Abstract
Contexts: Exposure to environmental pollutants such as carbon tetrachloride (CCl4) causes liver injuries. There are claims that extracts from Syzygium aromaticum (Linn.) Merrill & L.M.Perry, (Myrtaceae) protects from such injuries.
Objective: This study investigates the protective effects of cold-pressed S. aromaticum oil (CO) against CCl4-induced liver toxicity in rats.
Materials and methods: CO was orally administered to rats in two doses (100 and 200 mg/kg) along with CCl4 (1 mL/kg in olive oil) for 8 weeks. Indices of liver and kidney functions, lipid profile, and peroxidation were evaluated in rats’ serum and tissues. Fatty acids and bioactive lipids of CO were analyzed.
Results: High levels of monounsaturated fatty acids (39.7%) and polyunsaturated fatty acids (42.1%) were detected in CO. The oil contained high amounts of tocols and phenolics. The LD50 value at 24 h was approximately 5950 mg/kg. Treatment with 200 mg/kg CO resulted in a decrease of creatinine, urea, and uric acid levels to 0.86, 32.6, and 2.99 mg/dL, respectively. Levels of TL, TC, TAG, LDL-C, and VLDL-C were decreased to 167, 195.3, 584.5, 74.6, and 39.0 mg/L, respectively, after 8 weeks of treatment. Hepatic malondialdehyde levels were reduced and glutathione levels were elevated in CO-treated rats. CO reduced the activities of AST, ALT, and ALP as well as kidney function markers, protein, and lipid profiles, respectively. Histopathological examination of liver indicated that CO treatment reduced fatty degenerations, cytoplasmic vacuolization, and necrosis.
Conclusion: CO possessed a protective effect against CCl4-induced hepatotoxicity in rats, mediated possibly by the antioxidant properties of the oil.
Introduction
The liver plays an important role in metabolism of endo- and exo-genous substances, wherein the hepatic injury is associated with distortion of these functions. Liver, therefore, is susceptible to diseases including hepatitis, cirrhosis, alcohol-related disorders, and liver cancer (Al-Sayed et al., Citation2014). A major cause of these disorders is due to exposure to different environmental pollutants and xenobiotics [i.e., carbon tetrachloride (CCl4), thioacetamide, paracetamol, and alcohol]. These toxicants mainly damage the liver by producing reactive oxygen species (ROS) (Liu et al., Citation2011). ROS have been known to produce tissue injury through covalent binding and lipid oxidation and have been shown to augment fibrosis as seen from increased collagen synthesis (Adesanoye & Farombi, Citation2010; Blomhoff, Citation2005). Scavenging of free radicals by defense systems could reduce the fibrosis process in the tissues (Qian et al., Citation2008; Shim et al., Citation2010). Cooperative defense systems that protect body from free radical damage include antioxidants and enzymes [superoxide dismutase (SOD), catalase (CAT), and glutathione peroxidase (GPH)]. The role of protective enzymes is well known and has been studied deeply with in vivo models (Ghassam et al., Citation2014; Jaydeokar et al., Citation2014).
CCl4, an industrial solvent, is extensively used xenobiotic to induce chemical liver injury. CCl4 is metabolized by hepatic microsomal cytochrome P450 to free radical such as trichloromethyl radical (⋅CCl3) and proxy trichloromethyl radical (⋅OOCCl3) (Akram et al., Citation2011; Lee et al., Citation2004). Trichloromethyl, hepatotoxic metabolite of CCl4, can react with sulfhydryl groups (e.g., glutathione and protein thiols) and affect enzymes such as CAT and SOD. Over production of trichloromethyl-free radicals initiate a membrane lipid oxidation, eventually leading to various liver pathological processes, such as steatosis, fibrosis, or cirrhosis.
Nowadays, many people are suffering from hepatic damage induced by alcohol, chemicals and infections. Therefore, liver diseases continue to be a serious health problem worldwide. Interest in the role of naturally originated agents for the treatment of liver diseases was considered. Some natural compounds are demonstrated to have a protective role against liver diseases (Pramyothin et al., Citation2007; Shaker et al., Citation2010; Wan et al., Citation2009). Studies revealed that natural bioactive compounds, containing antioxidants, protect the liver against lipid oxidation, and impairment in antioxidant status induced by CCl4 (Noyan et al., Citation2006; Sanmugapriya & Venkataraman, Citation2006; Shaker et al., Citation2010, Citation2011). ROS production is linked with oxidative stress, which is defined as an imbalance in the generation of oxidants and the antioxidant defense. ROS have an important role in liver disease, while antioxidants might prevent hepatic damage through scavenger activity and increase the activity of intracellular antioxidant enzymes including SOD, GSH and CAT (Upur et al., Citation2009). Polyphenolic compounds have marked antioxidant effect and can prevent the liver damage caused by free radicals (Al-Sayed et al., Citation2014). Studies reported that natural antioxidants and plant extracts are efficacious in preventing oxidative stress-related liver pathologies due to particular interactions and synergism (Vitaglione et al., Citation2004; Yeh et al., Citation2013).
There is much evidence indicating that natural bioactive substances, extracts, and oils from vegetable, fruits, oilseeds, and medicinal plants exhibit strong antioxidant potential that could act against hepatic toxicity. One such candidate is clove [Syzygium aromaticum L. (Myrtaceae)] oil, which was chosen in the present study. S. aromaticum essential oil has antimicrobial and antioxidant properties (Gulcin et al., Citation2012; Huang et al., 2002). Cloves treatment reduced blood sugar and lipid oxidation in streptozotocin-induced diabetic rats by restoring the levels of antioxidant enzymes (Shukri et al., Citation2010). Clove oil was listed as a ‘‘Generally Regarded As Safe’’ substance by Food and Drug Administration (FDA) when administered at levels under 1500 ppm in food. In addition, the World Health Organization (WHO) Expert Committee on Food Additives has established the acceptable daily human intake of clove oil at 2.5 mg/kg body weight (Kildeaa et al., Citation2004). Cloves contain a variety of bioactive compounds such as sesquiterpenes, tannins, and triterpenoids. The main aroma constituent of clove buds, eugenol (4-allyl-2-methoxyphenol), was reported to have antifungal activity (Lee & Shibamoto, Citation2002; Miyazawa & Hisama, Citation2003). Eugenol was classified by United States Food and Drug Administration (FDA) to be a substance that is generally regarded as safe (Gulcin et al., Citation2012). Eugenol was reported to inhibit malonaldehyde formation from cod liver oil and the formation of hexanal (Gulcin et al., Citation2004). Over the last years, interest in cold pressed oils has been increased because cold pressing involves no heat or chemical treatments. Cold pressing also involves no refining process and may contain a high level of lipophilic bioactive phytochemicals including antioxidants (Ramadan et al., Citation2012). Recently, it was reported that cold-pressed clove oil (CO) is a rich source of essential fatty acids and bioactive compounds including tocols, phospholipids and phenolic compounds. CO was also characterized by its high antiradical potential against free radicals including DPPH and galvinoxyl radicals (Ramadan et al., Citation2013).
It is hard to find data in the literature on the protective impact of CO on CCl4-induced oxidative damage in rats. Therefore, in the present study, we investigated the protective impacts of CO against CCl4-induced oxidative stress and hepatotoxicity in rats. The extent of CCl4-induced liver injury was monitored by measuring different biochemical and histopathological parameters.
Materials and methods
Chemicals
Cold-pressed CO was obtained from a local market (Zagazig, Egypt). CO has specifications and codex standard approved by Egyptian organization for standardization and quality (approval number 66/92). CCl4 and standards used for tocols were purchased from Merck (Darmstadt, Germany). Assay kits and other chemicals were of the analytical grade and purchased from Sigma Chemical Co. (St. Louis, MO).
Gas chromatography (GC) of fatty acids methyl esters (FAME) in CO
Fatty acids in CO were transesterified into fatty acid methyl esters (FAME) by N-trimethylsulfoniumhydroxide (Macherey-Nagel, Germany) according to Arens et al. (Citation1994). FAME were detected on a Shimadzu GC-14A equipped with flame ionization detector (FID) and C-R4AX chromatopac integrator (Shimadzu Inc., Kyoto, Japan). The flow rate of the carrier gas helium was 0.6 mL/min and the split value with a ratio of 1:40. A sample of 1 μL was injected on a 30 m × 0.25 mm × 0.2 μm film thickness Supelco SPTM-2380 (Bellefonte, PA) capillary column. The injector and the FID temperature were set at 250 °C. The initial column temperature was 100 °C programmed by 5 °C/min until 175 °C and kept 10 min at 175 °C, then 8 °C/min until 220 °C, and kept 10 min at 220 °C. A comparison between the retention times of the samples with those of an authentic standard mixture (Sigma, St. Louis, MO; 99% purity specific for GLC), run on the same column under the same conditions, was made to facilitate identification.
HPLC of tocols in CO
A solution of 250 mg of CO in 25 mL n-heptane was directly used for the HPLC (Ramadan, Citation2013). The HPLC analysis was conducted using a Merck Hitachi low-pressure gradient system, fitted with an L-6000 pump, a Merck-Hitachi F-1000 Fluorescence Spectrophotometer (the detector wavelength was set at 295 nm for excitation, and at 330 nm for emission) and a D-2500 integration system; 20 μL of the samples were injected by a Merck 655-A40 Autosampler onto a Diol phase HPLC column 25 cm × 94.6 mm ID (Merck, Darmstadt, Germany) using a flow rate of 1.3 mL/min. The mobile phase used was n-heptane/tert-butyl methyl ether (99:1, v/v).
Extraction and quantification of phenolic compounds in CO
CO (1 g) was dissolved in n-hexane (5 mL) and mixed with 10 mL methanol–water (80:20, v/v) in a glass tube for 2 min in a vortex (Ramadan et al., Citation2010). After centrifugation at 3000 rpm for 10 min, the hydroalcoholic extracts were separated from the lipid phase by using a Pasteur pipette then combined and concentrated in vacuo at 30 °C until a syrup consistency was reached. The lipidic residue was redissolved in 10 mL methanol-water (80:20, v/v) and the extraction was repeated twice. Hydroalcoholic extracts were redissolved in acetonitrile (15 mL) and the mixture was washed three times with n-hexane (15 mL each). Purified phenols in acetonitrile were concentrated in vacuo at 30 °C then dissolved in methanol for further analysis. Aliquots of phenolic extracts were evaporated to dryness under nitrogen. The residue was re-dissolved in 0.2 mL water and diluted (1:30) Folin–Ciocalteu’s phenol reagent (1 mL) was added. After 3 min, 7.5% sodium carbonate (0.8 mL) was added. After 30 min, the absorbance was measured at 765 nm using a UV-260 visible recording spectrophotometer (Shimadzu, Kyoto, Japan). Gallic acid was used for the calibration and the results of triplicate analyses are expressed as parts per million of gallic acid.
Experimental animals
Experimental procedures involving the animals and their care were conducted in conformity with the institutional guidelines and the Guidelines for Care and Use of Laboratory Animals in Biomedical Research as adopted and promulgated by the World Health Organization. Healthy adult male albino rats (Wister Strain) of approximately same age, weighing approximately 120–140 g, were purchased from Organization of Biological Products and Vaccines (Helwan Farm, Helwan, Egypt). Animals were housed under ambient temperature (25 ± 2 °C) with 50 ± 5% relative humidity and a 12 h light-dark cycle. Rats received standard pellet diet comprised of 20% crude protein, 5% fat, 4.5% crude fiber, 8% ash, 2% calcium, 0.6% phosphorus, 3.4% glucose, 2% vitamins, and water ad libitum.
Experimental design
Twenty-four rats were used to study the protective role of CO on the CCl4-induced hepatotoxicity in rats. CCl4 was mixed with olive oil in the ratio of 1:1 (w/w) and used for hepatoprotective potential against CCl4-induced liver damage. Animals were divided randomly into four equal groups (six rats each) and treated as follows:
Group 1 (negative control group): Rats were fed on standard synthetic diet for 8 weeks.
Group 2 (positive control group): Rats were fed on standard diet and received with equal mixture of CCl4 and olive oil orally (three time a week) by gastric gavages at a dose of 1 mL/kg (b.w.) for 8 weeks.
Group 3: Rats were fed on standard diet and received CO orally (three times a week) by gastric gavages at a dose of 100 mg/kg, simultaneously with equal mixture of CCl4 and olive oil by gastric gavages at dose of 1 mL/kg during the last 4 weeks.
Group 4: Rats were fed on standard diet and received CO orally (three times a week) by gastric gavages at a dose of 200 mg/kg, simultaneously with equal mixture of CCl4 and olive oil by gastric gavages at dose of 1 mL/kg during the last 4 weeks.
Blood sampling and biochemical evaluation
Blood samples were collected at the end of experiment and obtained from the retro-orbital plexus veins from the individual rat by means of fine capillary heparinized tubes. Blood samples was collected and allowed to clot, serum was separated by centrifugation at 3000 rpm for 15 min. Serum was used to investigate the biochemical parameters including liver and kidney function tests and serum lipid profile.
Activities of liver enzymes including alanine transaminase (ALT) and aspartate transaminase (AST), alkaline phosphatase (ALP) as well as the serum total bilirubin (TB), direct bilirubin (DB), total protein and serum albumin were determined according to the methods of Reitman and Frankel (Citation1957), Tietz et al. (Citation1983), Doumas et al. (Citation1973), and Doumas et al. (Citation1971), respectively. The globulin was calculated by subtracting the albumin from serum total protein. Kidney function parameters including urea, uric acid, and creatinine were measured by using the method of Amer et al. (Citation2007). Lipid profile including total lipids (TL), triglycerides (TAG), total cholesterol (TC), high-density lipoprotein cholesterol (HDL-C), low-density lipoprotein cholesterol (LDL-C), and very low-density lipoprotein cholesterol (VLDL-C) was determined according to the methods of Ramadan et al. (Citation2008).
Antioxidant markers
Liver samples were washed immediately with ice-cold saline to remove excess blood. Liver tissue was homogenized in cold 0.1M potassium phosphate saline (pH = 7.4) at a concentration of 10% (w/v). The homogenate was then centrifuged at 5000 rpm for 10 min at 4 °C to obtain the supernatant, which was used to investigate antioxidant markers. Lipid peroxides (malondialdehyde, MDA) were measured according to Mihara and Uchiyama (Citation1978). Reduced glutathione (GSH) content in liver was measured spectrophotometrically using Ellman’s reagent (DTNB) following the method described by Moron et al. (Citation1979). The absorbance was read at 412 nm and the standard graph was drawn using different concentrations of a standard GSH solution and GSH contents were calculated as nM per mg of tissue protein.
Histopathological examination
Small tissue specimens were collected from fresh liver tissue of the rats and rapidly fixed in 10% neutral buffered formalin. After proper fixation, thin paraffin sections were routinely prepared and stained with hematoxylin and eosin stain (H&E) for the histopathological lesions in hepatic and renal tissues. The liver sections were graded numerically to assess the degree of histopathological features of acute hepatic injury.
Statistical analysis
All data were analyzed by one-way ANOVA. Duncan’s new multiple-range test was used to resolve the difference among treatment means. All statistical analyses were performed using the statistical software SPSS 11.0 (SPSS Ltd., Surrey, UK). A p < 0.05 was considered statistically significant. Ratio values were not arcsin transformed before statistical analysis.
Results
Fatty acids and bioactive lipids of CO
Nine fatty acids were found in CO. The percentages of C10:0, C12:0, C14:0, C16:0, C16:1, C18:0, C18:1n-9, C18:2n-6, and C18:3 were 2.76, 0.07, 0.06, 8.57, 0.36, 6.59, 39.4, 40.2, and 1.99%, respectively. Linoleic and oleic acids were the main fatty acids comprising together almost 80% of total fatty acids. CO contained significant levels of monounsaturated fatty acids (MUFA, 39.7 g/100 g total fatty acids) and had a polyunsaturated fatty acids (PUFA) content of 42.1 g/100 g of total fatty acids. Palmitic and stearic were the major saturated fatty acids (SFA), comprising together about 15% of total identified FAME. CO was characterized by high levels of unsaponifiables (25.3 g/kg). Levels of α-, β-, γ- and δ-tocopherols were 14 890, 56, 4184, and 186 mg/kg CO, respectively. In addition, amounts of α-, β-, γ-, and δ-tocotrienols were 1110, 55, 85, and 9498 mg/kg CO, respectively. α-Tocopherol constituted about 49.5% of the total tocols followed by δ-tocotrienol (31.6%) and γ-tocopherol (13.9%). Other tocols were measured in lower levels. CO was also characterized by high level of phenolics (4.6 mg GAE/g).
Effect of CO on liver enzymes, kidney function indicators, protein profile, lipids profile, and antioxidant markers in CCl4-induced liver injury rats
The LD50 value of CO was 5950 mg/kg based on a 24 h oral toxicity study. In a long-term toxicity study of CO (100, 200, and 400 mg/kg, p.o.), only 400 mg/kg induced a decrease in body weight. The effect of two doses of CO on serum liver enzymes in CCl4-treated rats was studied and the results are given in . After injection of CCl4, activities of AST, ALT, and ALP enzymes in the CCl4-treated group (groups 2) were significantly increased (p <0.05), as compared with the negative control (group 1). Administration of CO attenuated the increased levels of AST, ALT, and ALP enzymes induced by CCl4 and caused a subsequent recovery toward normalization comparable with the negative control (group 1). Treatment of animals with both doses of CO significantly reduced the activities of serum AST, ALT, and ALP enzymes as compared with the positive group of CCl4-treated alone. In addition, levels of TB and DB were also reduced significantly upon treatment with CO (groups 3 and 4).
Table 1. Changes in liver enzymes in CCl4-induced oxidative damage in rat liver.
presents the impact of CO on kidney function indicators and protein profile of CCl4-treated rats. Levels of creatinine, urea and uric acid in negative control (group 1) after 8 weeks of experiments were 0.716, 25.3, and 3.63 mg/dL, respectively. On one hand, it could be seen that treatment with CCl4 (group 2) resulted in increase in the levels of creatinine, urea and uric acid levels (1.85, 56.3, and 7.93 mg/dL, respectively). On the other hand, treatment with CO resulted in decrease of creatinine, urea, and uric acid levels. Concerning protein profile (total protein, albumin, globulin, and A/G ratio), results revealed that treatment with CCl4 (group 2) reduced the protein parameters in general (), while treatment with both doses of CO increased protein profile to levels resemble negative control (group 1).
Table 2. Impact of CO on kidney function indicators and protein profile in CCl4-induced injury in rat.
Impact of CO on lipid profile of CCl4-induced liver injury rats is presented in
Figure 1. Changes in lipid profiles (mg/L) in CCl4-induced injury in rat. Error bars show the variations of three determinations in terms of standard deviation.
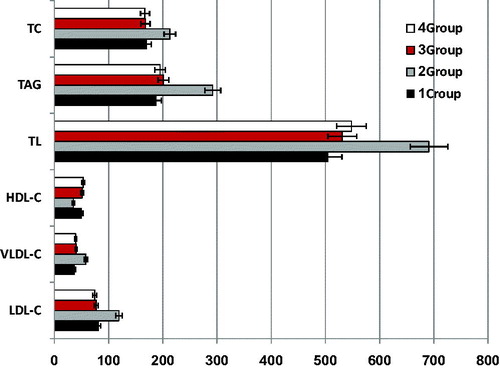
As shown in , the MDA (nmol/mg) level was markedly higher in CCl4-treated rats (group 2) after 8 weeks. Expected increases lipid oxidative indices in the CCl4-treated model confirmed that oxidative damage has been induced. Negative control (group 1) and groups (3 and 4) that treated with CO were characterized by lower levels of MDA. On one hand, it could be concluded that CO inhibited the elevation of MDA levels upon CCl4 administration. On the other hand, GSH level was markedly lower in CCl4-treated rats (group 2). However, the GSH level was significantly increased by CO treatment (groups 3 and 4) when compared with the positive control group. Administration of CO (groups 3 and 4) did significantly increase (p >0.05) the activity of GSH as compared to the CCl4-treated group.
Table 3. Changes in antioxidant markers in CCl4-induced injury in rats.
Histopathology of the liver
The results of hepatic histopathological examination are shown in
Figure 2. Photomicrograph of liver from (A) control rats showing normal histobasoligical structure (H&E × 300), (B) rats received CCl4 (100 mg/kg) showing severe fatty change in the hepatocytes (H&E × 300), (C) rats received CCl4 (100 mg/kg) and CO (100 mg/kg) showing degenerative changes in hepatocytes in the form of vaculation with focal mononuclear aggregation (H&E × 800), (D) rats received CCl4 100 mg/kg and CO (200 mg/kg) showing congestion of the central vein and hepatic sinusoids (H&E × 300).
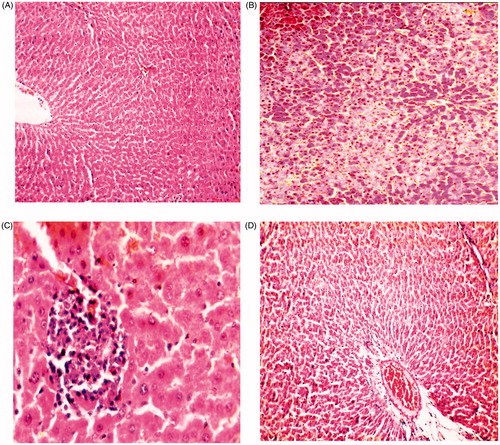
Discussion
The liver, as a key organ of metabolism and excretion, is constantly endowed with the task of detoxification. Hepatotoxicants, including viruses, fungal products, bacterial metabolites, minerals, environmental pollutants and chemotherapeutic agents, can induce various disorders of the organ (Ha et al., Citation2005). Among the various health problems, liver diseases, including hepatocellular carcinoma, fibrosis, cirrhosis and hepatitis appear to be the most serious. Hepatotoxins, such as ethanol, acetaminophen and CCl4, induced liver injury, which is characterized by varying degrees of hepatocyte degeneration and cell death (Yeh et al., Citation2013). Vitaglione et al. (Citation2004) suggested that ROS including superoxide and hydroxyl radicals have been proved to be associated with the intoxication by CCl4. Evidence suggested that CCl4 has been commonly used as a hepatotoxin in experimental hepatopathy (Hsu et al., Citation2008). Covalent binding of the metabolites of CCl4, trichloromethyl-free radicals, to cell proteins is considered to be the initial step in a chain of events that eventually lead to membrane lipid oxidation and finally to cell death.
In recent years, there has been a substantial increase in the use of so-called complementary dietary phenolics and alternative therapies to treat patients with liver disease. Epidemiological studies suggested an inverse relation between the consumption of phenol-rich foods and the risk of degenerative diseases, particularly cancers and cardiovascular diseases (Arts & Hollman, Citation2005). In this regard, there has been a great deal of interest in the screening and characterization of novel potentially therapeutic of phenolics, phenol-rich foods and medicinal plants (Murcia et al., Citation2001; Seeram et al., Citation2005). The antioxidant properties of phenolic compounds and their ability to modulate the activity of enzymes have been demonstrated in vitro studies and are believed to be a primary mechanism for their biological impacts. However, the question remains of whether these properties demonstrated in in vitro studies are relevant to protect against oxidative damage in vivo, where polyphenolic compounds are found at a very low concentrations depending on bioavailability and metabolism.
An experiment has been designed on rat model to evaluate the protective effects of the CO against the toxicity caused by CCl4 in rats. CCl4 increased lipid oxidation, and affects different blood biochemical parameters including liver enzymes, kidney function indicators, protein profile, lipid profile, and antioxidant markers. CO treatment prevented these effects, indicating that CO was able to attenuate the lipid oxidation induced by CCl4. For example, the protective role of CO was accompanied with a partial prevention of GSH depletion in the liver tissue. It is considered that hepatic GSH represents an enzyme reserve of the liver, which is responsible for reducing the hepatotoxicity induced by the active metabolites of CCl4. As GSH is also a crucial determinant of tissue susceptibility to oxidative damage, the partial protection of CO on GSH reserves provide an additional action not only to remove the active metabolites of CCl4 but also to scavenge free radicals, which are involved in lipid oxidation. The effect of CO was further confirmed by histopathological examinations. Hepatocyte necrosis, fatty change, hyaline degeneration, ballooning degeneration, and infiltration of Kupffer cells and lymphocytes were prominent in the histological findings. CO offers a hepatoprotection impact in central lobular necrosis, hepatic lipidosis, and cholangiocyte hyperplasia in rats.
It has been hypothesized that one of the principal causes of CCl4-induced liver injury is the formation of lipid peroxides by free radical derivatives of CCl4. Thus, the antioxidant potential or the inhibition of the generation of free radicals is important in protection against CCl4-induced hepatopathy. The body has an effective defense mechanism to prevent and neutralize the free radical induced damage by a set of endogenous antioxidant enzymes such as SOD and CAT. These enzymes constitute a mutually supportive defense team against ROS. Lipid peroxidation, a ROS-mediated mechanism, has been implicated in the pathogenesis of various liver injuries and subsequent liver fibrogenesis in experimental animals. The significant non-dose-dependent decrease in the hepatic lipid hydroperoxide confirmed that treatment with CO could effectively protect against the hepatic lipid oxidation induced by CCl4. Hence, it is possible that the mechanism of hepatoprotection of CO may be due to its antioxidant activity.
CO contains high-level health-promoting fatty acids such as MUFA and PUFA. From the health point of view, MUFA have been shown to lower “bad” LDL-C and retain “good” HDL-C. This is the major benefit of olive oil over the highly polyunsaturated oils, wherein PUFA reduce both the “bad” as well as the “good” serum cholesterol levels in blood (Ramadan et al., Citation2010). On one hand, clove oil also contains high levels unsaponifiables, tocols, and phenolic compounds. Oils rich in phenolics may play an important role in reducing the risk of many diseases. On the other hand, phenolics bioavailability was found to be higher when phenolic compounds are soluble in a lipid matrix than in an aqueous media (Ramadan, Citation2013). The antioxidant potential of phenolics is mainly due to their redox properties and is the result of various mechanisms: antiradical activity, transition-metal-chelating activity, and/or singlet-oxygen-quenching capacity (Bettaieb et al., Citation2010). Phenolics exhibit favorable hepatoprotective effects and could prevent chemical induced dyslipidemia, inflammatory response, and mitochondrial oxidative damage of rat hepatocytes. Therefore, bioactive compounds with remarkably high antioxidant activity, superior free radical-scavenging ability, and inhibition of lipid oxidation are contributed to the hepatoprotective effect in rats against liver damage induced by CCl4 (Hu et al., Citation2012; Liu et al., Citation2012; Tang et al., Citation2012; Yeh et al., Citation2013; Yousef et al., Citation2010). Because the phytochemicals, analysis of CO showed the presence of tocols and phenolic compounds, and hepatoprotective activities of such constituents are well known, there has been speculation that these constituents might be responsible for the observed hepatoprotective roles. From the overall results, it was concluded that CO exhibits antiradical and antioxidant activities, which could have a beneficial effect against oxidative liver damage induced by CCl4.
Conclusion
Cold-pressed clove oil is a rich source of health-promoting antioxidants and possessed a hepatoprotective effect against CCl4-induced toxicity in rats.
Declaration of interest
The authors report that they have no conflicts of interest.
References
- Adesanoye OA, Farombi EO. (2010). Hepatoprotective effects of Vernonia amygdalina (Astereaceae) in rats treated with carbon tetrachloride. Exp Toxicol Pathol 62:197–206.
- Akram E, Maryam E, Al-Ebrahimc M, et al. (2011). Protective effects of sodium molybdate on carbon tetrachloride-induced hepatotoxicity in rats. J Trace Elem Med Biol 25:67–71.
- Al-Sayed E, El-Lakkany NM, Seif el-Din SH, et al. (2014) Hepatoprotective and antioxidant activity of Melaleuca styphelioides on carbon tetrachloride-induced hepatotoxicity in mice. Pharm Biol 52:1581–90.
- Amer MMA, Ramadan MF, Abd El-Gleel W. (2007). Impact of Pulicaria incisa, Diplotaxis harra and Avicennia marina as hypocholesterolemic agent. Deutsche Leben Rund 103:320–7.
- Arens M, Schulte E, Weber K. (1994). Fettsäuremethylester, Umesterung mit Trimethylsulfoniumhydroxid (Schnellverfahren). Fat Sci Technol 96:67–8.
- Arts IC, Hollman PC. (2005). Polyphenols and disease risk in epidemiologic studies. Am J Clin Nutr 81:317S–25S.
- Bettaieb I, Bourgou S, Wannes WA, et al. (2010). Essential oils, phenolics, and antioxidant activities of different parts of cumin (Cuminum cyminum L.). J Agric Food Chem 58:10410–18.
- Blomhoff R. (2005). Dietary antioxidants and cardiovascular disease. Curr Opin Lipidol 16:47–54.
- Doumas BT, Perry BW, Sasse EA, Straumfjord JV. (1973). Standardization in bilirubin assays: Evaluation of selected methods and stability of bilirubin solutions. Clin Chem 19:984–93.
- Doumas BT, Watson WA, Biggs HG. (1971). Albumin standards and the measurement of serum albumin with bromcresol green. Clin Chem Acta 31:87–96.
- Ghassam BJ, Ghaffari H, Prakash HS, Kini KR. (2014). Antioxidant and hepatoprotective effects of Solanum xanthocarpum leaf extracts against CCl4-induced liver injury in rats. Pharm Biol 52:1060–8.
- Gulcin I, Elmastas M, Aboul-Enein HY. (2012). Antioxidant activity of clove oil – A powerful antioxidant source. Arabian J Chem 5:489–99.
- Gulcin I, Sat IG, Beydemir S, et al. (2004). Comparison of antioxidant activity of clove (Eugenia caryophylata Thunb) buds and lavender (Lavandula stoechas L.). Food Chem 87:393–400.
- Ha KT, Yoon SJ, Choi DY. (2005). Protective effect of Lycium chinense fruit on carbon tetrachloride-induced hepatotoxicity. J Ethnopharmacol 96:529–35.
- Hsu YW, Tsai CF, Chang WH, et al. (2008). Protective effects of Dunaliella salina-a carotenoids-rich alga, against carbon tetrachloride-induced hepatotoxicity in mice. Food Chem Toxicol 46:3311–17.
- Hu QH, Zhang X, Pan Y, et al. (2012). Allopurinol, quercetin and rutin ameliorate renal NLRP3 inflammasome activation and lipid accumulation in fructose-fed rats. Biochem Pharmacol 84:113–25.
- Huang GJ, Deng JS, Huang SS, et al. (2012). Protective effect of antrosterol from Antrodia camphorate submerged whole broth against carbon tetrachloride-induced acute liver injury in mice. Food Chem 132:709–16.
- Jaydeokar AV, Bandawane DD, Bibave KH, Patil TV. (2014). Hepatoprotective potential of Cassia auriculata roots on ethanol and antitubercular drug-induced hepatotoxicity in experimental models. Pharm Biol 52:344–55.
- Kildeaa MA, Allanb GL, Kearney RE. (2004). Accumulation and clearance of the anaesthetics clove oil and AQUI-S from the edible tissue of silver perch (Bidyanus bidyanus). Aquaculture 232:265–77.
- Lee KG, Shibamoto T. (2002). Antioxidant property of aroma extract isolated from clove buds [Syzygium aromaticum (L.) Merr. et Perry]. Food Chem 74:443–8.
- Lee KJ, Woo ER, Choi CY, et al. (2004). Protective effect of acteoside on carbon tetrachloride-induced hepatotoxicity. Life Sci 74:1051–64.
- Liu Q, Baohua K, Li G, et al. (2011). Hepatoprotective and antioxidant effects of porcine plasma protein hydrolysates on carbon tetrachloride-induced liver damage in rats. Food Chem Toxicol 49:1316–21.
- Liu S, Hou W, Yao P, et al. (2012). Heme oxygenase-1 mediates the protective role of quercetin against ethanol-induced rat hepatocytes oxidative damage. Toxicol in Vitro 26:74–80.
- Mihara M, Uchiyama M. (1978). Determination of malonaldehyde precursor in tissues by thiobarbituric acid test. Anal Biochem 86:271–8.
- Miyazawa M, Hisama M. (2003). Antimutagenic activity of phenylpropanoids from clove (Syzygium aromaticum). J Agric Food Chem 51:6413–22.
- Moron, MS, Depierre JW, Mannervik B. (1979). Levels of GSH, GR and GST activities in rat lung and liver. Biochim Biophys Acta 582:67–78.
- Murcia MA, Jimenez AM, Martinez-Tome M. (2001). Evaluation of the antioxidant properties of Mediterranean and tropical fruits compared with common food additives. J Food Prot 64:2037–46.
- Noyan T, Kömüroglu U, Bayram I, Sekeroglu MR. (2006). Comparison of the effects of melatonin and pentoxifylline on carbon tetrachloride-induced liver toxicity in mice. Cell Biol Toxicol 22:381–91.
- Pramyothin P, Ngamtin C, Poungshompoo S, Chaichantipyuth C. (2007). Hepatoprotective activity of Phyllanthus amarus Schum. Et. Thonn. extract in ethanol treated rats: In vitro and in vivo studies. J Ethnopharmacol 114:169–73.
- Qian ZJ, Jung WK, Kim SK. (2008). Free radical scavenging activity of a novel antioxidative peptide purified from hydrolysate of bullfrog skin, Rana catesbeiana Shaw. Bioresour Technol 99:1690–8.
- Ramadan MF. (2013). Healthy blends of high linoleic sunflower oil with selected cold pressed oils: Functionality, stability and antioxidative characteristics. Ind Crops Prod 43:65–72.
- Ramadan MF, Amer MMA, Awad A. (2008). Coriander (Coriandrum sativum L.) seed oil improves plasma lipid profile in rats fed diet containing cholesterol. Eur Food Res Technol 227:1173–82.
- Ramadan MF, Asker MMS, Tadros M. (2012). Antiradical and antimicrobial properties of cold-pressed black cumin and cumin oils. Eur Food Res Technol 234:833–44.
- Ramadan MF, Asker MMS, Tadros M. (2013). Lipid profile, antiradical power and antimicrobial properties of Syzygium aromaticum oil. Grasas Aceites 64:509–20.
- Ramadan MF, Kinni SG, Seshagiri M, Mörsel J-T. (2010). Fat-soluble bioactives, fatty acid profile and radical scavenging activity of Semecarpus anacardium seed oil. J Am Oil Chem Soc 87:885–94.
- Reitman S, Frankel S. (1957). Colorimetric GOT and GPT determination. Am J Clin Pathol 28:56–63.
- Sanmugapriya E, Venkataraman S. (2006). Studies on hepatoprotective and antioxidant actions of Strychnos potatorum Linn. seeds on CCl4-induced acute hepatic injury in experimental rats. J Ethnopharmacol 105:154–60.
- Seeram NP, Adams LS, Henning SM, Heber D. (2005). In vitro antiproliferative, apoptotic and antioxidant activities of punicalagin, ellagic acid and a total pomegranate tannin extract are enhanced in combination with other polyphenols as found in pomegranate juice. J Nutr Biochem 16:360–7.
- Shaker E, Khaled RZ, Wajahat ZM, et al. (2011). Comparison of imatinib, nilotinib and silymarin in the treatment of carbon tetrachloride-induced hepatic oxidative stress, injury and fibrosis. Toxicol Appl Pharm 252:165–75.
- Shaker E, Mahmoud H, Mnaa S. (2010). Silymarin, the antioxidant component and Silybum marianum extracts prevent liver damage. Food Chem Toxicol 48:803–6.
- Shim JY, Kim MH, Kim HD, et al. (2010). Protective action of the immunomodulator ginsan against carbon tetrachloride-induced liver injury via control of oxidative stress and the inflammatory response. Toxicol Appl Pharm 242:318–25.
- Shukri R, Mohamed S, Mustapha NM. (2010). Cloves protect the heart, liver and lens of diabetic rats. Food Chem 122:1116–21.
- Tang Y, Gao C, Xing M, et al. (2012). Quercetin prevents ethanol-induced dyslipidemia and mitochondrial oxidative damage. Food Chem Toxicol 50:1194–200.
- Tietz NW, Rinker AD, Shaw LM. (1983). International Federation of Clinical Chemistry. IFCC methods for the measurement of catalytic concentration of enzymes. Part 5. IFCC method for alkaline phosphatase (orthophosphoric-monoester phosphohydrolase, alkaline optimum, EC 3.1.3.1). J Clin Chem Clin Biochem 21:731–48.
- Upur H, Amat N, Blazekovic B, Talip A. (2009). Protective effect of Cichorium glandulosum root extract on carbon tetrachloride-induced and galactosamine-induced hepatotoxicity in mice. Food Chem Toxicol 47:2022–30.
- Vitaglione P, Morisco F, Caporaso N, Fogliano V. (2004). Dietary antioxidant compounds and liver health. Crit Rev Food Sci Nutr 44:575–86.
- Wan XY, Luo M, Li XD, He P. (2009). Hepatoprotective and antihepatocarcinogenic effects of glycyrrhizin and matrine. Chem Biol Interact 181:15–19.
- Yeh Y-H, Hsieh Y-L, Lee Y-T. (2013). Effects of yam peel extract against carbon tetrachloride-induced hepatotoxicity in rats. J Agric Food Chem 61:7387–96.
- Yousef MI, Omar SAM, El-Guendi MI, Abdelmegid LA. (2010). Potential protective effects of quercetin and curcumin on paracetamol-induced histological changes, oxidative stress, impaired liver and kidney functions and haematotoxicity in rat. Food Chem Toxicol 48:3246–61.