Abstract
Context: Muntingia calabura L. (family Muntingiaceae) and Melastoma malabathricum L. (family Melastomaceae) are traditionally used to treat gastric ulcer.
Objective: The present study determines the mechanisms of gastroprotective activity of the chloroform extract of leaves obtained from both the plants using several in vitro and in vivo assays.
Materials and methods: Phytochemical screening, HPLC analysis, and antioxidant activity of the respective extract were carried out. Gastroprotective activity was determined using ethanol-induced gastric ulcer assay while the mechanisms of gastroprotection were determined using the pyloric ligation assay. The test solutions [8% Tween-80 (vehicle), 20 mg/kg omeprazole, and different doses of extracts (50, 250, or 500 mg/kg] were administered orally once daily for 7 consecutive days before the animals were subjected to ethanol induced gastric ulcers.
Results: The chloroform-extracted M. calabura (CEMC) contains tannins, polyphenolics, triterpenes, and steroids while the chloroform-extracted M. malabathricum (CEMM) contains only triterpenes and steroids. CEMC, but not CEMM, exerted remarkably strong antioxidant activity in the 2,2-diphenyl-1-picrylhydrazyl (DPPH)- (86% versus 16%) and superoxide- (73% versus 36%) radical scavenging assays. Both extracts demonstrated significant (p < 0.05) gastroprotection with the EC50 value recorded at 192.3 or 297.7 mg/kg, respectively. In the pylorus ligation assay, CEMC and CEMM significantly (p < 0.05) reduced the total and free acidity and volume; while increased the pH of gastric juice as well as the gastric wall mucus content in comparison with the vehicle-treated group.
Discussion and conclusion: CEMC and CEMM exert gastroprotective effects in animals with ethanol-induced gastric ulcers via antioxidant and anti-secretory effects.
Introduction
One of the research fields within plant-based drug that have received much attention among scientists nowadays is discovery of new gastroprotective agents. The management of advanced peptic ulcers used to be a nightmare and, in most cases, surgery has been the only option left (Borelli & Izzo, Citation2000). Peptic ulcers and gastric ulcers are the common disorders of gastrointestinal tract, occurs as a result of an imbalance between the offensive (i.e., acid, pepsin, and H. pylori) and defensive factors (i.e., mucin, prostaglandin, bicarbonate, nitric oxide, and growth factors) (Rang et al., Citation2012). Various factors such as stress, smoking, nutritional deficiencies, alcoholism, and frequent intake of non-steroidal-anti-inflammatory drugs (NSAIDs) contribute to the development of peptic ulcer (Cemek et al., Citation2010). Despite a well-targeted therapeutic approaches, the effectiveness of various classes of currently available gastroprotective drugs (i.e., proton pump inhibitors, H2-blockers, antacids, and anticholinergics) are surpassed by their adverse side effects which include hypergastrinemia, hypersensitivity, gynecomastia, impotence, arrhythmia, and blood dyscrasias such as thrombocytopenia and enteric infections (Clostridium difficile) (Cemek et al., Citation2010; Rang et al., Citation2012). Scientists working in this field have continued their search to discover new gastroprotective drugs with less or, possibly no, side effects.
In our laboratory, Muntingia calabura L. (family Muntingiaceae) and Melastoma malabathricum L. (family Melastomaceae) have been studied extensively for the pharmacological properties. The leaves of M. calabura, in particular, have been used in Peruvian traditional medicine and Malay folklore medicine. Muntingia calabura possesses several medicinal values such as reducing gastric ulcer and swelling of prostate gland, and relieving headache and cold (Mahmood et al., Citation2014). The leaf of M. malabathricum had been claimed to treat wound, dysentery, diarrhea, ulcer, gastric ulcer, hemorrhoids, fever, and to prevent scarring from smallpox in the Malay, Indian, and Indonesian traditional medicine (Mohd. Joffry et al., Citation2012). Scientifically, both plants have been reported to exert antibacterial, antidiabetic, hypotensive, antiviral, antioxidant, cytotoxicity, antiproliferative, anticoagulant, inhibition of platelet-activating factor, wound healing, gastroprotective, antidiarrheal, anti-inflammatory, antinociceptive, and antipyretic activities at different doses/concentrations (Mahmood et al., Citation2014; Mohd. Joffry et al., Citation2012). Taking into account that both plants have been traditionally used to treat ulcer and the fact that the extracts of both plants exerted antioxidant and anti-inflammatory activities, the present study was carried out to determine the gastroprotective potential of chloroform extract, which represent the lipid-soluble extract, of M. calabura and M. malabathricum leaves.
Materials and methods
Drugs and chemicals
Absolute ethanol was purchased from Fisher Scientific (Pittsburgh, PA); ranitidine, omeprazole, and dimethylsulfoxide (DMSO) were purchased from Sigma Chemical Co. (St. Louis, MO). All other reagents used for the experiments were of analytical grade. All drugs and reagents were prepared fresh before use.
Plant materials
The leaves of M. calabura and M. malabathricum were collected from their natural habitat in Shah Alam, Selangor, Malaysia, between May and August 2012. The identification of the plants was performed by Dr. Shamsul Khamis, a botanist from the Institute of Bioscience (IBS), Universiti Putra Malaysia (UPM), Serdang, Selangor, Malaysia. A voucher specimen for M. calabura (SK2198/13) and M. malabathricum (ACP0017) have been deposited at the herbarium of the Laboratory of Natural Products, IBS, UPM.
Preparation of chloroform extracts
Preparation of chloroform extract of M. calabura (CEMC) and M. malabathricum (CEMM) leaves was carried out as previously described by Zakaria et al. (Citation2006, Citation2007). Briefly, matured leaves (500 g) of each plant were air-dried at room temperature (27 ± 2 °C) for 1–2 weeks and then ground into coarse powder. The powder was soaked in chloroform at a ratio of 1:20 (w/v) for 72 h and this procedure was repeated for another two times. The supernatants were filtered using cotton wools followed by Whatman No. 1 filter papers wherein each of the filtrate was collected and evaporated in a rotary evaporator (Buchi Rotavapor R210/215, Buchi Lab, Flawil, Switzerland) at 40 °C under reduced pressure.
Phytochemical screening of CEMC and CEMM
The phytochemical screening of both extracts was performed according to the conventional protocols as adopted by Ikhiri et al. (Citation1992).
Detection of flavonoids
Each sample (10 g) was separately boiled for 2–3 min in 100 ml of water in a water-bath. To 3 ml of the filtrate, 3 ml of acid–alcohol (ethanol:water:concentrated hydrochloric acid in the ratio of 1:1:1), solid magnesium (1 cm) and 1 ml of t-amyl-alcohol were added. The mixtures were then observed for a color change of rose-orange or violate.
Detection of triterpenes
Each sample (1 g) was separately extracted using ether over 24 h. The filtrate (1 ml) was evaporated to dryness and the residue was redissolved with several drops of acetic anhydride and then sulfuric acid. The mixtures were then observed for a green color change.
Detection of tannins
Each sample (0.2 g) was separately boiled in 5 ml of water. The mixtures were cooled and filtered. A few drops (3 drops) of 5% ferric chloride solution were added to the filtrate and observed for formation of a blue-black precipitate.
Detection of alkaloids
Each sample (0.5 g) was separately boiled with 10 ml of dilute hydrochloric acid (alcoholic) in a test tube for 5 min. The mixtures were cooled and the debris was allowed to settle. The supernatant was filtered into another test tube and 1 ml of each filtrate was tested with three drops of Dragendorff ’s reagent (potassium bismuth iodide solution). The mixture was shaken and observed for the appearance of an orange-red spot and a precipitate formation.
Detection of saponins
Each sample (0.2 g) was mixed with water and persistent froth formation was observed.
HPLC profiling of CEMC and CEMM
The HPLC profile of CEMC and CEMM was determined using the method developed according to the procedures described by Mamat et al. (Citation2013) with slight modifications at the Laboratory of Phytomedicine, Medicinal Plants Division, Forest Research Institute of Malaysia (FRIM), Kepong, Malaysia. In brief, 10 mg of each dried crude extract was dissolved in 1 ml methanol and filtered through a membrane filter with pore size of 0.45 μm prior to analysis. A HPLC system (Waters Delta 600 with 600 Controller, Waters Corp., Milford, MA) with photodiode array detector (Waters 996) (Waters Corp., Milford, MA) was used. A Phenomenex Lunacolumn (4.6 mm i.d. × 250 mm) was used (Phenomenex, Torrance, CA). Two solvents denoted as A and B were used for elution of the constituents. Solvent A was the aqueous mobile phase with 0.1% formic acid and solvent B was acetonitrile. Initial conditions were 95% A and 5% B with a linear gradient reaching 25% B at t = 12 min. This was maintained for 10 min. At t =22 min, B was reduced to 15%, which was then maintained until t = 30 min. The program returned to the initial solvent composition at t = 35 min. The flow rate used was 1.0 ml/min and the injection volume was 10 μl. The column oven was set at 27 °C and the eluent was monitored at 254, 300, and 366 nm. The retention time and UV spectra of major peaks were analyzed. Each extract chromatogram was then spiked separately or in combination with a list of flavonoid compounds, which served as the standard, namely quercitrin, dihydroquercitin, fisetin, quercetin, rutin, naringenin, silibinin, genistein pinostrobin, hesperetin, flavanone, 4′,5,7-trihydroxy flavanone, and 2,4,4′-trihydroxychalcone using the same solvent system in order to determine the presence of these constituents in the extract.
Antioxidant activity of CEMC and CEMM
2,2-Diphenyl-1-picrylhydrazyl radical scavenging assay
The antioxidant activity of both extracts was determined via their ability to scavenge the 2,2-diphenyl-1-picrylhydrazyl (DPPH) radicals according to the method of Blois (Citation1958) with slight modification using microplate system (MRX Microplate Reader, Dynex Technologies, Chantilly, VA). Fifty microliter of sample (1.0 mg/ml) was added to 50 μL of DPPH (FG: 394.32) (1.0 mM in ethanolic solution) and 150 μl of absolute ethanol in a 96-well microtiter plate. Samples were prepared in triplicates. The plate was shaken for 15 s at 500 rpm and left to stand at room temperature for 30 min. The absorbance of the resulting solution was measured spectrophotometrically at 520 nm.
Superoxide anion radical scavenging assay
The antioxidant activity of both extracts was further determined via their ability to scavenge superoxide anion radicals according to the modified method of Chang et al. (Citation1996). Briefly, nitro-blue tetrazolium (NBT) solution (100 ml of 4.1 mM/l) was prepared by mixing 3.15 g Tris-HCl, 0.1 g MgCl2, 15.0 mg 5-bromo-4-chloro-3-indolyl phosphate, and 34.0 mg 4-nitro blue tetrazolium chloride to 100 ml of distilled water. The reaction mixture (100 ml) was prepared by dissolving 0.53 g sodium carbonate (pH 10.2), 4.0 mg ethylene diamine tetraacetic acid (EDTA), and 500 mg xanthine in 0.025 mM NBT solution. The mixture was refrigerated at 4 °C. The reaction mixture (999 μl) was transferred into a microcuvette and placed in a 25 °C spectrophotometer cell holder. Superoxide generation was instigated by adding 1.0 μl of xanthine oxidase (XOD) (20 U/ml). The optical density (OD) was measured at 560 nm for 120 s using Lambda 2 S spectrophotometer (Perkin-Elmer, Norwalk, CT). Each extract was suspended in the reaction mixture at the concentration of 200 μg/ml. The stock solution (200 μl) was mixed with 799 μl of the reaction mixture and placed in a cell holder to autozero. One microliter of XOD (20 U/ml) was then added, mixed thoroughly and similarly measured for the XOD curves.
Oxygen radical absorbance capacity assay
Further analysis on the antioxidant capacity of both extracts was carried out using the oxygen radical absorbance capacity (ORAC) assay as described by Huang et al. (Citation2002) but with some modifications. 2,2′-Azobis(2-amidinopropane) dihydrochloride (AAPH) (0.65 g) was dissolved in 10 ml of 75 mM phosphate buffer (pH 7.4) to a final concentration of 240 mM (made fresh). A fluorescein stock solution (1 mM), prepared in 75 mM phosphate buffer (pH 7.4), was wrapped in foil and stored at 5 °C. Prior to use, the stock solution was immediately diluted to the ratio of 1:100 000 using the 75 mM phosphate buffer. Sodium fluorescein, which was prepared fresh daily, was added (150 μl) to the interior experimental wells while the blank wells received 25 μl of Trolox dilution and the sample wells received 25 μl samples. The plate was incubated for 10 min at 37 °C to allow equilibrium. BMG Omega Fluostar Fluorescent Spectrophotometer (Omega Inc., New York, NY) with injector was used with an excitation filter of 485 nm bandpass and emission filter of 528 nm bandpass. The plate reader was controlled by MARS data analysis software (BMG LABTECH Ltd., Saitama City, Japan). Reactions were initiated by the addition of 25 μl of AAPH solution (240 mM) using the microplate reader’s injector to achieve a final reaction volume of 200 μl followed by shaking at maximum intensity for 50 s. The fluorescence of each well was then monitored kinetically with data taken by top reading every 60 s. ORAC values were calculated using MARS Data Analysis Reduction Software (BMG LABTECH Ltd., Saitama City, Japan).
Total phenolic content of CEMC and CEMM
Both extracts were also subjected to the determination of total phenolic content (TPC) using the Folin–Ciocalteu reagent based on slightly modified method of Singleton and Rossi (Citation1965). Each extract (1 mg) was prepared using 1.0 ml of 80% methanol containing 1.0% hydrochloric acid and 1.0% of distilled water at room temperature on the shaker set at 200 rpm. The mixture was centrifuged at 6000 rpm for 15 min, and the supernatant transferred into vials prior to the TPC determination. Approximately 200 μl of supernatant extract was mixed with 400 μl of Folin–Ciocalteu reagent (0.1 ml/0.9 m) and incubated at room temperature for 5 min. This was followed by the addition of 400 μl of sodium bicarbonate (60.0 mg/ml) and the mixture was incubated at room temperature for 90 min. Absorbance was measured at 725 nm wherein the calibration curve was generated using the gallic acid standard OD. The TPC levels found in the samples were expressed as gallic acid equivalent (GAE)-TPC mg/100 g.
Experimental animals
All in vivo experiments were performed on male Sprague–Dawley rats (180–200 g; 8–10 weeks old) obtained from the Animal Unit, Faculty of Medicine and Health Sciences, UPM, Malaysia. The animals were caged in polypropylene cages with wood shaving and kept at room temperature (27 ± 2 °C; 70–80% humidity; 12 h light/darkness cycle) in the Animal Holding Unit (UPM). They were fed with standard pellet and allowed free access to water. The rats were fasted for 24 h prior to all assays. The vehicle (8% Tween-80), standard drugs (100 mg/kg ranitidine or 20 mg/kg omeprazole) or extracts (CEMC or CEMM; 50, 250, and 500 mg/kg) was administered orally (p.o.) using oral gavage. The use of animals in the following study was approved by the Animal Care and Used Committee (ACUC) of Faculty of Medicine and Health Sciences, UPM (Approval no. UPM/FPSK/PADS/BR-UUH/00474).
Gastric ulcer assays
Ethanol-induced gastric ulcers
The experiment was performed according to the method described by Balan et al. (2013). The rats were divided indiscriminately into 11 groups (n = 6) comprising of three doses for each fraction, positive control and the vehicle group. The rats were administered vehicle (8% Tween 80; 10 ml/kg), ranitidine (100 mg/kg), or extracts (CEMC or CEMM; 50, 250, and 500 mg/kg) orally for seven consecutive days. On the 7th day, the rats were induced with gastric ulceration by oral administration of absolute ethanol (5 ml/kg) after 1 h of treatment. The animals were then anesthetized using light ether and euthanized by cervical dislocation 1 h after ulcer induction.
Macroscopic analysis
The stomachs were removed and dissected along the greater curvature to determine the lesion damage. Every opened and spread-out stomach was photographed and the ulcer area was measured by superimposing transparent grid paper with minimum square equal to 1 mm2 (Bodhankar et al., Citation2006). The ulcer area (UA) in mm2 was determined for each stomach in the group. Percentage of protection conferred by each extract was calculated using the following formula:
Microscopic analysis
Gastric tissue samples from each group were collected and fixed in 10% formalin. The samples were then embedded in paraffin after the tissue was processed. This was followed by sectioning (3–5 μm) and staining with hematoxylin and eosin dye. The sections were viewed and analyzed using light microscopy and photographed.
Pylorus ligation induced ulceration
To explore some of the possible mechanisms of gastroprotective modulated by each extract, CEMC and CEMM were subjected to the pylorus ligation assay as described by Shay et al. (Citation1945) but with slight modifications. Briefly, 48 rats were divided into eight groups wherein Group-I (control) was treated (p.o) with vehicle (8% Tween 80), Group-II (positive control) was treated with 100 mg/kg ranitidine (p.o), Group-III, -IV, and -V were treated (p.o) with CEMC (50, 250, and 500 mg/kg, respectively), and Group-VI, -VII, and -VIII were treated (p.o) with CEMM (50, 250, and 500 mg/kg, respectively). The procedure of pylorus ligation assay was carried out 1 h after the administration of test compounds into the rats. Under light anesthesia induced by 100 mg/kg ketamine HCl (intramuscular (i.m.)) and 16 mg/kg xylazine HCl (i.m.), a 2 cm long incision was prepared in the abdomen just below the sternum. The stomach was exposed, and a thread was passed around the pyloric sphincter and tied in a tight knot. Care was taken while suturing the pyloric sphincter to avoid the blood vessels. The abdomen was then sutured and the skin was cleaned of any blood spots or bleeding. The rats were sacrificed 6 h after ligation by cervical dislocation, the stomachs were removed, and the contents were drained out, collected, and centrifuged. The stomach was opened along the greater curvature to determine the lesion damage as described by Balan et al. (2013). The percentage of protection was calculated using the same formula given earlier.
Determination of volume, pH, free, and total acidity of gastric content
The gastric content of rats stomach pretreated with vehicle, ranitidine, CEMC or CEMM, was drained and then centrifuged at 2500 rpm for 10 min followed by the determination of the volume, pH and acidity (free and total) of the gastric juice (Srivastava et al., Citation2010). The free acidity of the respective gastric content was measured via titration of the test solutions containing methyl orange reagent using 0.01 N NaOH until the color of the solution became yellowish. The volume of alkali added was noted. Then, 2–3 drops of phenolphthalein was added and the solution was titrated until a definite pink color appears. The total volume of NaOH added was noted and this corresponds to total acidity. Acidity was calculated using the following formula:
Estimation of gastric wall mucus content
The ability of CEMC and CEMM to affect the gastric wall mucus content was evaluated using the slightly modified method by Corne et al. (Citation1974). Briefly, the stomach was opened along the greater curvature, weighed, and immersed in 10 ml of 0.1% Alcian Blue prepared in 0.16 M sucrose/0.05 M sodium acetate (pH 5.8) for 2 h. The stomach was then successively rinsed twice in 0.25 M sucrose solution (15 min each) to remove the excessive dye. The remaining dye, which forms a complex with the gastric mucus, was extracted with 0.5 M MgCl2 for 2 h and shaken occasionally for 1 min in every 30 min interval. The blue extract formed was then shaken vigorously with an equal volume of diethyl ether. The samples were centrifuged at 3600 rpm for 10 min after the formation of emulsion was observed. The OD of Alcian Blue in the aqueous layer was read at 580 nm using a spectrophotometer. The quantity of Alcian blue-bind gastric mucus per gram wet stomach was then calculated from a standard curve.
Statistical analysis
The results were expressed as mean ± S.E.M. and analyzed using one-way analysis of variance (ANOVA), followed by Dunnett’s post hoc test. Results were considered significant when p < 0.05.
Results
Phytochemical screening of CEMC and CEMM
Phytochemical analysis of CEMC and CEMM was presented in . CEMC was found to contain phytoconsituents like tannins, triterpenes, and steroids while CEMM contained only triterpenes and steroids.
Table 1. Comparison on the phytochemical constituents between the dried leaves of chloroform extracts of M. calabura (MEMC) and M. malabathricum with their respective extract, MEMC or (MEMM).
HPLC profiling of CEMC and CEMM
The HPLC profiles of CEMC () and CEMM () demonstrated the presence of several major peaks at various wavelengths (i.e., 210, 254, 280, 300, 330, and 366 nm). For CEMC, two major peaks were recorded at the wavelength of 330 nm, which are at the retention time of 20.729 and 22.124 min. Further analysis demonstrated that the two peaks exert their λmax value in the region of 241.3–289.8 and 324.3 nm (). For CEMM, five major peaks were also detected at the wavelength of 330 nm, which are at the retention time of 20.678, 21.219, 22.083, 23.446, and 26.384 min. Further analysis demonstrated that the first three peaks exert their λmax value in the region of 285.1; while for peaks 3 and 4, the λmax fall in the region of 244.9 and 196.7–241.3 nm, respectively (). Based on the λmax value of the detected peaks, several flavonoid-based compounds were suggested to be part of the phytoconstituents of the respective MEMC and MEMM. To confirm this suggestion, the chromatogram of MEMC and MEMM was compared with the respective chromatogram of several pure flavonoids available in the laboratory (i.e., rutin, fisetin, quercetin, quercitrin, naringenin, genistein, pinostrobin, and hesperetin). Rutin was detected in both extracts while pinostrobin was only detected in MEMM ().
Figure 1. (A) HPLC profiles of CEMC at different wavelengths. (B) HPLC profiles of CEMM at different wavelengths. (C) HPLC chromatograms of CEMC and CEMM compared to rutin and dihydro-quercitin at the wavelength of 254 nm. Rutin and possibly dihydroquercitin were detected in CEMM, but not CEMC.
Antioxidant activity of CEMC and CEMM
The antioxidant potential of CEMC and CEMM assessed using various antioxidant assays are shown in . CEMC exerted high while CEMM showed low antioxidant activity when assessed using the DPPH- or superoxide anion-radical scavenging assay. The percentage of antioxidant for CEMC and CEMM against the former assay was approximately 86% and 16%; while for the latter assay, the percentage of antioxidant was 73% and 36%, respectively. According to the standard procedures, an activity is considered high if the percentage of radical scavenging activity was above 70% and is considered low if the percentage of radical scavenging activity was below 50%. In addition, CEMC was also found to exert the highest antioxidant activity in comparison to CEMM when assessed using the ORAC assay. Although CEMC contain almost 10-fold higher TPC value in comparison to CEMM, both extracts were still considered to have low TPC value. This suggestion was based on the standard TPC procedure that assumed a compound/an extract to have a high TPC value if the value recorded is >1000 mg gallic acid equivalent (GAE)/100 g.
Table 2. The antioxidant profiles and TPC values of CEMC and CEMM, at the concentration of 200 μg/ml, as assessed using the respective oxidative assays.
Effect of CEMC and CEMM against ethanol-induced gastric ulcer in rats
The effects of CEMC and CEMM against ethanol-induced gastric ulcer in rats are shown in . Pre-treatment with vehicle was found to successfully produce severe gastric ulceration as indicated by the increase in the ulcer score and ulcer area reading. Pre-treatment with ranitidine significantly (p < 0.05) reduced the gastric ulcer formation indicated by decrease in both the ulcer score and ulcer area when compared with the vehicle-treated group. Interestingly, pre-treatment with CEMC and CEMM, at all doses, produced a highly significant (p>0.05) and dose-dependent gastroprotective activity with the recorded percentages of protection ranging between 11.8–100% and 13.0–95%, respectively.
Table 3. Effects of CEMC or CEMM on ethanol-induced gastric ulcer model in rats.
Macroscopic and microscopic analysis of CEMC and CEMM effect against ethanol-induced gastric ulcer
Macroscopic analysis of the stomach pretreated with various test solutions is shown in . Pre-treatment with vehicle (8% Tween 80; ) produced severe gastric ulcer formation characterized by the formation of severe hemorrhagic streaks on the surface of the stomach in comparison to the stomach of the normal controls (untreated; ). Interestingly, pretreatment with 100 mg/kg ranitidine (), CEMC (), or CEMM (Figure E21–2E3) caused marked reduction in the formation of gastric ulcer tissues, which is further supported by the decrease in hemorrhagic streaks appearance.
Figure 2. Macroscopic analysis of the rat stomach pre-treated with the respective test solution followed by the ethanol-induced gastric ulcer formation. (A) Normal untreated stomach shows normal surface appearance and absence of ulcer formation. (B) Pretreatment with vehicle (8% Tween 80; negative control) shows severe hemorrhagic ulcer and lesions of the surface of the stomach. (C) Pretreatment with 100 mg/kg ranitidine (positive control) shows almost normal surface appearance with slight gastric ulcer formation indicated by reduced in hemorrhagic tissue appearance. (D1) Pretreatment with 50 mg/kg CEMC shows severe gastric ulcer formation and marked hemorrhagic streaks appearance. (D2) Pretreatment with 250 mg/kg CEMC shows mild gastric ulceration and reduction in hemorrhagic streaks appearance. (D3) Pretreatment with 500 mg/kg CEMC shows complete absence of ulcer formation and lack of hemorrhagic streaks appearance. (E1) Pretreatment with 50 mg/kg CEMM shows severe ulceration and marked hemorrhagic streaks appearance. (E2) Pretreatment with 250 mg/kg CEMM shows mild gastric ulcer formation and marked reduction in hemorrhagic streaks appearance. (E3) Pretreatment with 500 mg/kg CEMM shows complete absence of gastric ulcer formation and marked decrease in hemorrhagic streaks appearance.
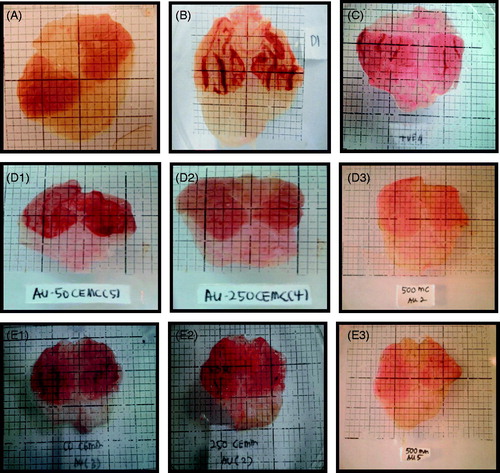
Microscopic analysis of the stomach tissues pretreated with various test solutions is shown in for magnification ×10 and for magnification ×40. Pre-treatment with vehicle (8% Tween 80; ) produced a total disruption of the epithelial layer (EL) of the mucosa and gastric pits (GP) with the presence of inflammatory cells (i.e., neutrophils (N)) in comparison with the gastric tissue in normal controls (untreated; ), which exhibits architecture of a normal mucosa layer (M) showing the presence of gastric glands, muscularis mucosa (MM) and gastric pits (GP). Pretreatment with 100 mg/kg ranitidine () shows irregular architecture of the epithelial surface of mucosa probably due to mild injury induced by ethanol undergoing the recovery phase. Pretreatment with CEMC () and CEMM () were found to improve the architecture of the gastric tissue in a dose-dependent manner with marked gastroprotective effects started at the dose of 250 mg/kg.
Figure 3. (A) Microscopic analysis at the ×10 magnification of the rat stomach pre-treated with the respective test solution followed by the ethanol-induced gastric ulcer formation. (i) Gastric tissue of normal (untreated) group shows mucosa layer (M) consist of gastric glands, which extend from the level of muscularis mucosa (MM) to open into the stomach lumen via gastric pits (GP). (ii) Gastric tissue of vehicle (negative control)-treated group shows a total disruption of the epithelial layer (EL) of the mucosa and GP. (iii) Gastric tissue of 100 mg/kg ranitidine (positive control)-treated group shows the irregular architecture of the epithelial surface of mucosa, which is still in the recovery phase. (iv) Gastric tissue of 50 mg/kg CEMC-treated group shows the total disruption of EL indicated by the slough off of the mucosa lining that had occurred in a few places. Structure of GP was also disrupted and the mucosa layer show presence of inflammatory cells. (v) Gastric tissue of 250 mg/kg CEMC-treated group shows that the EL is undergoing recovery indicated by the tissue architecture that resemble the normal lining of stomach. The cells in the mucosa were not disrupted as the lesions on the surface are fewer. (vi) Gastric tissue of 500 mg/kg CEMC-treated group shows that the EL lining the stomach was almost in its normal form and lesions are hardly found at the mucosa layer. (vii) Gastric tissue of 50 mg/kg CEMM-treated group shows mild disruption of the EL with the slough off of the surface of the mucosa. Edema is present at the MM layer. (viii) Gastric tissue of 250 mg/kg CEMM-treated group shows that the mucosa layer is highly populated with H and N. Morphology of the cells were also changed and did not resemble normal structure of cells. (ix) Gastric tissue of 500 mg/kg CEMM-treated group shows improvement in the structure of the mucosa with the morphology of the cells resembles those of the normal untreated cells. The surface of EL shows better lining at the top of mucosa layer (Hematoxylin & eosin; magnification ×10). (B) Microscopic analysis at the ×40 magnification of the rat’s stomach pre-treated with the respective test solution followed by the ethanol-induced gastric ulcer formation. (i) Gastric tissue of normal (untreated) group shows that the gastric glands contain mixed population of cells such as parietal cell (P), which is acid-secreting cells and chief cells (C) that are located towards the bases of the gastric glands. Mucus will line the GP in which gastric gland will open. (ii) Gastric tissue of vehicle (negative control)-treated group shows a high number of neutrophils (N) and hemorrhage (H) within the mucosa layer. (iii) Gastric tissue of 100 mg/kg ranitidine (positive control)-treated group shows the presence of inflammatory exudates mainly N and mild H within the mucosa layer. (iv) Gastric tissue of 50 mg/kg CEMC-treated group shows the presence of H and N, which can be seen clearly and mostly reside at the base of the mucosa layer. (v) Gastric tissue of 250 mg/kg CEMC-treated group also shows that N were present at the base of the mucosa layer with the absence of H. (vi) Gastric tissue of 500 mg/kg CEMC-treated group shows normal structure of the P and C cells with the absence of H and inflammatory exudates. (vii) Gastric tissue of 50 mg/kg CEMM-treated group shows the presence of H and N, which were found mainly at the base of the mucosa layer. (viii) Gastric tissue of 250 mg/kg CEMM-treated group shows the presence of low number of N and H that were mainly located at the base of mucosa layer. (ix) Gastric tissue of 500 mg/kg CEMM-treated group shows the least number of N and H, and the lining of the mucosa had almost the same architecture as the normal stomach (hematoxylin & eosin; magnification ×40).
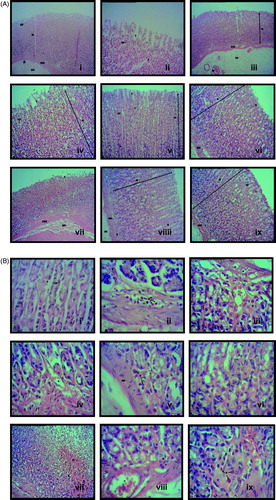
Effect of CEMC and CEMM on stomach in pyloric ligation-induced gastric ulcer model
The effects of CEMC and CEMM on pyloric ligation-induced gastric ulcer in rats are summarized in . Groups treated with CEMC or CEMM, at all doses, produced a significant (p < 0.05) decrease in the volume, but increase in the pH of gastric juice when compared with the vehicle-treated group. Both activities occur in a dose-independent manner. Both extracts were also found to reduce the total and free acidity of the gastric juice in a dose-dependent manner. Moreover, CEMC and CEMM, at all doses, significantly (p < 0.05) increase the gastric wall mucus concentration in a dose-dependent manner.
Table 4. Effects of CEMC or CEMM on pylorus ligation-induced gastric ulcer model in rats.
Discussion
The utilization of various herbal medicines in the treatment of various disorders is now generally acknowledged. Global concern on natural products as preventive and therapeutic mediators has led to a greater approval of the wealthy legacy of traditional systems of medicine (Gill et al., Citation2009). The plant-based natural products are generally believed to be safer in contrast to the synthetics products. These compounds can be used directly as lead for the improvement of new medicines or as pharmacological means to discover new active compounds, subsequently they can be life-saving or resolving the value of life in long-lasting maladies (Rates, 2001). For over a century, peptic ulcer disease has been one of the leading causes of gastrointestinal surgery, with high morbidity and mortality rates. The incidence of duodenal ulcers is dominant in Western inhabitants and gastric ulcers are more common in Asia (de Sousa Falcão et al., Citation2008; Yuan et al., Citation2006). The management of peptic ulcers by means of plant-based natural products and the protection of induced gastric ulcer in laboratory animals using medicinal plants have been reported elsewhere (Disi et al., Citation1998; Muralidharan & Srikanth, Citation2009).
In the present study, the gastroprotective activity of chloroform extract of M. calabura and M. malabathricum leaves were investigated as part of our aims to establish the pharmacological potential of those plants. Various pharmacological activities have been reported for each of the plant as described earlier, which include the gastroprotective activity of methanol extract of the respective plant (Balan et al., Citation2014; Zabidi et al., Citation2012). Since methanol have the ability to extract both the polar and non-polar bioactive compounds, further conclusion on the involvement of those compounds in the observed gastroprotective activity could not be made. Therefore, the present study was carried out using chloroform extract, which only extracted the non-polar compounds, to see whether the non-polar compounds in M. calabura and M. malabathricum leaves possess gastroprotective activity. Based on the results obtained, the non-polar bioactive compounds present in CEMC and CEMM did possess gastroprotective activity when assessed using the ethanol-induced gastric ulcer model in rats. This finding suggested that the gastroprotective potential exerted by M. calabura and M. malabathricum involved, in part, modulation by the non-polar bioactive compounds.
Various mechanisms are believed to take part in the formation of gastric ulcer in different experimental models (Bose et al., Citation2003; Parmar & Desai, Citation1993). Therefore, it is unfeasible to suggest a single mechanism for gastroprotective effect of a particular drug. Ethanol-induced gastric ulcer assay is a classic ulcer assay and is generally used to determine the gastroprotective activity of natural or synthetic compounds. Ulcer was induced by ethanol possibly via a combination of direct toxic action of ethanol, reduce in the presence of gastric wall mucus and decrease in the bicarbonate secretion (Okoli et al., Citation2009). Through its direct action, ethanol penetrates the gastric mucosa rapidly causing injury to the gastrointestinal mucosa cells and membranes (Kumar et al., Citation2011). These processes of formation of gastric mucosal lesions commence through microvascular injury, increase of the vascular permeability, which affected the bicarbonate and mucus secretion, sodium and potassium flow, pepsin secretion, and the loss of hydrogen ions and histamine into the lumen (Szabo, 1987). Increase in vascular permeability also increases the intracellular membrane permeability to sodium and water causing massive accumulation of calcium that clarifies the pathogenesis of gastric mucosal injury. The development of gastric lesions results in depression of the gastric mucosa defense mechanisms (i.e., the reduction of gastric mucus substance, mucosal cell injury, and damaged mucosal blood flow) (Galani et al., Citation2010; Kinoshita et al., Citation1995). In this assay, the consequential lesions developed following the administration of ethanol become visible as multiple, different sizes of hemorrhagic red streaks along the glandular part of the stomach. Other than that ethanol can also augment the production of reactive oxygen species (ROS) (i.e., superoxide anion and hydroxyl radicals) (Bagchi et al., Citation1998) and affect the release of arachidonate metabolites and histamine, influx of Ca2+, levels of endogenous glutathione and prostaglandin, stimulation of leukotriene C4/D4 (LTC4/D4), and platelet-activating factor (PAF) (Okoli et al., Citation2009; Rachchh & Jain, Citation2008). The ability to trigger the synthesis of ROS was attributed to the metabolism of ethanol and those ROS will play a part in the mechanism of acute and chronic ulceration of the gastric mucosa. ROS, by acting directly or indirectly by triggering lipid peroxidation, will initially attack and damage many biological molecules (e.g., prostaglandins) followed by a continuous chain of reactions that will lead to alteration in membrane permeability of cells resulting in cells damage and eventually cell death (Londonkar & Poddar, Citation2009; Okoli et al., Citation2009).
As observed in the antioxidant study, CEMC, but not CEMM, was able to produce remarkable antioxidant activity in the DPPH- and superoxide anion-radical scavenging and ORAC assays. Based on these findings, it is possible to suggest that the gastroprotective activity showed by CEMC involved the antioxidant and free radical scavenging effects of the extract. However, the high antioxidant activity shown by CEMC was in contrast to its low TPC value suggesting that the antioxidant effect was not influenced by the TPC content of CEMC. Several reports on extracts that demonstrated high antioxidant activity but contained low TPC value supported the present findings (Alali et al., Citation2007; Kaur & Sharma, Citation2014). In addition, the low TPC value of CEMC is in line with the extract’s phytochemical screening that showed low intensity in the presence of polyphenolics and tannins, as well as the absence of flavonoids. Although polyphenolic compounds have been well known to exert antioxidant activity owing to their redox properties, their ability to act like reducing agents and metals chelators, or scavenger of free radicals in CEMC should be ignored due to their low content (Rice-Evans et al., Citation1996). The low concentration or lack of polyphenolics might be due to the type of solvent used in the extraction processes wherein in the present study non-polar solvent, chloroform, was used (Makris & Kefalas, Citation2004). This suggestion was based on report made by Zam et al. (Citation2013) that polyphenolic compounds were best extracted using polar solvent system. The role of anti-inflammatory mechanism in the modulation of gastroprotective activity of CEMC should also be mentioned. CEMC has earlier been reported to exert weak anti-inflammatory activity when assessed using the carrageenan-induced paw edema assay, thus, suggests that the anti-inflammatory activity of CEMC did not contribute in a major way towards its mechanisms of gastroprotective. It is believed that the antioxidant activity of CEMC play a major role in modulating the gastroprotective activity of the extract.
On the contrary, CEMM-produced gastroprotective effect is suggested to involve a non-antioxidant-mediated mechanism based on the fact that the extract exerted a very weak antioxidant effect when tested in all antioxidant assays. This is further supported by the lack of polyphenolic compounds, tannins, and flavonoids in CEMM. One of the possible mechanisms that could contribute to the gastroprotective activity demonstrated by CEMM is the anti-inflammatory activity of the extract, which was proven using the carrageenan-induced paw edema model (Zakaria et al., Citation2006). This suggestion is supported by Nidavani et al. (Citation2014) on the various plants that have been demonstrated to exert anti-inflammatory and gastroprotective activities. The combination of gastroprotective and anti-inflammatory actions of CEMM is tremendously encouraging given the fact that there are serious limitations of a large number of anti-inflammatory agents that demonstrate a propensity to generate gastric irritation, bleeding and mucosal cellular injury (Lima et al., Citation2006; Shiotani & Graham, Citation2002). Furthermore, CEMM might act at the stage wherein anti-inflammatory or other activities (i.e., proton pumping or H2 receptor blocking) instead of antioxidant are required to attenuate the gastric ulcer formation.
Other than the antioxidant and anti-inflammatory actions, several other mechanisms could be postulated to take place based on the assay used in the present study. In the case of ethanol-induced assay, the ability of ethanol to excite LTC4 suggested that CEMC- and CEMM-induced gastroprotective activity by suppressing the lipoxygenase pathway (Nwafor et al., Citation2000). It is also worth to mention that the ability of CEMC and CEMM to inhibit the ethanol-induced gastric ulcer suggested that both extracts might exert a local and non-specific mechanism known as adaptive cytoprotection. According to Bighetti et al. (Citation2005), adaptive cytoprotection may take place as a consequence of the ability of some compounds stimulates prostaglandin production, which is fundamental for mucus and bicarbonate synthesis. Moreover, Morimoto et al. (Citation1991) have earlier demonstrated that ethanol-induced gastric mucosal lesions are not inhibited by antisecretory agents, but by agents that enhance mucosal defensive factors while Okoli et al. (Citation2009) have suggested that compounds, which can help in the recovery of mucosal defensive factors, might be utilized to hamper ethanol effects on gastric mucosa. The ability of CEMC and CEMM to attenuate ethanol effects on gastric mucosa might suggest that both extracts possessed an ability to promote recovery of mucosal defensive factors. Further investigations on the possible mechanisms of gastroprotective adopted by both extracts were performed using the pylorus ligation assay in rats. Digestive effect of the accumulated gastric juice is supposed to be accountable for generating ulcers in the pyloric ligated rats. Besides gastric acid secretion, reflex or neurogenic effect has also been proposed to play an essential task in the development of gastric ulcer in this model (Bose et al., Citation2003; Goswani et al., Citation1997). Since ulcer formation is directly related to factors such as gastric volume, free and total acidity, these parameters were evaluated for anti-ulcer activity (Khayum et al., Citation2009). In the present study, the extracts were found to reduce the volume and total acidity, and at the same time increase the pH, of the gastric juice when administered orally. Moreover, CEMC and CEMM caused significant increase in the concentration of gastric wall mucus. Overall, these results suggest that both extracts possessed a possible local antisecretory action (Burci et al., Citation2013; Lima et al., Citation2006). Omeprazole, as a comparison drug, reduced the volume and acidity while increasing the pH of the gastric juice, and increases the gastric wall mucus content. Both the extracts were thought to resume the role as proton pump inhibitors (i.e., omeprazole and lansoprazole) or histamine H2 receptor antagonists (i.e., ranitidine and cimetidine) due to their ability to inhibit acid secretion possibly by blocking the histamine H2 receptors on the parietal cells (Eriksson et al., 1996; Sachs et al., Citation2006).
It is widely acknowledged that free radicals are involved in the development of ulcers and in ethanol-induced gastric ulcer and pylorus ligation models, increasing the oxidative and decreasing the antioxidative biomarkers (Pihan et al., Citation1988; Rastogi et al., Citation1998). The fact that CEMC possesses remarkable antioxidant and free radicals scavenging activities have been proven in the present study and is, therefore, suggested to play a role in the attenuation of gastric ulcers in both models. These potentials of CEMC are attributed to the presence of tannins and polyphenolics, as well as triterpenes, which are believed to act synergistically to produce remarkable effects. Although CEMM also demonstrated the presence of triterpenes, the extract did not contain tannins and polyphenolics compounds, which might explain its weak antioxidant effects. Moreover, the diversity in pharmacological effects of triterpenoids have been reported by Manosroi et al. (Citation2013) wherein some of the studied triterpenoids were found to produce no activity when assessed using the DPPH radical scavenging assay but still exert anti-inflammatory activity against TPA-induced inflammation in mice and inhibitory action against melanogenesis in B16 melanoma cells induced by α-melanocyte-stimulating hormone (α-MSH) and Epstein–Barr virus early antigen (EBV-EA) activation induced by 12-O-tetradecanoylphorbol 13-acetate (TPA) in Raji cells. This diversity in actions together with the synergistic effects between the different phytoconstituents might support the differences in activity between CEMC and CEMM. The present study also supported previous claim that the chloroform extract mainly contains triterpenoids in major concentration (Itokawa et al., Citation1990). Other than their anti-inflammatory activity, pentacyclic triterpenoids are also known to encourage mucus secretion. Hence, the mucus secreting potential and subsequent anti-ulcer activity of CEMC and CEMM may be linked to the presence of triterpenes (Deoda et al., Citation2011).
In conclusion, CEMC exerts gastroprotective activity possibly via the antioxidant, anti-inflammatory mechanisms and by increasing the gastric mucosa defense while CEMM demonstrates gastroprotective effect plausibly via non-antioxidant, anti-inflammatory actions, and also by increasing the gastric mucosa defense.
Declaration of interest
The authors report that they have no conflicts of interest. The authors thank the Faculty of Medicine and Health Sciences, UPM, Malaysia, for providing the facilities and supporting this study. This research was supported by the Fundamental Research Grant Scheme (FRGS; Reference no. 04-01-14-1445FR) and Transdisciplinary Research Grant Scheme (TRGS; Reference no. 600-RM|/TRGS 5/3 (112014)-), which were awarded by the Ministry of Higher Education (MOHE), Malaysia.
References
- Alali FQ, Tawaha K, El-Elimat T, et al. (2007). Antioxidant activity and total phenolic content of aqueous and methanolic extracts of Jordanian plants: An ICBG project. Nat Prod Res 21:1121–31.
- Bagchi D, Carryl O, Tran M. (1998). Stress, diet and alcohol-induced oxidative gastrointestinal mucosal injury in rats and protection by bismuth subsalicylate. J Appl Toxicol 18:3–13.
- Balan T, Mohd Sani MH, Velan S, et al. (2014). Antiulcer activity of methanol extract of Muntingia calabura leaves and the possible mechanisms of action involved. Pharm Biol 52:410–18.
- Bighetti AE, Antônio MA, Kohn LK, et al. (2005). Antiulcerogenic activity of a crude hydroalcoholic extract and coumarin isolated from Mikania laevigata Schultz Bip. Phytomedicine 12:72–7.
- Blois MS. (1958). Antioxidant determinations by the use of a stable free radical. Nature 181:1199–200.
- Bodhankar SL, Jain BB, Ahire BP, et al. (2006). The effect of rabeprazole and its isomers on aspirin and histamine-induced ulcers in rats. Indian J Pharmacol 38:357–8.
- Borelli F, Izzo AA. (2000). The plant kingdom as a source of anti-ulcer remedies. Phytother Res 14:581–91.
- Bose M, Motghare VM, Dakhale GN, et al. (2003). Antiulcer activity of levcromakalim and nicorandilin albino study. Pol J Pharmacol 55:91–95.
- Burci LM, Pereira IT, da Silva LM, et al. (2013). Antiulcer and gastric antisecretory effects of dichloromethane fraction and piplartine obtained from fruits of Piper tuberculatum Jacq. in rats. J Ethnopharmacol 148:165–74.
- Cemek M, Yilmaz E, Büyükokuroğlu ME. (2010). Protective effect of Matricaria chamomilla on ethanol-induced acute gastric mucosal injury in rats. Pharm Biol 48:757–63.
- Chang WS, Lin CC, Chiang HC. (1996). Superoxide anion scavenging effect of coumarins. Am J Chin Med 24:11–17.
- Corne SJ, Morrisey SM, Woods RJ. (1974). A method for the quantitative estimation of gastric barrier mucus. J Physiol 242:116–17.
- de Sousa Falcão H, Leite JA, Barbosa-Filho JM, et al. (2008). Gastric and duodenal antiulcer activity of alkaloids: A review. Molecules 13:3198–223.
- Deoda RS, Kumar D, Bhujbal SS. (2011). Gastroprotective effect of Rubia cordifolia Linn. on aspirin plus pylorus-ligated ulcer. Evid-Based Complement Alternat Med eCAM 2011:541624. [Epub ahead of print]. doi:10.1155/2011/541624.
- Disi AM, Tamimi SO, Abuereish GM. (1998). Effects of Anchusa strigosa root aqueous extract on gastric ethanol-induced ulcer in laboratory animals. J Ethnopharmacol 60:189–98.
- Eriksson S, Långström G, Rikner L, et al. (1995). Omeprazole and H2-receptor antagonists in the acute treatment of duodenal ulcer, gastric ulcer and reflux oesophagitis: A meta-analysis. Eur J Gastroenterol Hepatol 7:467–75.
- Galani VJ, Goswami SS, Shah MB. (2010). Antiulcer activity of Trichosanthes cucumerina Linn. against experimental gastro-duodenal ulcers in rats. Orient Pharm Exp Med 10:222–30.
- Gill NS, Garg M, Bansal R, et al. (2009). Evaluation of antioxidant and antiulcer potential of Cucumis sativum L. seed extract in rats. Asian J Clin Nutr 1:131–8.
- Goswani S, Jain S, Santani D. (1997). Antiulcer activity of cromakalim against experimentally induced gastric and duodenal ulcers in rats and guinea pigs. J Pharm Pharmacol 49:195–209.
- Huang D, Ou B, Hampch-Woodill M, et al. (2002). High throughput assay of oxygen radical absorbance capacity (ORAC) using a multichannel liquid handling system coupled with a microplate fluorescence reader in 96-well format. J Agric Food Chem 5:4437–44.
- Ikhiri K, Boureima D, Dan-Kouloudo D. (1992). Chemical screening of medicinal plants used in the traditional pharmacopoeia of Niger. Int J Pharmacogn 30:251–62.
- Itokawa H, Qiao YF, Takeya K. (1990). New arborane type triterpinoids from Rubia cordifolia var. pratensis, and R. onchotrica. Chem Pharm Bull 38:1435–7.
- Kaur S, Sharma K. (2014). Comparative analysis of total phenolic content and anti-oxidant activity of vegetables. Int J Innov Res Dev 3:192–9.
- Khayum MA, Nandakumar K, Gouda TS, et al. (2009). Antiulcer activity of stem extracts of Tinospora malabarica (Lamk.). Pharmacologyonline 1:885–90.
- Kinoshita M, Kume E, Tamaki H. (1995). Roles of prostaglandins, nitric oxide and the capsaicin-sensitive sensory nerves in gastroprotection produced by ecabet sodium. J Pharmacol Exp Ther 275:494–501.
- Kumar A, Singh V, Chaudhary AK. (2011). Gastric antisecretory and antiulcer activities of Cedrus deodara (Roxb.) Loud. In Wistar rats. J Ethnopharmacol 134:294–7.
- Lima ZP, Severi JA, Pellizzon CH, et al. (2006). Can the aqueous decoction of mango flowers be used as an antiulcer agent? J Ethnopharmacol 106:29–37.
- Londonkar RL, Poddar PV. (2009). Studies on activity of various extracts of Mentha arvensis Linn against drug induced gastric ulcer in mammals. World J Gastrointest Oncol 1:82–8.
- Mahmood ND, Mamat SS, Kamisan FH, et al. (2014). Amelioration of paracetamol-induced hepatotoxicity in rat by the administration of methanol extract of Muntingia calabura L. leaves. Biomed Res Int 2014:695678. [Epub ahead of print]. doi:10.1155/2014/695678.
- Makris DP, Kefalas P. (2004). Carob pod polyphenolic antioxidants. Food Tech Biotechnol 42:105–8.
- Mamat SS, Kamarolzaman MFF, Yahya F, et al. (2013). Methanol extract of Melastoma malabathricum leaves exerted antioxidant and liver protective activity in rats. BMC Complement Alternat Med 13:326. [Epub ahead of print]. doi:10.1186/1472-6882-13-326.
- Manosroi A, Jantrawut P, Ogihara E, et al. (2013). Biological activities of phenolic compounds and triterpenoids from the galls of Terminalia chebula. Chem Biodivers 10:1448–63.
- Mohd Joffry S, Yob NJ, Rofiee MS, et al. (2012). Melastoma malabathricum (L.) Smith: A review of its ethnomedicinal, chemical and pharmacological uses. Evid-Based Complement Alternat eCAM 2012:258434. [Epub ahead of print]. doi:10.1155/2012/258434.
- Morimoto Y, Shimohara K, Oshima S, et al. (1991). Effects of the new anti-ulcer agent KB-5492 on experimental gastric mucosal lesions and gastric mucosal defensive factors, as compared to those of teprenone and cimetidine. Jpn J Pharmacol 57:495–505.
- Muralidharan P, Srikanth J. (2009). Antiulcer activity of Morinda citrifolia Linn. fruit extract. J Sci Res 1:345–52.
- Nidavani RV, Mahalakshmi AM, Shalawadi M. (2014). Potent ulcer protective action of anti-inflammatory herbs: A short review. World J Pharm Res 3:2057–66.
- Nwafor PA, Okwuasaba FB, Bind LG. (2000). Antidiarrhoeal and antiulcerogenic effects of methanolic extract of Asparagus pubescens root in rats. J Ethnopharmacol 72:421–7.
- Okoli CO, Ezike AC, Akah PA, et al. (2009). Studies on wound healing and antiulcer activities of extract of aerial parts of Phyllanthus niruri L. (Euphorbiaceae). Am J Pharmacol Toxicol 4:118–26.
- Parmar NS, Desai JK. (1993). A review of the current methodology for the evaluation of gastric and duodenal antiulcer agents. Indian J Pharmacol 25:120–35.
- Pihan G, Regillo C, Szabo S. (1988). Free radicals and lipid peroxidation in ethanol-or aspirin-induced gastric mucosal injury. Digest Dis Sci 32:1395–401.
- Rachchh MA, Jain SM. (2008). Gastroprotective effect of Benincasa hispida fruit extract. Indian J Pharmacol 40:271–5.
- Rang HP, Dale MM, Ritter JM, et al. (2012). Rang and Dale’s Pharmacology, 7th ed. Edinburgh: Churchill Livingstone.
- Rastogi L, Patnaik GK, Dikshit M. (1998). Free radicals and antioxidant status following pylorus ligation induced gastric mucosal injury in rats. Pharmacol Res 38:125–32.
- Rice-Evans CA, Miller NJ, Paganga G. (1996). Structure–antioxidant activity relationships of flavonoids and phenolic acids. Free Radical Biol Med 20:933–56.
- Sachs G, Shin JM, Howden CW. (2006). Review article: The clinical pharmacology of proton pump inhibitors. Aliment Pharmacol Ther 23:2–8.
- Shay H, Komarov SA, Fels SS, et al. (1945). A simple method for the uniform production of gastric ulceration in the rat. Gastroenterology 5:43–61.
- Shiotani A, Graham DY. (2002). Pathogenesis and therapy of gastric and duodenal ulcer disease. Med Clin North Am 86:1447–66.
- Singleton VL, Rossi Jr JA. (1965). Colorimetry of total phenolics with phosphomolybdic-phosphotungstic acid reagents. Am J Enol Viticult 16:144–58.
- Srivastava V, Viswanathaswamy AH, Mohan G. (2010). Determination of the antiulcer properties of sodium cromoglycate in pylorus-ligated albino rats. Indian J Pharmacol 42:185–8.
- Szabo S, Trier JS, Brown A, et al. (1985). Early vascular injury and increased vascular permeability in gastric mucosal injury caused by ethanol in the rat. Gastroenterology 88:228–36.
- Yuan Y, Padol IT, Hunt RH. (2006). Peptic ulcer disease today. Nat Clin Pract Gastroenterol Hepatol 3:80–9.
- Zabidi Z, Wan Zainulddin WN, Mamat SS, et al. (2012). Antiulcer activity of methanol extract of Melastoma malabathricum leaves in rats. Med Princ Pract 21:501–3.
- Zakaria ZA, Balan T, Suppaiah V, et al. (2014). Mechanism(s) of action involved in the gastroprotective activity of Muntingia calabura. J Ethnopharmacol 151:1184–93.
- Zakaria ZA, Hanan Kumar G, Mohd Zaid SNH, et al. (2007). Analgesic and antipyretic actions of Muntingia calabura chloroform extract in animal models. Orient Pharm Exp Med 7:34–40.
- Zakaria ZA, Raden Mohd Nor RNS, Sulaiman MR, et al. (2006). Antinociceptive and anti-inflammatory properties of Melastoma malabathricum leaves chloroform extract in experimental animals. J Pharmacol Toxicol 1:337–45.
- Zam W, Bashour G, Abdelwahed W, et al. (2013). Simple and fast method for the extraction of polyphenol and the separation of proanthocyanidins from carob pods. Sch Acad J Pharm 2:375–80.