Abstract
Context: Polyscias fruticosa (L.) Harms (Araliaceae) is used as a traditional remedy for asthma in Ghana.
Objective: The objective of this study is to establish the anti-asthmatic property and a possible mode of activity of an ethanol leaf extract of P. fruticosa (PFE).
Materials and methods: The time (min) for pre-convulsive dyspnea, and time for recovery, after sensitization with 150 μg OVA and induction of bronchospasm with 1% acetylcholine or histamine in normal, and 100, 250, and 500 mg/kg PFE-treated Dunkin Hartley guinea pigs, were recorded. Atropine (0.1 mg), mepyramine (0.1 mg), and PFE (1 mg) effect on a contractile response of 2.0 × 10−2 μg/ml acetylcholine and 5.8 × 10−2 μg/ml histamine on the isolated guinea pig ileum was investigated. Cytological and histological studies were conducted using guinea pig peritoneal mast cells and mesenteric cells, respectively, to establish PFE effect on compound 48/80-induced mast cell degranulation.
Results: PFE (100–500 mg/kg) prolonged the onset of pre-convulsive dyspnea by 76.1–180.2% (p ≤ 0.01–0.001), and decreased recovery time by 71.9–78.5% (p ≤ 0.01–0.001). It also enhanced percentage protection against histamine-induced bronchospasm by 15.8–80.1-fold (p ≤ 0.05–0.01), and decreased percentage recovery time 2.5–3.3-fold (p ≤ 0.05–0.01). PFE significantly inhibited (60.4 ± 8.3%) contractile responses of histamine and produced significant inhibition (56–79%: p ≤ 0.001) of mast cell degranulation.
Conclusion: PFE has anti-asthmatic, antihistaminic, and mast cell stabilization effect making it useful in traditional asthma management.
Introduction
Asthma is an inflammatory disease of the lungs associated with bronchial hyperactivity, bronchospasm, mucus secretion, edema, and cellular infiltration (Global Initiative for Asthma, Citation2013). It is associated with frequent episodes of wheezing dyspnea with chronic airway narrowing and chest tightness, thus causing chronic respiratory impairment (Weinberger & Abu-Hasan, Citation2007).
The global community has seen a significant rise in the threat of asthma over the past decades (Braman, Citation2006), with interesting alerts of bronchial asthma rating third after asbestosis and tuberculosis (Alexopoulos et al., Citation2011; Moucaut et al., Citation2013); hence, the expression asthma endemicity (Mazaik, Citation2005). Asthma is the most common chronic disease among children and also affects millions of adults. It can be fatal and causes an estimated 250 000 deaths annually, one in 250 deaths worldwide (Anonymus, Citation2011). In Ghana, the World Health Organization (WHO) estimates an asthma country incidence rate of 1.5/1000 per year (Amoah et al., Citation2012; WHO, Citation2009).
Pathologically, asthma is characterized by eosinophilic and lymphocytic inflammation of the bronchial mucosa with deposition of collagen beneath the epithelium and hyperplasia of the cells of all structural elements. In early asthmatic response, immunoglobulin E produced by allergens present binds to mast cells in the airway mucosa resulting in antigen–antibody interaction. This triggers the release of mediators such as histamine, tumor necrotic factor (TNF)-α, chymase, prostaglandins, leukotrienes C4 and D4, interleukins, prostaglandin D2, tryptase, and amphiregulin following degranulation (Brunton et al., Citation2008). When released, the mediators diffuse throughout the airway mucosa triggering the smooth muscle contraction, vasodilation, increased vascular permeability (causing tissue swelling), contraction of bronchial smooth muscle (causing airflow obstruction), and increased mucus secretion contributing to airflow obstruction in the lower airways (Galli et al., Citation2008).
The late asthmatic response which develops 6–9 h after allergen exposure is characterized by the recruitment, activation, and migration of eosinophils, lymphocytes, neutrophils, and macrophages into the lamina propria, epithelium, and the airway lumen (Galli et al., Citation2008). The cytokines especially interleukins 5, 9, and 13, chemokines, and growth factors produced during the early asthmatic response drive this process. This allows for further release of mediators and further bronchoconstriction. The cytokines are thought to attract and activate eosinophils, stimulate IgE production by B lymphocytes, and directly aid mucus production by the bronchial epithelial cells (Brightling et al., Citation2002; Miller, Citation2001).
Eosinophils are the predominant cell type during the late asthmatic response to allergens, with their numbers peaking 24 h after allergen challenge (Smith & Broadley, Citation2007; Toward & Broadley, Citation2004). Eosinophils release many biological products including eicosanoids, oxygen-free radicals, Th2 cytokines, growth factors, and toxic granule products which contribute to contraction of smooth muscle, airways hyper responsiveness and increased vascular permeability (Bousquet et al., Citation2000). Granule products such as major basic protein, eosinophil cationic protein, and eosinophil-derived neurotoxin contribute directly to the development of airways hyper responsiveness and epithelial shredding (Gleich et al., Citation1993). Other products such as growth factors, metalloproteases, and elastase are involved in fibrosis and tissue remodeling (Lagente et al., Citation2005).
Although the pathogenesis of asthma is clearly understood, treatment is still a problem, as medications such as corticosteroids, anti-leukotrienes, and monoclonal immunoglobulin E (IgE) inhibitors which are ideal for treating immunologically mediated aspect of asthma are costly and thus unaffordable for individuals earning lower income, who unfortunately are in the majority in Ghana.
With the increased burden of asthma on individual and national economies culminating from exorbitant drug price, drug unavailability, and unaffordability, it is just right that readily available sources of anti-asthma drugs are scientifically characterized not to just add to scientific knowledge but to serve as cheaper alternatives or adjuncts to conventional anti-asthma drugs. There is growing body of reports regarding the use of medicinal plants by many tribes to manage asthma and other inflammatory disorders (Eisenberg et al., Citation1998). The dried root tuber of Rehmannia glutinosa Libosch (Scrophulariaceae); the rhizomes of Alisma orientale (Sam.) Juzepcz (Alismataceae), Dioscorea opposite Thunb (Dioscoreaceae), and Atractylodes ovata (Asteraceae/Compositae); the root bark of Paeonia suffruticosa Andr (Paeoniaceae); the root of Glycyrrhiza glabra (Leguminosae) and Zingiber officinale (Zingiberaceae); the seeds of Lablab purpureus (Fabaceae), Coix lacryma-jobi (Poaceae), and Psoralea corylifolia (Fabaceae); the fruits of Lotus fruit, Schisandra chinensis (Schisandraceae) and Ziziphus jujube Mill (Rhamnaceae), Ophiopogon japonicas tuber (Asparagaceae); the peel of Citrus aurantifolia (Rutaceae), and many more have all being used in the preparation of various drugs for the management of asthma in various parts of the world (Clark et al., Citation2010).
Polyscias fruticosa (L.) Harms (Araliaceae), commonly called the tea tree, is one plants used traditionally in the management of asthma in Ghana. This plant has significant anti-inflammatory property and is safe to use: the NOAEL being less than 1000 mg/kg (Koffuor et al., Citation2014). The ethanol leaf extract of P. fruticosa (L.) Harms has been found to contain glycosides (saponins and cyanogenetic), alkaloids, and sterols (Koffuor et al., Citation2014; Vo et al., Citation1998) which augurs well for anti-inflammatory activity. Polyscias fruticosa is also reported to have analgesic, anti-inflammatory, antitoxin, molluscicidal, diuretic, and antibacterial activities (Abayaneh & Mahendra, Citation2013; Varadharajan & Rajalingam, Citation2011; Bensita et al., Citation1998).
This study, therefore, sought to establish the anti-asthmatic property of an ethanol leaf extract of P. fruticosa and its possible mode of activity using in vivo and in vitro guinea pig models.
Materials and methods
Plant collection and authentication
The leaves of P. fruticosa were collected from Anwomaso (6.7000° N, 1.5333° W), a suburb of the Kumasi Metropolis in the Ashanti Region of Ghana, in December, 2013 The plant was identified and authenticated by Dr. George Henry Sam at the herbarium unit of the Department of Herbal Medicine of the Faculty of Pharmacy and Pharmaceutical Sciences, KNUST, Kumasi, Ghana, where a voucher specimen (KNUST/HM/13/W010) has been deposited.
Preparation of the ethanol leaf extract of P. fruticosa (PFE)
Leaves of P. fruticosa were washed, shade-dried (26–30 °C), and pulverized into fine powder using a mechanical blender. A 3.0 kg quantity of the powder was soaked in 1 L of absolute ethanol in a volumetric flask on a rotary mixer for 72 h. The filtrate obtained after extraction was concentrated in a rotary evaporator (Rotavapor R-210, Buchi, Flawil, Switzerland). The residue (58.2 g; yield: 1.94%) obtained was dried in a desiccator, labeled PFE, and stored at 4 °C in a refrigerator for use in this study.
Chemicals and drugs
Acetylcholine bromide, histamine dihydrochloride, atropine sulphate, mepyramine maleate (Sigma Chemical Co., St. Louis, MO), ovalbumin (Central Drug House, Pvt. Ltd., Delhi, India), sodium cromoglycate (Ashford Laboratory Ltd., Macau, Macau), prednisolone, chlorpheniramine maleate (Letap Pharmaceuticals Ltd, Accra, Ghana), and ketotifen fumarate (Akorn, Inc., Decatur, IL) were the main chemicals and drugs used in this study.
Experimental animals
Healthy male Dunkin Hartley guinea pigs (400–500 g) were used for the study. They were housed in sanitized aluminum cages (70 × 42 × 28 cm) with a base dressing of sawdust as bedding, fed on commercial pellet diet (Agricare Ltd, Kumasi, Ghana), and kept under ambient conditions of temperature (26 ± 4 °C), relative humidity (60 ± 5%) and light/dark cycle.
Ethical considerations
Laboratory study was carried out in a level 2 biosafety laboratory. Protocols for the study were approved by the Committee on Animal Research, Publication and Ethics (CARPE). All activities during the studies conformed to accepted principles for laboratory animal use and care (EU directive of 1986: 86/609/EEC). All the technical team observed all institutional biosafety guidelines for protection of personnel and laboratory
Dosing of drugs to experimental animals
Dosing of PFE, selected based on its known traditional usage, was by oral gavage. Individual dose volumes were calculated based on the animal’s most recent recorded body weight. The oral route of administration was used because it is the usual human exposure route.
Acetylcholine-induced bronchoconstriction
This is an experimental model to determine anti-asthmatic as well as in vivo anti-muscarinic activity (Karol, Citation1994). Twenty guinea pigs weighing between 400 and 500 g were sensitized by intra-peritoneal injection of 1 ml of a solution of 150 μg of ovalbumin (OVA) and 100 mg of alum in normal saline, once a week for 2 weeks. On day 15, the animals were put into five groups (n = 4) and a booster injection of the alum–ovalbumin mixture was administered again to complete the sensitization phase. The challenge phase began 24 h after the sensitization phase. Here, the animals were exposed to 1% aerosolized acetylcholine bromide in an air-tight plexiglass chamber (24 × 14 × 24 cm) after pretreatment of a group with 2 ml/kg normal saline (vehicle), 10 mg/kg prednisolone, or 100, 250, or 500 mg/kg PFE. The time taken for pre-convulsive dyspnea (PDT), i.e., the time of aerosol exposure to the onset of difficulty in breathing leading to the appearance of convulsions, and the time for recovery (RT) i.e., the time animals with pre-convulsive dyspnea, were placed in fresh air to recover, in the OVA-sensitized but treated animals were then recorded and compared with that obtained for a non-sensitized and non-treated group subject to acetylcholine aerosol.
Histamine-induced bronchoconstriction
The bronchodilatory activity as well as the in vivo antihistaminic activity of PFE was studied in guinea pigs using histamine-induced bronchospasm model (Armitage et al., Citation1961; Sarpate et al., Citation2009). Twenty guinea pigs which have been fasted for 24 h were put into five groups (A–E). Animals in each group were exposed to 1% histamine aerosol using a nebulizer at a pressure of 300 mmHg in an air-tight plexiglass chamber (24 × 14 × 24 cm). This caused progressive signs of difficulty in breathing leading to asphyxia. The time of onset of asphyxia (PAT) was recorded. The time taken for each animal to fully recover was also measured as the recovery time (RT). After 24 h, guinea pigs in the various groups were treated with 2 ml/kg normal saline (vehicle), 2 mg/kg chlorpheniramine, or 100, 250, and 500 mg/kg of PFE. Two hours thereafter the animals were subjected to histamine aerosol, and PAT and RT determined. The percentage protection offered by the treatment against asphyxia was calculated, using the formula:
where T1 = PAT before drug treatment, and T2 = PDT after pre-treatment with reference or test drugs. The percentage recovery time was also calculated similarly.
Determination of site of action of PFE
This study was carried out as described by Koffuor et al. (Citation2012). A piece of an isolated guinea pig ileum (about 2.0 cm) was mounted in 40 ml Tyrode physiological solution in a Harvard tissue bath (Harvard Apparatus Ltd, Kent, UK) maintained at 32 °C. The tissue was aerated, and allowed to stabilize in the bath for 15 min. With a contact time of 30 s, a time cycle of 1 min, and a Harvard kymograph (Harvard Apparatus Ltd, Kent, UK) speed of 4 mm/min, a complete dose–response tracing was generated for acetylcholine (1.25 × 10−3 − 0.16 μg) and histamine (2.5 × 10−3 − 0.32 μg). Sub-maximal doses for agonists that gave about 70% of the agonist’s maximum response (i.e., 2.0 × 10−2 μg and 5.8 × 10−2 μg, respectively) were selected and response again obtained for inhibition by their standard antagonists, atropine (0.1 mg) and mepyramine (0.1 mg), respectively. The procedure was repeated for acetylcholine and histamine in the presence of PFE (1.0 mg). The percentage inhibition of the agonists by the antagonists was calculated as follows:
where Y is the submaximal agonist dose response, and X is the response of the submaximal doses of the agonist in the presence of the antagonist.
Mast cell stabilization effect (cytological study)
This study was carried out as previously described by Parmar et al. (Citation2010) with some modification. A guinea pig was sacrificed by cervical dislocation and immediately injected with Tyrode solution into the peritoneal cavity, with gentle massage in this region for 90 s, to facilitate cell recovery. A midline incision was made to expose the peritoneum. The pale peritoneal fluid was aspirated using a blunted plastic Pasteur pipette and collected in plastic centrifuge tube. The fluid was centrifuged at 1000 rpm for 15 min and the supernatant was discarded to obtain reveal a pale cell pellet. The cell pellets were resuspended in fresh Tyrode solution and re-centrifuged, discarding the supernatant. The aliquots of cell pellets were put into groups and treated as follows:
Test tube 1: no treatment (CON)
Test tube 2: 20 μg/ml sodium cromoglycate (DSCG)
Test tube 3: 125 μg/ml PFE
Test tube 4: 250 μg/ml PFE
Test tube 5: 500 μg/ml PFE
The tubes were incubated at 37 °C for 10 min. Compound 48/80 (0.1 ml, 10 μg/ml) was added to each tube and again incubated for 10 min at 37 °C. The cells were carefully spread over glass slides, fixed in 95% ethanol, and stained with 1% toluidine blue for 3 min. The stain was washed off, slides dried in air, and mounted using DPX (Distrene, Plasticiser, Xylene) and finally cover slipped. The mast cells were counted using Leica DM 750 microscope (Leica Microsystems CM5 GmbH, Wetzlar, Germany) from randomly selected high power objective fields (40× magnification). The percent degranulation of the mast cells was calculated by counting the number of degranulated mast cells from total of at least 100 mast cells counted. Percent inhibition of mast cell degranulation for each treatment was then calculated by the following formula:
DMC is the number of degranulated mast cells and TMC is the total number of mast cells
Mast cell stabilization effect (histological study)
This study was carried out as previously described by Gohil and Mehta (Citation2011) with some modification. The animal was sacrificed and pieces of mesentery from the ileum were collected. The pieces were put into Petri dishes containing Tyrode solution and treated as follows:
Petri dish 1: no treatment (CON)
Petri dish 2: 20 μg/ml sodium cromoglycate (DSCG)
Petri dish 3: 10 μg/ml ketotifen (KET)
Petri dish 4: 125 μg/ml PFE
Petri dish 5: 250 μg/ml PFE
Petri dish 6: 500 μg/ml PFE
Each Petri dish was incubated at 37 °C for 15 min. Compound 48/80 (0.1 ml, 10 μg/ml) was added to each Petri dish and again incubated for 10 min at 37 °C. All the pieces were placed in 10% buffered formalin solution. The tissues were processed and sectioned to obtain thin sections. The sections were then mounted on slides and stained with toluidine blue for 3 min. The slides were washed under tap water for 2 h to enhance the metachromatic staining of the mast cells by the toluidine blue. The slides were dipped in xylene, followed by the addition of DPX and finally cover-slipped. The mast cells were counted using Leica DM 750 microscope (Leica Microsystems CM5 GmbH, Wetzlar, Germany) from randomly selected high-power objective fields (40× magnification). The percent degranulation of the mast cells was calculated by counting the number of degranulated mast cells from total of at least 100 mast cells counted. Percent inhibition of mast cell degranulation for each treatment was then calculated as shown in the section above.
Statistical analysis
Data obtained were expressed as mean ± SEM. Significant differences in measured parameter were done using paired t-test (two-tailed) or one-way analysis of variance (ANOVA) with Bonferroni’s multiple comparison test (post hoc test) in Graph-Pad Prism for Windows Version 5.0 (Graph-Pad Software, San Diego, CA). p ≤ 0.05 was regarded as statistically significant.
Results
Acetylcholine-induced bronchoconstriction
There was a significant increase in the time taken for acetylcholine-induced pre-convulsive dyspnea by 76.1–180.2% (from 1.97 ± 0.08 min to between 3.47 ± 0.15 and 5.52 ± 0.17 min: p ≤ 0.01–0.001), and significant decrease in recovery time after pre-convulsive dyspnea by 71.9–78.5% (from 2.7 ± 0.09 min to between 0.76 ± 0.13 and 0.58 ± 0.03 min: p ≤ 0.01–0.001), in animals treated with PFE (100–500 mg/kg), and prednisolone compared with the normal animals (non-sensitized, non-treated). However, there was a significant reduction (p ≤ 0.001) in time taken for pre-convulsive dyspnea in vehicle-treated OVA-sensitized animals and a significant increase (p ≤ 0.001) in recovery time compared to normal animals ().
Figure 1. Effect of pre-treatment of OVA-sensitized guinea-pigs with 2 ml/kg normal saline (NS), 10 mg/kg prednisolone (PRED), and 100, 250, or 500 mg/kg PFE on (A) time taken for acetylcholine-induced pre-convulsive dyspnea (PDT), and (B) recovery time after pre-convulsive dyspnea (RT). Values plotted are means ± SEM (n = 4). Comparing drug-treated OVA-sensitized animals with non-sensitized, non-treated (NSNT): **p < 0.01; ***p < 0.001. Comparing vehicle-treated OVA-sensitized animals with NSNT: ††p < 0.01; †††p < 0.001.
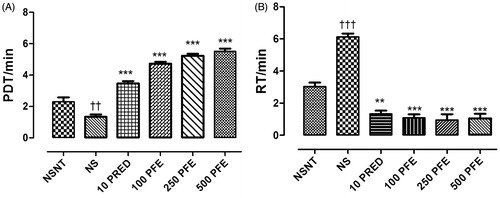
Histamine-induced bronchoconstriction
PFE (100–500 mg/kg) showed a dose-dependent increase in percentage protection against histamine-induced bronchospasm by 15.8–80.1-fold (i.e., from −0.55 ± 3.45% to between 8.12 ± 2.66 and 43.58 ± 11.12%: p ≤ 0.05–0.01) compared with the control. This significant effect was comparable with chlorpheniramine (p ≤ 0.001). The percentage recovery time after histamine-induced bronchospasm for PFE treatments dose dependently decreased 2.5–3.3-fold (i.e., from 41.6 ± 10.45% to between −61.4 ± 20.31 and −95.78 ± 24.58%: p ≤ 0.05–0.01). Chlorpheniramine showed the highest percentage recovery time (−178.3 ± 38.09%: p ≤ 0.001) ().
Figure 2. The effect of 2 ml/kg normal saline (NS), 2 mg/kg chlorpheniramine (CPM), and 100, 250, and 500 mg/kg PFE on (A) percentage protection and (B) recovery time, in histamine-induced bronchospasm in guinea pigs, 2 h post-drug treatment. Values plotted are means ± SEM (n = 4). ns p > 0.05, *p ≤ 0.05, **p ≤ 0.01, ***p ≤ 0.001.
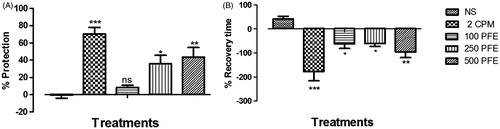
Site of action of PFE
Acetylcholine- and histamine-produced graded dose–responses on a guinea pig ileum were obtained after applying various doses of acetylcholine and histamine (). Atropine produced very significant (p ≤ 0.001) inhibition (68.37 ± 0.83%) of the selected sub-maximal dose of acetylcholine compared with PFE (12.47 ± 3.77%). The selected submaximal dose of histamine was significantly inhibited by PFE (60.4 ± 8.32%) in a manner similar to (p > 0.05) mepyramine (81.6 ± 5.06%) ().
Figure 3. Log dose-response curves for acetylcholine (1.25 × 10−3 – 0.16 μg) and histamine (2.5 × 10−3 – 0.32 μg) on an isolated guinea pig ileum.
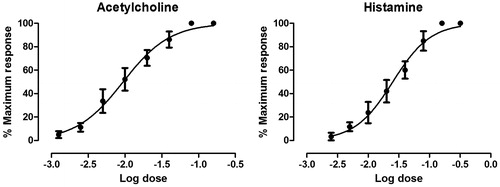
Figure 4. Percentage inhibition of the contractile effect of 2.0 × 10−2 μg acetylcholine, and 5.8 × 10−2 μg histamine on an isolated guinea pig ileum in the presence of 0.1 mg atropine, 0.1 mg mepyramine, and 1 mg PFE. Values plotted are means ± SEM (n = 3). ns p > 0.05, ***p ≤ 0.001 (one-way ANOVA followed by Dunnett's post-hoc test).
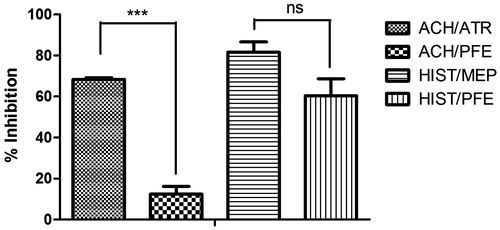
Cytological studies
Compound 48/80 produced mast cell degranulation (93.0 ± 0.92%) of the guinea pig peritoneal cells. Pre-treatment of the peritoneal mast cells with 125, 250, and 500 μg/ml of PFE, and sodium cromoglycate produced 74.24 ± 0.59, 74.32 ± 0.74, 57.71 ± 0.61, and 78.60 ± 1.07% inhibition of the compound 48/80-induced mast cell degranulation, respectively ().
Figure 5. The effects of “no treatment” (CON), 20 μg/ml sodium cromoglycate (DSCG), 10 μg/ml ketotifen (KET), and 125, 259, and 500 μg/ml PFE on compound 48/80 induced (A) peritoneal tissue mast cells degranulation and (B) mesenteric tissue mast cell degranulation. Bars plotted are mean ± SEM (n = 5). ***p < 0.001 (one-way ANOVA followed by Dunnett’s post-hoc test).
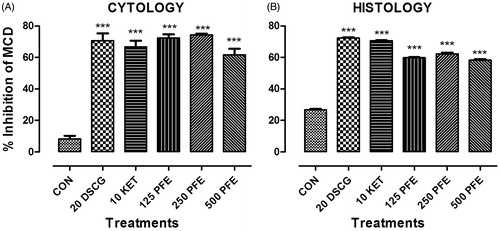
Histological studies
Compound 48/80 produced significant 73.14 ± 0.4% mast cell degranulation in the guinea-pig mesenteric tissue. Pre-treatment of the mesenteric tissue with 125, 250, and 500 μg/ml PFE produced 59.74 ± 0.4, 62.20 ± 0.9, and 58.20 ± 0.7% inhibition of compound 48/80-induced mast cell degranulation, respectively. Sodium cromoglycate and ketotifen produced 72.24 ± 0.5, and 70.60 ± 0.2% inhibition, respectively (). Sample microscopic pictures of fields showing intact and degranulated mast cell from the cytological and histological studies are shown in .
Discussion
This study attempts to establish the anti-asthmatic property of an ethanol leaf extract of P. fruticosa, and its possible mode of activity. Dunkin Hartley guinea pigs were used in this study because the anatomy of their airway, their response to inflammatory mediators, and their anaphylactic bronchoconstriction on antigen challenge is similar to humans (Ricciardolo et al., Citation2008). The extreme sensitivity of their airways to acetylcholine and histamine in causing bronchoconstriction is also very prominent.
In sensitization of the animals, ovalbumin (OVA) was used (Smith & Broadley, Citation2007). Guinea pigs actively sensitized with ovalbumin provide a well-established animal model that has been used extensively for studying the pathogenic mechanisms and therapeutic treatments of bronchial hyper-responsiveness; because many of their pathophysiological features closely resemble those seen in asthmatic patients (Wu et al., Citation2000). Ovalbumin also induces asthma in humans and shows an ability to induce chronic airway inflammation (Bousquet et al., Citation2000). Moreover, OVA is a T-cell-dependent antigen which is non-toxic and inert (Zhang et al., Citation1997), thus making it appropriate for the study.
Acetylcholine and histamine were used as spasmogenic agents to induce pre-convulsive dyspnea. Acetylcholine on the muscarinic receptors causes bronchoconstriction of the airway smooth muscle and mucus secretion from the goblet cells of the mucous bronchial glands in the medium-to-large airways by acting on M1, M2, and/or M3 receptors. The fact that PFE abolished pre-convulsive dyspnea induced by acetylcholine gives a possibility that PFE could be an anti-muscarinic agent; which needed verification. Histamine is the best-known mediator of bronchoconstriction; acting through H1-receptor stimulation on the airway smooth muscle is the most implicated mediator in bronchoconstriction in asthma (Kalpana et al., Citation2011; Rang & Dale, Citation2007). PFE and chlorpheniramine (H1 receptor antagonist) also abolished histamine-induced bronchoconstriction (by prolonging the onset of pre-convulsive dyspnea) suggesting antihistaminic effect.
PFE gave an outstanding longer time for the development of pre-convulsive dyspnea for all dose levels; effects were similar to that of prednisolone. Prednisolone is a steroid with good anti-inflammatory activity. It causes the inhibition of gene transcription for COX-2, cytokines, cell adhesion molecules, and inducible NO synthase; all of which contribute to inflammation in asthma.
In order to verify anti-muscarinic or anti-histaminic property of PFE, the effect of the extract was investigated on the contractility of acetylcholine and histamine on the isolated guinea pig ileum preparation. The isolated guinea pig ileum is a low tone tissue which has muscarinic receptor subtypes M2 and M3, and histaminic receptor subtypes H1, H2, and H3 (Bertaccini & Coruzzi, Citation1995; Leurs et al., Citation1991). Histamine, mediated by the H1 receptor subtype, contracts the longitudinal muscle in the guinea pig ileum. These contractions can be antagonized by mepyramine (Trzeciakowski, Citation1987; Zavecz & Yellin, Citation1982), a competitive H1 receptor blocker. The significantly high percentage antagonism of both mepyramine and PFE suggested H1 receptor blockade and hence PFE possessing antihistaminic effect similar to mepyramine.
Acetylcholine mediates contractions of the guinea pig ileum by activating M3 receptors on the longitudinal muscles. The significant inhibitory effect by atropine but not by PFE, therefore, indicates that PFE does not have significant antimuscarinic activity. The significant reduction in pre-convulsive dyspnea induced by acetylcholine in the OVA-sensitized guinea pigs by PFE could, therefore, not be a result of significant antimuscarinic activity.
After ovalbumin sensitization, there is bronchial hyperactivity (tachykinins involved) which leads to bronchospasm, and inflammatory effect such as mucus secretion, edema, and cellular infiltration, on exposure to an antigen (e.g., acetylcholine), a condition similar to asthma. This hyper-responsiveness is mediated by histamine and the leukotrienes. The abolishing of acetylcholine-induced pre-convulsive dyspnea/bronchospasm after sensitization is, therefore, indicative of an anti-asthmatic property of PFE, attributable to antihistaminic and possible anti-inflammatory property of PFE. Bronchial inflammation causes narrowing due to edema and swelling caused by an immune response to an allergen. Anti-inflammation will, therefore, decrease significantly the time for pre-convulsive dyspnea.
Asthma is well managed if a drug has both antihistamine and mast cell-stabilizing effects. PFE, sodium cromoglycate, and ketotifen had significant mast cell stabilization effect. Mast cells are the primary responders in allergic reactions, triggered by cross-linking of a high affinity IgE receptor (FCεRI) (Gohil & Mehta, Citation2011). When mast cells are activated by an allergen that binds to serum IgE attached to their FCεRI receptors, they release cytokines, eicosanoids and their secretory granules (Amin, Citation2012). After activation, mast cells exert their biological effects by releasing preformed and de novo-synthesized mediators, such as histamine, leukotrienes and various cytokines/chemokines (Okayama et al., Citation2007). IgE-mediated mast cell degranulation is an important initial event in the development of asthma.
Mast cell degranulation was achieved with compound 48/80. This chemical causes mast cell degranulation and promotes histamine release by activation of G-proteins and influx of Ca2+ ions into the mast cell. Compound 48/80 activates phospholipidase D (PLD), leading to the generation of endogenous lysophosphatic acid receptor-activating phospholipids with subsequent mast cell degranulation (Palomaki & Laitinen, Citation2006). Sodium cromoglycate inhibits mediator release from bronchial mast cells; reverse the increased functional activation in leukocytes obtained from the blood of asthmatic patients; suppress the activating effects of chemotactic peptides on human neutrophils, eosinophils, and monocytes; inhibit parasympathetic and cough reflexes; and inhibit leukocyte trafficking in asthmatic airways.
Properties of ketotifen which may contribute to its anti-allergic activity and its ability to affect the underlying pathology of asthma include the inhibition of the development of airway hyper-reactivity associated with the activation of platelets by platelet activating factor (PAF), inhibition of PAF-induced eosinophil accumulation and inhibition of the release of mast cell mediators such as histamine, leukotrienes C4 and D4, and PAF (Mita & Shida, Citation1995). Ketotifen also has calcium ion antagonistic property (Craps & Ney, Citation1984).
The similarity of result between PFE and the reference mast cell stabilizers suggests that PFE may have phytochemical components that could act via some of the mechanisms outlined for the reference drugs.
Preliminary phytochemical screening of the ethanol leaf extract of P. fruticosa conducted by Koffuor et al. (Citation2014) indicated the presence of sterols, alkaloids, saponins, and cyanogenic glycosides, which may have conferred anti-inflammatory property to the extract. Studies have shown that plant sterols increase cytokine production by T helper cells type 1 (Th1); thus could be essential in conditions with Th2 increased response as characteristic features such as asthma (Katan et al., Citation2003). Saponins have been identified to have anti-inflammatory and mast cell stabilizing activity (Soetan et al., Citation2006) attributable to its ability to inhibit the synthesis of prostaglandins, thromboxanes, arachidonic acid, histamine, bradykinin and serotonin; all these being markers of asthma (Desai et al., Citation2009; Jeffers, Citation2006). Alkaloids have the property of dilating bronchial airways, thus enhancing breathing, therefore, is used in managing respiratory diseases.
Conclusion
The ethanol leaf extract of P. fruticosa has anti-asthmatic, antihistaminic, and mast cell stabilization effects, making it very useful in the traditional management of asthma.
Acknowledgements
Our gratitude also goes to Dr. Samuel Abokyi, Department of Optometry, University of Cape-Coast, Ghana for his technical support, and the entire laboratory staff, especially Mr. Thomas Ansah, of the Department of Pharmacology, Kwame Nkrumah University of Science and Technology, Ghana.
Declaration of interest
The authors report that they have no conflicts of interest.
References
- Abayaneh MR, Mahendra R. (2013). Antifungal Metabolites from Plants. Heidelberg, Berlin: Springer-Verlag.
- Alexopoulos EC, Bouros D, Dimadi M, et al. (2011). Comparative analysis of induced sputum and bronchoalveolar lavage fluid (BALF) profile in asbestos exposed workers. J Occup Med Toxicol 6:1–7.
- Amin K. (2012). The role of mast cells in allergic inflammation. Respir Med 106:9–14.
- Amoah AS, Forson AG, Boakye DA. (2012). A review of epidemiological studies of asthma in Ghana. Ghana Med J 46:23–8.
- Anonymus. (2011). Global Asthma Report (GAR) Paris, France: The International Union Against Tuberculosis and Lung Disease.
- Armitage AK, Boswood J, Large BJ. (1961). Thioxanthines with potent bronchodilator and coronary dilator properties. Br J Pharmacol Chemother 16:59–76.
- Bensita MB, Nilani P, Madhu CD. (1998). Studies on the antipyretic, anti-inflammatory, analgesic and molluscicidal properties of Polyscias fruticosa (L) Harms. Ancient Sci Life 17:313–19.
- Bertaccini G, Coruzzi G. (1995). An update on histamine H3 receptors and gastrointestinal functions. Dig Dis Sci 40:2052–63.
- Bousquet J, Jeffery PK, Busse WW, et al. (2000). Asthma. From bronchoconstriction to airways inflammation and remodelling. Am J Respir Crit Care Med 161:1720–45.
- Braman SS. (2006). The global burden of asthma. Chest 130:4S–12.
- Brightling CE, Bradding P, Symon FA, et al. (2002). Mast-cell infiltration of airway smooth muscle in asthma. N Engl J Med 346:1699–705.
- Brunton LL, Parker KL, Blumenthal DK, Buxton ILO. (2008). Goodman and Gilman's Manual of Pharmacology and Therapeutics. 12th ed. New York: The McGraw-Hill Company.
- Clark CE, Arnold E, Lasserson TJ, Wu T. (2010). Herbal interventions for chronic asthma in adults and children: A systematic review and meta-analysis. Prim Care Respir J 19:307–14.
- Craps LP, Ney UM. (1984). Ketotifen: Current views on its mechanism of action and their therapeutic implications. Respiration 45:411–21.
- Desai S, Desai D, Kaur H. (2009). Saponins and their biological activities. Pharmacol Times 41:13–16.
- Eisenberg DM, Davis RB, Ettner SL, et al. (1998). Trends in alternative medicine use in the United States, 1990–1997: Results of a follow-up national survey. JAMA 280:1569–75.
- Galli S, Tsai M, Piliponsky A. (2008). The development of allergic inflammation. Nature 24:445–54.
- Gleich GJ, Adolphson CR, Leiferman KM. (1993). The biology of the eosinophilic leukocyte. Annu Rev Med 44:85–101.
- Global Initiative for Asthma (GINA). (2013). Vancouver, Washington, DC: National Heart, Lung, and Blood Institute; World Health Organization. “Global Strategy for Asthma Management and Prevention”.
- Gohil PV, Mehta AA. (2011). Evaluation of mast cell stabilizing and anti-anaphylactic activity of polyherbal formulation. Adv Biol Res 5:304–8.
- Jeffers M. (2006). Tannins as Anti-Inflammatory Agents. Miami, OH: University Oxford.
- Kalpana GP, Jaysukh RD, Tanmay AS, et al. (2011). Evaluation of the effect of Onosma bracteatum, Wall (Boraginaceae) using experimental allergic and inflammatory models. Global J Pharmacol 5:40–9.
- Karol MH. (1994). Animal models of occupational asthma. Eur Respir J:555–68.
- Katan MB, Grundy SM, Jones P, et al. (2003). Efficacy and safety of plant stanols and sterols in the management of blood cholesterol levels. Mayo Clin Proc 78:965–78.
- Koffuor GA, Boye A, Amoateng P, et al. (2012). Investigating the site of action of an aqueous extract of Heliotropium indicum Linn (Boraginaceae) on smooth muscles. Res J Pharmacol 6:12–19.
- Koffuor GA, Boye A, Ofori-Amoah J, et al. (2014). Anti-inflammatory and safety assessment of Polyscias fruticosa (L.) Harms (Araliaceae) leaf extract in ovalbumin-induced asthma. J Phytopharmacol 3:337–42.
- Lagente V, Manoury B, Nénan S, et al. (2005). Role of matrix metalloproteinases in the development of airway inflammation and remodelling. Braz J Med Biol Res 38:1521–30.
- Leurs R, Brozius MM, Smit MJ, et al. (1991). Effects of histamine H1-, H2-, and H3-receptor selective drugs on the mechanical activity of guinea-pig small and large intestine. Br J Pharmacol 102:179–85.
- Mazaik W. (2005). The asthma epidemic and our artificial habitats. BMC Pulm Med 5:1–7.
- Miller AL. (2001). The etiologies, pathophysiology, and alternative/complementary treatment of asthma. Altern Med Rev 6:20–47.
- Mita H, Shida T. (1995). Comparison of anti-allergic activities of the histamine H1 receptor antagonists epinastine, ketotifen and oxatomide in human leukocytes. Arzneimittelforschung 45:35–40.
- Moucaut A, Nienhaus A, Courtois B, et al. (2013). The effect of introducing IGRA to screen French healthcare workers for tuberculosis and potential conclusions for the work organisation. J Occup Med Toxicol 8:12. doi: 10.1186/1745-6673-8-12.
- Okayama Y, Ra C, Saito H. (2007). Role of mast cells in airway remodelling. Curr Opin Immunol 19:687–93.
- Palomaki VA, Laitinen JT. (2006). The basic secretagogue compound 48/80 activates G-proteins indirectly via stimulation of phospholipase d-lysophatidic acid receptor axis and 5-HT1A receptors in rat brain sections. Br J Pharmacol 147:596–605.
- Parmar S, Gangwal A, Sheth N. (2010). Mast cell membrane stabilization and anti-histaminic actions-possible mechanism of action of anti-inflammatory action of Murraya koenigii. J Curr Pharm Res 2:21–5.
- Rang HP, Dale MM. (2007). Rang and Dale’s Pharmacology, 6 ed. Edinburgh: Churchill Livingstone.
- Ricciardolo FL, Nijkamp F, De Rose V, Folkerts G. (2008). The guinea pig as an animal model for asthma. Curr Drug Targets 9:452–65.
- Sarpate RV, Deore TK, Tupkari SV. (2009). Bronchodilatory effect of Sphaeranthus indicus Linn against allergen induced bronchospasm in guinea pigs. Phcog Mag 5:74–7.
- Smith N, Broadley KJ. (2007). Optimisation of the sensitisation conditions for an ovalbumin challenge model of asthma. Int Immunopharmacol 7:183–90.
- Soetan K, Oyekunle M, Aiyelaagbe O, Fafunso M. (2006). Evaluation of the anti-microbial activity of saponins of extract of Sorghum bicolor L. Moench. Afr J Biotechnol 5:2405–7.
- Toward TJ, Broadley KJ. (2004). Early and late bronchoconstrictions, airway hyper-reactivity, leucocyte influx and lung histamine and nitric oxide after inhaled antigen: Effects of dexamethasone and rolipram. Clin Exp Allergy 34:91–102.
- Trzeciakowski JP. (1987). Inhibition of guinea pig ileum contractions mediated by a class of histamine receptor resembling the H3 subtype. J Pharmacol Exp Ther 243:874–80.
- Varadharajan R, Rajalingam D. (2011). Diuretic activity of Polyscias fruticosa (L.) Harms. Int J Innovative Drug Discov 1:15–18.
- Vo DH, Yamamura S, Ohtani K, et al. (1998). Oleanane saponins from Polyscias fruticosa. Phytochemistry 47:451–7.
- Weinberger M, Abu-Hasan M. (2007). Pseudo-asthma: When cough, wheezing, and dyspnea are not asthma. Pediatrics 120:855–64.
- WHO. Public Health and the Environment. Geneva: World Health Organization; 2009. Ghana: Country Profile of Environmental Burden of Disease.
- Wu Z, Zhou D, Chen G, Lee L. (2000). Airway hyperresponsiveness to cigarette smoke in ovalbumin-sensitized guinea pigs. Am J Respir Crit Care Med 161:73–80.
- Zavecz JH, Yellin TO. (1982). Histamine receptors in the myenteric plexus longitudinal muscle of the guinea-pig ileum: H1-and H2-receptor-mediated potentiation of the contractile response to electrical stimulation. J Pharmacol Exp Ther 233:177–82.
- Zhang Y, Lamm WJ, Albert RK, et al. (1997). Influence of the route of allergen administration and genetic background on the murine allergic pulmonary response. Am J Respir Crit Care Med 155:661–9.