-MS m/z 803 [M+H]+; HR-ESI-MS m/z 801.2086 [M−H]−; 1H-NMR (400 MHz, DMSO-d6): 6.45 (1H, s, H-6), 6.83 (1H, s, H-8), 7.81 (1H, br. s, H-2′), 6.95 (1H, d, J = 7.9 Hz, H-5′), 7.67 (1H, d, J = 7.9 Hz, H-6′), 5.76 (1H, d, J = 7.3 Hz, H-1′′), 5.43 (1H, d, J = 7.3 Hz, H-1′′′), 4.63 (1H, d, J = 6.7 Hz, H-1′′′′), 3.85 (3H, s, OCH3) (Whang & Lee, Citation1999).
Isorhamnetin 3-O-[α-l-rhamnopyranosyl(1→2)]-β-d-glucopyranosyl(1→6)-β-d-glucopyranosyl-7-O-β-d-glucopyranoside (14): light yellow powders; UV (λmax): 254, 353 nm; ESI-MS m/z 949 [M+H]+, 971 [M+Na]+; HR-ESI-MS m/z 947.2681 [M−H]−; 1H-NMR (400 MHz, DMSO-d6): 6.46 (1H, d, J = 2.0 Hz, H-6), 6.80 (1H, d, J = 2.0 Hz, H-8), 7.75 (1H, d, J = 2.1 Hz, H-2′), 6.95 (1H, d, J = 8.4 Hz, H-5′), 7.66 (1H, dd, J = 8.4, 2.1 Hz, H-6′), 5.50 (1H, d, J = 7.7 Hz, H-1′′), 5.06 (1H, br. s, H-1′′′), 0.95 (3H, d, J = 6.2 Hz, H-6′′′), 4.39 (1H, d, J = 7.7 Hz, H-1′′′′), 4.67 (1H, d, J = 7.0 Hz, H-1′′′′′), 3.86 (3H, s, OCH3) (Carotenuto et al., Citation1997).
Rutin (15): light yellow powders; m.p. 184–187 °C; UV (λmax): 256, 363 nm; ESI-MS m/z 611 [M+H]+; HR-ESI-MS m/z 609.1465 [M−H]−; 1H-NMR (400 MHz, DMSO-d6): 12.58 (1H, s, 5-OH), 6.20 (1H, br. s, H-6), 6.40 (1H, br. s, H-8), 7.54 (2H, br. s, H-2′, 6′), 6.86 (1H, d, J = 7.6 Hz, H-5′), 5.34 (1H, d, J = 6.4 Hz, H-1′′), 4.39 (1H, br. s, H-1′′′), 0.99 (3H, d, J = 5.3 Hz, H-6′′′) (Cheng et al., Citation2001).
Apigenin 6,8-di-C-α-l-arabinopyranoside (16): yellow powders; m.p. 164–166 °C; UV (λmax): 216, 272, 336 nm; ESI-MS m/z 535 [M+H]+; HR-ESI-MS m/z 533.1293 [M−H]−; 1H-NMR (400 MHz, 60 °C, DMSO-d6): 13.80 (1H, br. s, 5-OH), 6.84 (1H, s, H-3), 8.20 (2H, s, H-2′, 6′), 6.93 (2H, d, J = 8.6 Hz, H-3′, 5′), 4.67 (1H, d, J = 9.6 Hz, H-1′′), 4.84 (1H, d, J = 9.7 Hz, H-1′′′); 13C-NMR (100 MHz, 60 °C, DMSO-d6): 164.1 (C-2), 102.2 (C-3), 182.3 (C-4), 158.7 (C-5), 108.4 (C-6), 161.5 (C-7), 104.7 (C-8), 154.9 (C-9), 103.4 (C-10), 121.1 (C-1′), 129.4 (C-2′, 6′), 116.0 (C-3′, 5′), 161.2 (C-4′), 74.1 (C-1′′), 69.0 (C-2′′), 74.1 (C-3′′), 69.3 (C-4′′), 70.1 (C-5′′), 74.9 (C-1′′′), 69.2 (C-2′′′), 74.9 (C-3′′′), 68.6 (C-4′′′), 70.9 (C-5′′′) (Xie et al., Citation2003).
Tricin (17): light yellow powders; ESI-MS m/z 331 [M+H]+; HR-ESI-MS m/z 329.0656 [M-H]−; 1H-NMR (400 MHz, DMSO-d6): 6.99 (1H, s, H-3), 6.21 (1H, d, J = 2.0 Hz, H-6), 6.56 (1H, d, J = 2.0 Hz, H-8), 7.33 (2H, s, H-2′, 6′), 3.89 (6H, s, OCH3×2); 13C-NMR (100 MHz, DMSO-d6): 164.6 (C-2), 104.1 (C-3), 182.3 (C-4), 157.8 (C-5), 99.3 (C-6), 164.1 (C-7), 94.7 (C-8), 161.9 (C-9), 104.2 (C-10), 120.9 (C-1′), 104.8 (C-2′, 6′), 148.7 (C-3′, 5′), 140.3 (C-4′), 56.8 (OCH3×2) (Gong et al., Citation2012).
Syringin (18): white powders; m.p. 273–275 °C; ESI-MS m/z 373 [M+H]+; 1H-NMR (600 MHz, CD3OD): 6.77 (2H, s, H-2, 6), 6.57 (1H, d, J = 15.8 Hz, H-7), 6.35 (1H, dt, J = 15.8, 5.6 Hz, H-8), 4.24 (2H, d, J = 5.6 Hz, H-9), 3.88 (6H, s, 3, 5-OCH3), 4.60 (1H, br. s, H-1′); 13C-NMR (150 MHz, CD3OD): 135.2 (C-1), 105.4 (C-2, 6), 154.3 (C-3, 5), 135.8 (C-4), 131.2 (C-7), 130.0 (C-8), 63.5 (C-9), 105.3 (C-1′), 75.7 (C-2′), 77.8 (C-3′), 71.3 (C-4′), 78.3 (C-5′), 62.5 (C-6′), 57.2 (OCH3×2) (Kitajima et al., Citation1998).
3,5-Dimethoxy-4-hydroxybenzyl methanol 4-O-β-d-glucopyranoside (19): white powders; ESI-MS m/z 369 [M+Na]+, 385 [M+K]+; 1H-NMR (600 MHz, CD3OD): 6.72 (2H, s, H-2, 6), 4.54 (2H, s, H-7), 3.87 (6H, s, OCH3×2), 4.86 (1H, d, J = 7.5 Hz, H-1′); 13C-NMR (150 MHz, CD3OD): 135.2 (C-1), 105.6 (C-2, 6), 154.2 (C-3, 5), 139.7 (C-4), 65.1 (C-7), 57.0 (OCH3×2), 105.5 (C-1′), 75.7 (C-2′), 77.7 (C-3′), 71.3 (C-4′), 78.2 (C-5′), 62.7 (C-6′) (Kitajima et al., Citation1998).
Phenethyl O-β-d-glucopyanoside (20): colorless needles (MeOH); ESI-MS m/z 307 [M+Na]+; 1H-NMR (400 MHz, CD3OD): 7.29–7.18 (5H, m, H-2-6), 2.95 (2H, m, H-7), 3.89 (1H, m, H-8a), 3.69 (1H, m, H-8b), 4.32 (1H, d, J = 7.8 Hz, H-1′); 13C-NMR (100 MHz, CD3OD): 140.0 (C-1), 129.4 (C-2, 6), 130.0 (C-3, 5), 127.2 (C-4), 37.2 (C-7), 71.6 (C-8), 104.4 (C-1′), 75.1 (C-2′), 78.1 (C-3′), 71.8 (C-4′), 77.9 (C-5′), 62.8 (C-6′) (Kitajima et al., Citation1998).
3,4,5-Trihydroxybenzoic acid ethyl ester (21): colorless needles (MeOH); m.p. 248–249 °C; ESI-MS m/z 199 [M+H]+, 221 [M+Na]+; 1H-NMR (400 MHz, CD3OD): 7.06 (2H, s, H-2, 6), 4.29 (2H, q, J = 7.2 Hz, H-1′), 1.36 (3H, t, J = 7.2 Hz, H-2′); 13C-NMR (100 MHz, CD3OD): 120.3 (C-1), 108.6 (C-2, 6), 145.7 (C-3, 5), 138.3 (C-4), 167.2 (C-7), 60.2 (C-1′), 13.2 (C-2′) (Kitajima et al., Citation1998).
Anti-complement activity of the isolates
The ethanol extract of V. tianshanica was found to show obvious anti-complement activity (CH50 = 0.026 ± 0.001 mg/mL and AP50 = 0.065 ± 0.006 mg/mL). Bioactivity-directed fractionation of the active ethanol extract led to reveal that the petroleum ether (PE) fraction was the most active fraction with a CH50 value of 0.011 ± 0.002 mg/mL and a AP50 value of 0.018 ± 0.004 mg/mL, as compared with the EtOAc fraction (CH50 = 0.059 ± 0.002 mg/mL and AP50 = 0.098 ± 0.094 mg/mL) and the n-butanol fraction with a marginal effect (CH50 = 0.337 ± 0.063 mg/mL and AP50 = 0.460 ± 0.080 mg/mL). In this study, a detailed phytochemical investigation was performed on the ethyl acetate and n-butanol fractions, resulting in isolation of 17 flavonoids (mostly flavonol O-glycosides) and four phenolic acid derivatives (). All the isolates except 12, 14, 18, and 19 (due to unavailability of sufficient quantities) were tested for their in vitro anti-complement activity against both CP and AP, and the results were summarized in .
Table 2. Anti-complement activity of compounds 1–21 from V. tianshanica through the classical pathway (CP) and alternative pathway (AP)a,b.
Six flavonol glycosides (1–4, 10, and 15) exhibited anti-complement activity with CH50 and AP50 values ranging from 0.113 to 0.536 mg/mL, and from 0.237 to 1.579 mg/mL, respectively. Compounds 5, 7, and 11 had a slight inhibitory effect on the CP and was inactive on the AP. Compounds 6, 8, 9, 13, and 20 were inactive against either CP or AP. The phenolic acid derivative 21 showed good anti-complement activity with a CH50 value of 0.156 mg/mL and an AP50 value of 0.120 mg/mL. The result demonstrated that the anti-complement activity of the flavonol glycosides (1–9) decreased with the increased number of the sugar units. Furthermore, compounds 1 and 3 with a free hydroxyl at C-7 possessed a higher inhibitory effect than their corresponding glycosides 2 and 6, suggesting that an unglycosylated hydroxyl at C-7 might be a determining factor for optimum anti-complement activity.
Target identification of the active isolates on the complement activation cascade
To better understand the anti-complement mechanism of these active phenolic compounds in V. tianshanica, a flavonol O-glycoside (1), a flavone C-glycoside (16), and a phenolic derivative (21) were selected and evaluated in the complement activation cascade using complement-depleted (C-depleted) sera. As shown in , kaempferol 3-O-β-d-glucopyranoside (1) did not regain the hemolytic activities of C1q-, C2-, C4-, and C9-depleted sera. The hemolysis percentages were around or less than 10%, while it did restore hemolysis significantly in C3- and C5-depleted sera (80.79 ± 1.51% and 88.92 ± 2.41%, respectively). The result indicated that 1 interacted with C1q, C2, C4, and C9 components. Similarly, 16 was found to interact with C1q, C4, and C5, while 21 interacted with C1q, C3, C4, and C9 of the complement system (). The target study suggested that different types of compounds might act on the different targets of the complement system.
Figure 2. Targets of kaempferol 3-O-β-d-glucopyranoside (1, A), apigenin 6,8-di-C-α-l-arabinopyranoside (16, B), and 3,4,5-trihydroxybenzoic acid ethyl ester (21, C) on the complement activation cascade. Compounds 1-, 16-, and 21-treated sera were mixed with various complement-depleted (C-depleted) sera, and the capacity to restore the hemolytic capacity of depleted sera by the classical pathway was estimated by adding sheep antibody-sensitized erythrocytes. Cont represents the complement control group. Data are expressed as mean ± SD of triplicate measurements.
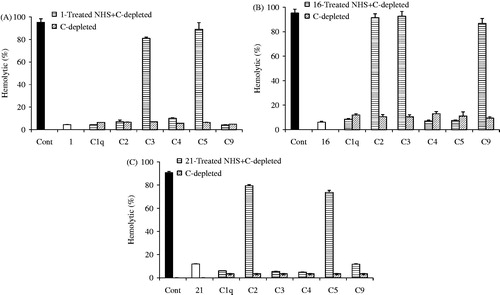
It is generally accepted that the complement system promotes inflammatory factors, indirectly released inflammatory mediators and cytokines (Chen et al., Citation2010). Thus the complement system participates in the inflammatory response and is involved in the pathophysiology of a variety of infectious and non-infectious inflammatory diseases. Therefore, appropriate inhibition of excessive activation of the complement system is an effective way of treating inflammatory diseases. In the present study, phenolic acids were identified as anti-complement components of V. tianshanica. Among these, compounds 1, 3, 10, 15, and 21 showed better anti-complement activity. Therefore, these compounds are plausible candidates for developing potent anti-complement agents.
Conclusion
In this study, 21 phenolic compounds were isolated and identified from the ethanol extract of V. tianshanica. To the best of our knowledge, this is the first report on isolation of these compounds except 1 and 10 from V. tianshanica. Among these, 11 compounds demonstrated anti-complement activity against the classical and alternative pathways. The targets of compounds 1, 16, and 21 on the complement system were also disclosed. Our result shows that these active compounds are plausible candidates for potent anti-complement agents.
Declaration of interest
The authors report that they have no conflicts of interest. The authors are grateful for financial support from the National Natural Science Fund of China (No. 81473421).
References
- Aquino R, Behar I, D'agostino M, et al. (1987). Phytochemical investigation on Mercurialis annua. Biochem Syst Ecol 15:667–9
- Cao J, Qin Y, Yin CL, et al. (2013). Chemical constituents of Viola yedoensis and their antioxidant activity. Chin J Exp Tradit Med Form 19:282–6
- Cao J, Yin CL, Qin Y, et al. (2014). Approach to the study of flavone di-C-glycosides by high performance liquid chromatography-tandem ion trap mass spectrometry and its application to characterization of flavonoid composition in Viola yedoensis. J Mass Spectrom 49:1010–24
- Carotenuto A, Fattorusso E, Lanzotti V, et al. (1997). The flavonoids of Allium neapolitanum. Phytochemistry 44:949–57
- Chen M, Muckersie E, Luo C, et al. (2010). Inhibition of the alternative pathway of complement activation reduces inflammation in experimental autoimmune uveoretinitis. Eur J Immunol 40:2870–81
- Cheng YX, Zhou J, Tan NH. (2001). The chemical constituents of Parakmeria yunnanensis. Acta Bot Yunn 23:352–6
- Editorial Committee of Chinese Materia Medica. (2005). Chinese Material Medica Uygur Medicine Volume. Shanghai, China: Shanghai Science and Technology Press
- Feng SX, Liu MF, Wei XY, et al. (2008). Triterpenoids and flavonoids from Microcos paniculata. J Trop Subtrop Bot 16:51–6
- Gohar AA, Elmazar MMA. (1997). Isolation of hypotensive flavonoids from Chenopodium species growing in Egypt. Phytother Res 11:564–7
- Gong JY, Mao JW, Huang WS, et al. (2012). Study on preparation of tricin reference substance by semi-preparative HPLC in Bambusae Caulis in Taenias. Chin Tradit Herbal Drugs 43:919–21
- Kim JE, Jung MJ, Jung HA, et al. (2002). A new kaempferol 7-O-triglucoside from the leaves of Brassica juncea L. Arch Pharm Res 25:621–4
- Kirschfink M. (1997). Controlling the complement system in inflammation. Immunopharmacology 8:51–62
- Kitajima J, Ishikawa T, Tanaka Y, et al. (1998). Water-soluble constituents of fennel. V. Glycosides of aromatic compounds. Chem Pharm Bull 46:1587–90
- Li W, Xie JY, Li H, et al. (2012). Viola yedoensis liposoluble fraction ameliorates lipopolysaccharide-induced acute lung injury in mice. Am J Chin Med 40:1007–18
- Lu Y, Sun Y, Foo LY, et al. (2000). Phenolic glycosides of forage legume Onobrychis viciifolia. Phytochemistry 55:67–75
- Lustep HL, Clark WM. (2001). Current status of neuroprotective agents in the treatment of acute ischemic stroke. Curr Neurol Neurosci Rep 1:13–18
- Ma XM, Zhou XY, Zhang L, et al. (2004). Antimicrobial test in vitro of extracts from Viola tianshanica Maxim. Lishizhen Med Mater Med Res 15:470–1
- Makridea SC. (1998). Therapeutic inhibition of the complement system. Pharmacol Rev 50:59–87
- Mei WL, Yong Y, Ni W, et al. (2000). Flavonoids from Knema globularia. Acta Bot Yunn 22:358–60
- Peiris JSM, Chu CM, Cheng VCC, et al. (2003). Clinical progression and viral load in a community outbreak of coronavirus-associated SARS pneumonia: A prospective study. Lancet 361:1767–72
- Qin Y, Yin CL, Cheng ZH. (2013). A new tetrahydrofuran lignan diglycoside from Viola tianshanica Maxim. Molecules 18:13636–44
- Shen XY, Xie CX. (2009). Study on antioxidantive activity of extracts from Viola tianshanica Maxim. Food Sci 30:139–41
- Shen XY, Xie CX, Deng WP. (2009a). Analysis of volatile oil from Viola tianshanica Maxim. by GC/MS. J Chin Mass Spectrom Soc 30:51–4
- Shen XY, Xie CX, Liu D. (2009b). Study on antimicrobial activity of Viola tianshanica Maxim. extracts. Biotechnology 19:71–3
- Song WH, Cheng ZH, Chen DF. (2014). Anticomplement monoterpenoid glucosides from the root bark of Paeonia suffruticosa. J Nat Prod 77:42–8
- Tang YP, Lou FC, Wang JH. (2001). Two kaempferol triglycosides from pericarps of Sophora japonica L. China J Chin Mat Med 26:839–41
- Vukics V, Ringer T, Kery A, et al. (2008). Analysis of heartsease (Viola tricolor L.) flavonoids by micro-liquid chromatography coupled to multistage mass spectrometry. J Chromatogr A 1206:11–20
- Wan C, Yu Y, Zhou S, et al. (2011). Isolation and identification of phenolic compounds from Gynura divaricata leaves. Pharmacogn Mag 7:101–8
- Wang YS, Huang R, Li L, et al. (2008). Flavonoids from Litsea glutinosa. Chin Tradit Herbal Drugs 39:1466–8
- Whang WK, Lee MT. (1999). New flavonol glycosides from leaves of Symplocarpus renifolius. Arch Pharm Res 22:423–9
- Wu JM, Zhang Y, Zhang HY, et al. (2012). NMR numerical analysis of sutchuenoside A. J Zhengzhou Univ 33:68–71
- Wu W, Wang CZ, Li X, et al. (2011). Chemical constituents of antitumor active fraction of Lysimachia clethroides. Chin Tradit Herbal Drugs 42:38–41
- Xiang B, Du GH, Wang XC, et al. (2010). Elucidating the structure of two cyclotides of Viola tianshanica Maxim. by MALDI TOF/TOF MS analysis. Acta Pharm Sin 45:1402–9
- Xie C, Veitch NC, Houghton PJ, et al. (2003). Flavone C-glycosides from Viola yedoensis Makino. Chem Pharm Bull 51:1204–7
- Xu L, Wu D. (2009). Chemical constituents of aerial parts of Asarum heterotropides Fr. Schmidt var. mandshuricum (Maxim.) Kitaga (2). J Shenyang Pharm Uinv 26:964–6
- Yang J, Yang H, Chen LQ, et al. (2011). A study on extraction technology and anti-inflammatory effect of essential oil of Viola tianshanica. J Hubei Univ Nat 28:5–7
- Yu JD, Dai Z, Lin RC. (2009). Chemical constituents of Viola tianshanica. China J Chin Mat Med 34:2916–17