Abstract
Context: Ganoderma triterpenoids (GTs) have been recognised as an important bioactive ingredient in Ganoderma Lucidum (Leyss. ex Fr.) Karst. (Polyporaceae), widely used for treating and preventing chronic hepatopathy of various etiologies.
Objective: The objective of this study is to better understand the hepatoprotective effect of GTs and to enhance their use in food supplement pharmaceutical and medical industries.
Materials and methods: HepG2 cells were pretreated in the presence or absence of GTs (50, 100 and 200 μg/ml) for 4 h, then exposed with 60 μmol/L of t-BHP for an additional 4 h. The cell viability was evaluated by MTT method. ALT, AST and LDH production in culture medium and intracellular MDA, GSH and SOD levels were determined. Moreover, the total triterpenoid content and chemical constituents in GTs were detected by ultraviolet spectrophotometry and HPLC/Q-TOF-MS, respectively.
Results: GTs (50, 100 and 200 μg/ml) significantly increased the relative cell viability by 4.66, 7.78 and 13.46%, respectively, and reduced the level of ALT by 11.44%, 33.41% and 51.24%, AST by 10.05%, 15.63% and 33.64%, and LDH by 16.03%, 23.4% and 24.07% in culture medium, respectively. GTs could also remarkably decrease the level of MDA and increase the content of GSH and SOD in HepG2 cells. Furthermore, the total triterpenoid content in GTs was 438 mg GAAEs/g GTs. And 16 triterpenoids in GTs were identified or tentatively characterised.
Discussion and conclusion: Our results showed that GTs had potent cytoprotective effect against oxidative damage induced by t-BHP in HepG2 cells, thus suggesting their potential use as liver protectant.
Introduction
Drug metabolism takes place largely in the liver which is vulnerable to drug-induced injury (Ramachandra Setty et al. Citation2007). However, the overproduction of reactive oxygen species (ROS) during drug metabolisms would result in cellular damage by targeting various macromolecules, such as protein, lipid and nucleic acids (Wu et al. Citation2011), heavily involving in the cause and progression of hepatic damage (Campo et al. Citation2008). Synthetic antioxidants have recently been used in some food applications for ROS scavenging. Nevertheless, they were restricted due to their potential hazards to health, such as liver damage and carcinogenesis (Lobo et al. Citation2010). Therefore, due to the absence of proper medication, the focus has now considerably shifted toward natural ingredients, offering low toxicity and interesting biological properties as alternative therapies for the treatment of hepatic damage (Sharma et al. Citation2011).
Ganoderma lucidum (Leyss. ex Fr.) Karst (Polyporaceae) is a popular medicinal mushroom, which fruiting body is called “Lingzhi” in China and “Reishi” in Japan (Zhang et al. Citation2003). Lingzhi has long been used as a folk remedy for the promotion of health and longevity in China and other oriental countries (Lin and Zhang Citation2004). Also, it is used as an important ingredient in traditional Chinese medicine herbal formulations since ancient time for the prevention and treatment of various types of diseases, such as neurasthenia, insomnia, anorexia, dizziness, chronic hepatitis, hypercholesterolemia, coronary heart disease, hypertension and carcinomas (Choi et al. Citation2014). Due to the potential medicinal value and extensive acceptability, much attention has been focused on the search for bioactive compounds from this mushroom.
Ganoderma triterpenoids (GTs) representing the main bioactive components in this mushroom have attracted considerable attention, being claimed to possess extensive and significant pharmacological properties, such as anti-HIV, anti-hypertensive, hepatoprotective, cytotoxic, anti-allergic, cholesterol-reducing, platelet aggregation-inhibiting and histamine release-inhibiting activities (Lee et al. Citation2010). However, to the best of our knowledge, the hepatoprotective activity of triterpenoids from Ganoderma lucidum in vitro has not been carried out.
In order to extend the understanding of the hepatoprotective effect of GTs, our previous work was conducted to investigate the cytoprotective effect of GTs against the oxidative damage in HepG2 cells induced by tert-butyl hydrogenperoxide (t-BHP) in vitro. The cytotoxicity induced by t-BHP and the maximal noncytotoxic concentration (MNCC) of GTs were measured by using MTT method. The possible protective effect of GTs was evaluated by preincubating cells with and without GTs, followed by treatment with t-BHP. The content of alanine aminotransferase (ALT), aspartate aminotransferase (AST) and lactate dehydrogenase (LDH) in the culture medium was determined, as well as the intracellular level of malondialdehyde (MDA), glutathione (GSH) and superoxide dismutase (SOD). Furthermore, the content of total triterpenoid in GTs was detected by using ultraviolet spectrophotometry, and the chemical constituents in GTs were characterised by using HPLC/Q-TOF-MS.
Materials and methods
Chemicals and reagents
Analytical grade ethanol, chloroform (CHCl3), sodium bicarbonate (NaHCO3), hydrochloric acid (HCl) and phosphoric acid (H3PO4) were purchased from Sinopharm Chemical Reagent, Co., Ltd. (Shanghai, China). Chromatographic grade acetonitrile (ACN) was obtained from Merk (Darmastadt, Germany). Ganoderic acid A with purity more than 98% was obtained from Must Bio-technology Co., Ltd. (Chengdu, China). 3-(4,5-Dimethylthiazolyl-2)-2,5-diphenyl tetrazolium bromide (MTT), phosphate buffer saline (PBS), dimethyl sulfoxide (DMSO) and t-BHP were purchased from Sigma-Aldrich (St. Louis, MO). Fetal bovine serum (FBS), Dulbecco’s Modified Eagle Medium (DMEM), trypsin and penicillin–streptomycin were obtained from Hyclone Laboratories, INC. (Logan, UT). Bicinchoninic acid (BCA) protein assay kit was purchased from Tiangen Biotech CO., Ltd. (Beijing, China). Diagnostic kits for alanine aminotransferase (ALT), aspartate aminotransferase (AST), lactate dehydrogenase (LDH), malondialdehyde (MDA), glutathione (GSH) and superoxide dismutase (SOD) were provided by Jiancheng Institute of Biotechnology (Nanjing, China). All other chemicals were of the highest purity available in China.
Plant material and preparation of GTs
Ganoderma lucidum fruiting bodies were kindly provided by research associate Tiqiang Chen, Institute of Edible Fungi, Fujian Academy of Agricultural Sciences, Fuzhou, China. The voucher specimen was deposited at Academy of Integrative Medicine, Fujian University of Traditional Chinese Medicine for future reference.
GTs were prepared according to the method with minor modification (Weng et al. Citation2007). Briefly, the dried fruiting bodies of Ganoderma lucidum were finely pulverised into ultrafine powder and subsequently extracted with 95% ethanol by ultrasonic circulating extraction. The mixture was filtered and concentrated to dryness with a rotary evaporator. The residue was dissolved in CHCl3. The CHCl3 solvable fraction was further extracted with saturated NaHCO3 for three times, and the alkali solution was collected and acidified with 6 mol/L HCl to a pH value of 2–3. The solution was then extracted with CHCl3 for three times. The CHCl3 fraction was evaporated under reduced pressure to yield GTs.
The total triterpenoid content in GTs
The total triterpenoid content was determined according to the report previously with some modification (Gao et al. Citation2011), by using ultraviolet–visible spectrophotometry (UV-1800 UV/VIS spectrometer, Shimadzu, Tokyo, Japan) with ganoderic acid A as the control and 5% glacial acetic acid–vanillin solution and perchloric acid as the developer. The total triterpenoid content in GTs was calculated as milligrams of ganoderic acid A equivalents (GAAEs) per gram of GTs.
MTT assay for the cytotoxicity of t-BHP and maximal noncytotoxic concentration of GTs
The HepG2 (hepatocellular carcinoma) cell line was procured from American Type Culture Collection (ATCC, Rockville, MD). The cytotoxicity of t-BHP and the maximal noncytotoxic concentration (MNCC) were assessed according to a MTT method as described previously (Yang et al. Citation2013). In brief, exponentially growing HepG2 cells with a density of about 2 × 105 cells/ml were plated into 96-well plates and allowed to adhere for 24 h. The cells were then treated with various concentrations of GTs (50, 100, 200, 400, 800 and 1200 μg/ml) or t-BHP (10, 20, 40, 60, 80, 100 and 150 μmol/L) in separate wells for 4 h. The cells in the control wells received medium containing the same volume of DMSO (0.1%). After the incubation, 20 μl of MTT reagent (5 mg/ml in PBS) was added and cells were incubated for an additional 4 h. The formazan produced by the viable cells was solubilised by the addition of 100 μl DMSO. The suspension was placed on a micro-vibrator for 10–15 min and the absorbance was recorded at 490 nm by the ELISA reader (BioTek ELx800, Winooski, VT). The experiment was performed in triplicate. The relative cell viability was calculated with respect to the untreated control using the following formula:
The cytoprotective effect of GTs
The above-mentioned MTT method was used to evaluate the cytoprotective effect of GTs. Briefly, HepG2 cells were preincubated in the presence or absence of GTs at various concentrations (50, 100 and 200 μg/ml) for 4 h, then the cells were stimulated with t-BHP (60 μmol/L) for an additional 4 h. After the incubation, cell morphological features were observed by using an Olympus inverted phase contrast microscope (200×) (Olympus Corporation, Center Valley, PA). 20 μl of MTT reagent (5 mg/ml in PBS) was added and cells were incubated for an additional 4 h. The formazan produced by the viable cells was solubilised by the addition of 100 μl DMSO. The suspension was placed on a micro-vibrator for 10–15 min and the absorbance was recorded at 490 nm by the ELISA reader (BioTek ELx800, Winooski, VT). The experiment was performed in triplicate. Cell viability was expressed as a percentage relative to the untreated controls.
Determination of ALT, AST and LDH production in culture medium
The activities of ALT, AST and LDH in cell culture medium were measured by commercially available assay kits. Briefly, HepG2 cells (2 × 106 cells/dish) were plated into 100 mm-diameter culture dish. After incubation for 24 h, HepG2 cells were preincubated in the presence or absence of GTs at different concentrations (50, 100 and 200 μg/ml) for 4 h, then the cells were stimulated with t-BHP (60 μmol/L) for a further 4 h. Control cells received 0.1% of DMSO instead of GTs. The cell culture medium was harvested and centrifuged at 4 °C with a speed of 1500 rpm for 5 min to remove floating cell, the supernatant was collected for enzyme activity assay. Results were expressed as unit per liter (U/L).
Determination of MDA, GSH and SOD levels in HepG2 cells
Accumulated intracellular MDA, GSH and SOD levels were measured following the manufacturer's instructions. Briefly, HepG2 cells (2 × 106 cells/dish) were plated into 100 mm diameter culture dish. After incubation for 24 h, HepG2 cells were preincubated in the presence or absence of GTs at different concentrations (50, 100 and 200 μg/ml) for 4 h, then the cells were stimulated with t-BHP (60 μmol/L) for a further 4 h. Control cells received 0.1% of DMSO instead of GTs. After removing the culture medium, HepG2 cells were rinsed with PBS, and dislodged carefully from the culture dish into a 15 ml centrifuge tube by using a cell scraper, and centrifuged at 4 °C with a speed of 1000 rpm for 10 min, the collected cells were resuspended in PBS and sonicated for 15 s in an ice bath. The intracellular MDA, GSH and SOD levels were then determined, and the total protein content was determined by the Bradford method according to the manufacture’s specification. Results were expressed per milligram or gram of the total protein.
HPLC/Q-TOF-MS analysis for GTs
HPLC/Q-TOF-MS analysis was performed on an SHIMADZU LC20AT HPLC instrument (SHIMADZU, Tokyo, Japan) coupled with a BRUKER micrOTOF-QII mass spectrometer (Bruker, Bremen, Germany) equipped with an electrospray (ESI) interface. The HPLC instrument includes a binary pump, an online degasser, and a thermostatically controlled column compartment. Chromatographic separation was carried out at 25 °C on an Inertsil ODS-SP (4.6 mm × 250 mm, 5 μm, GL Sciences Inc., Tokyo, Japan). The chromatographic conditions were as follows: a flow rate of 1.0 ml/min, a sample injection volume of 5 μl, mobile phase A (100% acetonitrile) and mobile phase B (0.1% phosphoric acid–water). The gradient profile was optimised as follows: 0–10 min, 25–30% A; 10–30 min, 30–40% A; 30–60 min, 40–60% A; 60–90 min, 60% A. The peaks were detected with diode array detector at 254 nm.
For the negative ion mode MS detection, the operating parameters were as follows: drying gas (N2) flow rate, 4.0 L/min; drying gas temperature, 180 °C; nebuliser, 2.0 Bar; capillary, +3500 V; end plate offset, −500 V; collision energy, 8.0 V; and transfer time, 80 μs. The sample collision energy was set at 25–55 V. All the acquisition and analysis of data were controlled by Data Analysis Version 4.0 SP1 (Bruker Technologies, Bremen, Germany). Mass spectra were recorded across the range 100–1000 m/z with accurate mass measurement of all mass peaks. Accurate mass measurements of each peak from the total ion chromatogram (TIC) were obtained by means of an automated calibration delivery system using a dual-nebuliser ESI source that introduces a low flow (100 μl/min) of a calibrating solution (sodium formate solution, Bruker Technologies, Bremen, Germany) which contains the internal reference masses at 1518.7125 in a positive ion mode and m/z 112.9856 and 1472.7341 in a negative ion mode. The chemical constituents in GTs were identified or tentatively characterised by comparing MS spectrum with ganoderic acid A and the literature (Yang et al. Citation2007).
Statistical analysis
All data are presented as mean ± standard deviation of three determinations. Statistical comparison was performed via a one-way analysis of variance followed by Dunnett’s test by using SPSS software version 19.0 (SPSS, Chicago, IL). A value of p < 0.05 and p < 0.01 was considered to be statistically significant.
Results
Preparation and total triterpenoid content of GTs
In this study, GTs were extracted from the dry fruiting body of G. lucidum by hot reflux technology with 95% ethanol, and further isolated by using alkaline extraction and acid precipitation method. The yield of GTs was 1.68% based on the dry weight of G. lucidum powder. The total triterpenoid content was 438 GAEs mg/g GTs determined by using ultraviolet–visible spectrophotometry.
Determination for the cytotoxicity of t-BHP and MNCC of GTs
Prior to in vitro hepatoprotective studies, the cytotoxicity of t-BHP and the MNCC of GTs was evaluated by the MTT assay in HepG2 cells. As shown in , the relative cell viability decreased remarkably (p < 0.05), after incubation with increasing concentration of t-BHP (>40 μmol/L) for 4 h. When exposed with 60 μmol/L of t-BHP, the relative cell viability was 68.22%, leading to 31.78% reduction in the relative cell viability (p < 0.01). However, when the concentration used was 80 μmol/L, the relative cell viability was only 25.01% and the inhibitory rate was 74.99% (p < 0.01). Furthermore, as given in , the relative cell viability was 103.27% in HepG2 after treatment with 200 μg/ml of GTs (p > 0.05), indicating that HepG2 cells were well-tolerated to the treatment of GTs (≤200 μg/ml) without showing any cytotoxicity. However, the relative cell viability decreased evidently (p < 0.01) with increasing concentrations (≥400 μg/ml) of GTs. Therefore, the MNCC of GTs was determined as 200 μg/ml, the cytotoxic concentration of t-BHP (60 μmol/L) and non-cytotoxic concentrations of GTs (50, 100 and 200 μg/ml) were selected for the subsequent assessment of whether GTs were able to protect HepG2 cells against t-BHP-induced toxicity.
Figure 1. Cytotoxicity of t-BHP and GTs on cultured human hepatoma HepG2 cells. Relative cell viability was measured by the MTT assay. (A) HepG2 cells were treated with different concentrations of t-BHP (10, 20, 40, 60, 80, 100 and 150 μmol/L) for 4 h. (B) HepG2 cells were treated with various concentrations of GTs (50, 100, 200, 400, 800 and 2000 μg/ml) for 4 h. Relative cell viability was calculated according to the formula. All data are expressed as the means ± SD (error bars) of values from three independent experiments. *p < 0.05 and **p < 0.01 were considered significant versus the control group.
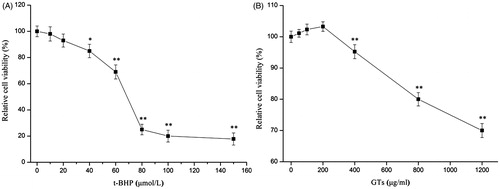
Evaluation for protective effect of GTs
The cytoprotective effect of GTs was evaluated by using the MTT assay and morphological observation. As presented in , it was found that pretreatment with GTs (50, 100, and 200 μg/ml) significantly improved the relative cell viability by 4.66%, 7.78% and 13.46%, respectively, compared with that in the model group (p < 0.05). Moreover, cell morphology was assessed by using an Olympus inverted phasecontrast microscope (200×) (Olympus Corporation, Center Valley, PA). displayed morphological changes of HepG2 cells. Our result showed that the cells presented a fusiform, bipolar shape, adhered and aligned densely, and little cell death was observed in the control (a). And no significant change was observed in MNCC group (b). However, the amount of HepG2 cells diminished dramatically in model group (c), which appeared to be round, contractive, floated and intercellular space increased obviously as compared with the control cells. When HepG2 cells were pretreated with 50 (low-dose group), 100 (medium-dose group) and 200 μg/ml (high-dose group) of GTs for 4 h, the number of cells significantly increased compared with the model group (d–f).
Figure 2. Evaluation of the cytoprotective effect of GTs by MTT assay and morphological observation. (A) HepG2 cells were pretreated with indicated concentrations of GTs for 4 h, and then incubated with or without 60 μmol/L of t-BHP for a further 4 h. Relative cell viability was calculated according to the formula. (B) Morphological alterations to HepG2 cells, pretreated with indicated concentrations of GTs for 4 h, and then incubated with or without 60 μmol/L of t-BHP for a further 4 h. (a and b) Little cell death was observed in the control (Con.) and the MNCC group. (c) The amount of HepG2 cells diminished dramatically in the model group. (d–f) The number of cells significantly increased in a dose-dependent manner, when HepG2 cells were pretreated with 50 (L-d., low-dose group), 100 (M-d., medium-dose group) and 200 μg/ml (H-d., high-dose group) of GTs for 4 h compared with 0 μg/ml (Mod., model group) of GTs. Cell morphology was assessed by using an Olympus inverted phase contrast microscope (200×) (Olympus Corporation, Center Valley, PA). All data are expressed as the means ± SD (error bars) of values from three independent experiments. *p < 0.05 and **p < 0.01 were considered significant versus the model group. #p < 0.05 and ##p < 0.01 were considered significant versus the control group.
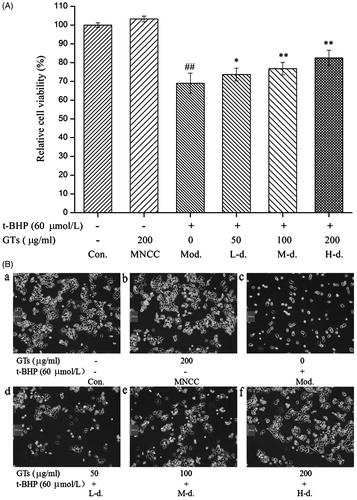
Determination of ALT, AST and LDH production in culture medium
The leakage of ALT, AST and LDH in culture medium was measured in order to further evaluate the hepatoprotective activity of GTs, As presented in ), compared with control cells, t-BHP challenge caused significant increase of ALT, AST and LDH leakage from the cytoplasm into culture medium in the model group (p < 0.01). However, the activities of these three biochemical enzymes did not change obviously in the MNCC group (p > 0.05). Additionally, pretreatment of the cells with various concentrations (50, 100 and 200 μg/ml) of GTs significantly inhibited the leakage of ALT by 11.44, 33.41 and 51.24%, AST by 10.05, 15.63 and 33.64%, and LDH by 16.03, 23.4 and 24.07% in culture medium, respectively (p < 0.05 or p < 0.01).
Figure 3. GTs decreased the up-regulation of ALT, AST, LDH and MDA and increased the down-regulation of GSH and SOD induced by t-BHP in HepG2 cells. HepG2 cells were pretreated with indicated concentrations of GTs for 4 h, and then incubated with or without 60 μmol/L of t-BHP for a further 4 h. The leakage of ALT (A), AST(B) and LDH (C) released by HepG2 cells was measured in the culture supernatant. Hepatocellular (D) GSH, (E) MDA and (F) SOD were assessed from HepG2 cell lysates. All data are expressed as the means ± SD (error bars) of values from three independent experiments. *p <0.05 and **p <0.01 were considered significant versus the model group; #p <0.05 and ##p <0.01 were considered significant versus the control group.
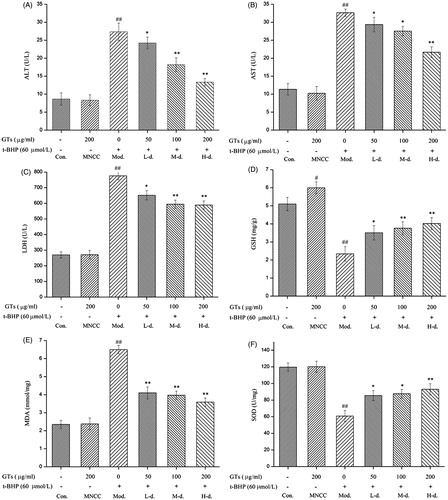
Determination of MDA, GSH and SOD levels in HepG2 cells
The intracellular level of MDA, GSH and SOD were quantified in this study. As shown in ), compared with the control cells, t-BHP treatment resulted in a significant increase in MDA and decrease in GSH, and SOD in HepG2 cells in the model group (p < 0.01), Nevertheless, no obvious change was observed in the MNCC group (p > 0.05). Furthermore, it was found that GTs (50, 100 and 200 μg/ml) pretreatment caused remarkable increase in the intracellular content of SOD by 40.85, 44.37, and 53.10% and GSH by 50, 60.68 and 71.79%, respectively, with a concurrent decrease in the intracellular level of MDA by 36.92, 38.92 and 44.77%, respectively (p < 0.05 or p < 0.01).
Chemical constituents of GTs by HPLC/Q-TOF-MS
To characterise the chemical constituents in GTs, an HPLC/Q-TOF-MS method was developed. All information of MS data indicated retention time, precise molecular mass and provided the MS data that were necessary for the structural identification. The total ion chromatography (TIC) of GTs and ganoderic acid A is shown in . The compound identified, retention time, chemical formula, the accurate/measured mass and ppm error are listed in . By comparing with ganoderic acid A and literature (Yang et al. Citation2007), a total of 16 triterpenoids were identified or tentatively characterised with the mass error of molecular ions within ± 6 ppm.
Table 1. Characterisetion of chemical constituents in GTs by HPLC/Q-TOF-MS.
Discussion
Increasing evidence has indicated that cellular damage mediated by oxidative stress contributed to the initiation and progression of many liver diseases (Videla Citation2009). Many effects have recently been made to develop the complementary and alternative medicines for reducing oxidative stress and improving liver functions. Therapeutically effective agents from natural sources, exemplified by quercetin (Mariee et al. Citation2012), silymarin (Ahmad et al. Citation2013) and oleanolic acid (Lu et al. Citation2014), are particularly attractive for treatments as they have the potential to reduce the risk of drug toxicity (Shivananjappa et al. Citation2013). Ganoderma, a traditional Chinese medicinal mushroom, has been widely used for treating and preventing chronic hepatopathy of various etiologies (Peng et al. Citation2013). GTs represented the main bioactive ingredients in G. lucidum, which has been studied a lot before. It has been demonstrated that GTs possessed powerful protective effects against liver damage induced by CCl4 or d-Gal in mice (Lin et al. Citation2002).
Our current work aimed to investigate the cytoprotective activity of GTs in vitro against the oxidative damage induced by t-BHP in HepG2 cells, aiming to provide a better understanding of the hepatoprotective efficacy. HepG2 cell lines have been proposed as an alternative model to hepatocytes, which are used in various metabolic and drug toxicity studies, possessing the advantage of being available in plenty, easy maintenance, rapid cryopreservation and ability to retain drug metabolic and enzyme activities. Moreover, HepG2 cells possess many morphological and biochemical features of normal hepatocytes. Since it retains many of the phenotypic and genotypic characteristics of liver cells, this cell line has been used in various studies related to medicinal plants for their liver protecting property (Krithika et al. Citation2009). t-BHP, an organic hydroperoxidant, can be metabolised to free radical intermediates, which can subsequently initiate lipid peroxidation, affect cell integrity and form covalent bonds with cellular molecules, resulting in cell oxidative damage characterised by aminotransferase and lactate dehydrogenase (LDH) leakage and formation of malondialdehyde (MDA) in hepatocyte cultures (Wang et al. Citation2000). t-BHP-induced toxicity in HepG2 cells has increasingly been employed as a model to investigate the cytoprotection of natural products (Akanitapichat et al. Citation2010).
In our present study, GTs were extracted by CHCl3 combining with alkali dissolution-acid deposition method. The yield and the total triterpenoids content of GTs were 1.68% and 438 GAEs mg/g GTs, respectively. 60 μmol/L of t-BHP (inhibitory rate of 31.78%) and various concentrations (≤200 μg/ml, MNCC) of GTs were employed to investigate the hepatoprotective effect of GTs from the result of MTT assay (). The possible protective effect of GTs against oxidative damage induced by t-BHP was evaluated by preincubating cells with and without GTs for 4 h, followed by treatment with t-BHP (60 μmol/L) for an additional 4 h. It was found that pretreatment with various concentrations (50, 100 and 200 μg/ml) of GTs could increase cell viability significantly () and enhance cell survival (), when exposed to 60 mmol/L of t-BHP. The result of morphological features observation was in line with that of MTT assay, thus suggesting that GTs could ameliorate the t-BHP-induced oxidative damage in HepG2 cells.
The mechanism involved was ascertained by evaluation of the leakage of enzymes like ALT, AST and LDH, quantification of intracellular MDA and GSH levels and measurement of SOD activity. The liver cells contain high concentration of ALT and AST in cytoplasm. Damage to hepatic cells causes a leakage of liver-specific enzymes in plasma, causing increased serum enzyme levels like ALT and AST in serum. They are recognised as the notable signs of cellular damage and functional integrity of liver cell membrane. And hepatotoxicity in vitro can be directly determined by measuring levels of hepatic transaminase release into the culture medium (Cherubini et al. Citation2005). LHD is localised in the cytoplasm of cells and thus extruded into the serum, when cells are damaged or necrotic. The measurement of total lactate dehydrogenase can be useful when only a specific organ, such as the liver, is known to be involved. LDH is a sensitive intracellular enzyme, which can be used as an indication in liver cell damage (Kim et al. Citation2001). Therefore, the extent of cellular damage was measured in terms of release of leakage enzymes including AST, ALT and LDH. Increased release of these intracellular enzymes indicated membrane damage and instability owing to oxidative injury induced by the hepatoxin. The leakage of ALT, AST and LDH in culture medium was measured in order to demonstrate the hepatoprotective activity of GTs. As shown in ), t-BHP challenge caused significant increase of ALT, AST and LDH leakage from the cytoplasm into culture medium in model group (p < 0.01), which was agreement with the observation (Khanal et al. Citation2009). Pretreatment with various GTs decreased the level of ALT, AST and LDH in culture medium significantly (p < 0.05 or p < 0.01).
Toxicity assays, beyond the use of classical markers including transaminases and lactate dehydrogenase, have commonly focused on the redox state of organs by antioxidant activity enzymes and measurements of defense molecule, macromolecule oxidation (de Freitas et al. Citation2013). SOD plays an important role in scavenging toxic intermediates of incomplete oxidation, which is regarded as one of the most primary antioxidant enzymes in the enzymatic defense mechanism (de Oliveira et al. Citation2015). GSH, an abundant tripeptide in liver, is an important defense molecule against oxidising xenobiotics such as free radicals, peroxides and akylating agents, playing a pivotal role in the detoxification processes. Enhanced levels of GSH protect the cells against a potential oxidative damage (Shivananjappa et al. Citation2013). MDA is one lipid peroxidation product, which has been used as a biomarker of lipid peroxidation for several decades. It appears to offer a facile means of assessing lipid peroxidation in biological materials (Draper and Hadley Citation1990). As given in ), t-BHP treatment led to a significant decrease in the level of SOD and GSH, along with an evident increase in the level of MDA in HepG2 cells in the model group (p < 0.01). The changes induced by t-BHP were alleviated gradually with the increasing concentration of GTs (p < 0.05 or p < 0.01). Taken together, it was suggested that the possible underlying mechanism for the hepatoprotective effect of GTs in vitro was because of its ability to increase antioxidase activity, inhibit lipid peroxidation and maintenance of glutathione in reduced state.
HPLC-MS has become an efficient technique for the rapid simultaneous identification and determination of the multiple compounds in natural products (Cai et al. Citation2013). In our present study, the total ion chromatography (TIC) of GTs and ganoderic acid A were obtained by using HPLC/Q-TOF-MS (). And a total of 16 triterpenoids were identified or tentatively characterised with the mass error of molecular ions within ± 6 ppm () by comparing with ganoderic acid A and literature. However, due to the paucity and cost of reference substances, some structural isomerides, such as ganoderenic acid B, ganoderic acid AM1, ganoderic acid D, lucidenic acid D and ganoderic acid J (accurate mass: 515.3003), were not confirmed and listed in the table. Recently, it was reported that ganoderenic acid A as the potent inhibitor of β-glucuronidase, had a potent hepatoprotective effect on CCl4-induced liver injury (Kim et al. Citation1999). Several triterpenoids, such as ganoderic acid C2, ganoderenic acid B, lucidone B, were shown to exhibit hepatoprotective effect against dl-galactosamine-induced cell damage in HL-7702 cells by the MTT colorimetric assay (Liu et al. Citation2014). Furthermore, ganoderesin B, ganoderol B and lucidone A had significant hepatoprotective activity evidenced from their remarkable inhibitory activities against the increase of ALT and AST levels in HepG2 cells induced by H2O2 (Peng et al. Citation2013). Our result showed that GTs contained various effective triterpenoids, including ganoderenic acid A, ganoderic acid C2 and ganoderenic acid B, suggesting that the hepatoprotective activity of GTs might be attributable to the presence of these active triterpenoids or their potential synergistic effect.
Conclusion
To summarise, GTs offered significant cytoprotection against the oxidative damage induced by t-BHP in HepG2 cells, as revealed by reduction of ALT, AST and LDH levels in the culture medium and remarkable decrease in intracellular MDA content, together with elevation of SOD activity and GSH concentration. Our recent study supported the in vivo study for the hepatoprotective effect of GTs (Lin et al. Citation2002), thus further demonstrating that GTs could serve as a potent hepatoprotective agent in the food supplement, pharmaceutical and medical industries.
Acknowledgements
This work was conducted in Class III Laboratory of Traditional Chinese Medicine on Pharmacognosy of State Administration of Traditional Chinese Medicine of People’s Republic of China and Fujian Provincial Key Laboratory of Integrative Medicine on Geriatrics of Fujian University of Traditional Chinese Medicine. The authors are grateful for the assistance of Da-xin Chen (Academy of Integrative Medicine Biomedical Research Center) in HPLC/Q-TOF-MS identifying the chemical compositions in GTs.
Declaration of interest
The study was financially supported by the Natural Science Foundation of Fujian Province (Grant no. 2015J01688) from Fujian Provincial Department of Science & Technology; as well as Science and Technology Cooperation Project of Municipality and University (Grant no. 2013-G-105) sponsored by Fuzhou Municipal Bureau of Science and Technology.
References
- Ahmad I, Shukla S, Kumar A, Singh BK, Kumar V, Chauhan AK, Singh D, Pandey HP, Singh C. 2013. Biochemical and molecular mechanisms of N-acetyl cysteine and silymarin-mediated protection against maneb- and paraquat-induced hepatotoxicity in rats. Chem Biol Interact. 201:9–18.
- Akanitapichat P, Phraibung K, Nuchklang K, Prompitakkul S. 2010. Antioxidant and hepatoprotective activities of five eggplant varieties. Food Chem Toxicol. 48:3017–3021.
- Cai H, Cao G, Cai B. 2013. Rapid simultaneous identification and determination of the multiple compounds in crude Fructus corni and its processed products by HPLC-MS/MS with multiple reaction monitoring mode. Pharm Biol. 51:273–278.
- Campo GM, Avenoso A, Campo S, Nastasi G, Traina P, D'Ascola A, Rugolo CA, Calatroni A. 2008. The antioxidant activity of chondroitin-4-sulphate, in carbon tetrachloride-induced acute hepatitis in mice, involves NF-kappaB and caspase activation. Br J Pharmacol. 155:945–956.
- Cherubini A, Ruggiero C, Polidori MC, Mecocci P. 2005. Potential markers of oxidative stress in stroke. Free Radic Biol Med. 39:841–852.
- Choi S, Nguyen VT, Tae N, Lee S, Ryoo S, Min BS, Lee JH. 2014. Anti-inflammatory and heme oxygenase-1 inducing activities of lanostane triterpenes isolated from mushroom Ganoderma lucidumin RAW264.7 cells. Toxicol Appl Pharmacol. 280:434–442.
- de Freitas RB, Rovani BT, Boligon AA, de Brum TF, Piana M, da Silva Jesus R, Veloso CF, Kober H, Moresco RN, da Costa Araldi IC, et al. 2013. Hepatotoxicity evaluation of aqueous extract from Scutia buxifolia. Molecules 18:7570–7583.
- de Oliveira TM, de Carvalho RB, da Costa IH, de Oliveira GA, de Souza AA, de Lima SG, de Freitas RM. 2015. Evaluation of p-cymene, a natural antioxidant. Pharm Biol. 53:423–428.
- Draper HH, Hadley M. 1990. Malondialdehyde determination as index of lipid peroxidation. Meth Enzymol. 186:421–431.
- Gao Y, Zhang R, Zhang J, Gao S, Gao W, Zhang H, Wang H, Han B. 2011. Study of the extraction process and in vivo inhibitory effect of ganoderma triterpenes in oral mucosa cancer. Molecules 16:5315–5332.
- Khanal T, Choi JH, Hwang YP, Chung YC, Jeong HG. 2009. Saponins isolated from the root of Platycodon grandiflorum protect against acute ethanol-induced hepatotoxicity in mice. Food Chem Toxicol. 47:530–535.
- Kim DH, Shim SB, Kim NJ, Jang IS. 1999. Beta-Glucuronidase-inhibitory activity and hepatoprotective effect of Ganoderma lucidum. Biol Pharm Bull. 22:162–164.
- Kim KA, Lee WK, Kim JK, Seo MS, Lim Y, Lee KH, Chae G, Lee SH, Chung Y. 2001. Mechanism of refractory ceramic fiber-and rock wool-induced cytotoxicity in alveolar macrophages. Int Arch Occup Environ Health. 74:9–15.
- Krithika R, Mohankumar R, Verma RJ, Shrivastav PS, Mohamad IL, Gunasekaran P, Narasimhan S. 2009. Isolation, characterization and antioxidative effect of phyllanthin against CCl4-induced toxicity in HepG2 cell line. Chem Biol Interact. 181:351–358.
- Lee I, Kim J, Ryoo I, Kim Y, Choo S, Yoo I, Min B, Na M, Hattori M, Bae K. 2010. Lanostane triterpenes from Ganoderma lucidum suppress the adipogenesis in 3T3-L1 cells through down-regulation of SREBP-1c. Bioorg Med Chem Lett. 20:5577–5581.
- Lin ZB, Wang MY, Liu Q, Che QM. 2002. Effects of total triterpenoids extract from Ganoderma lucidum (Curt.: Fr.) P. Karst. (Reishi Mushroom) on experimental liver injury models induced by carbon tetrachloride or d-galactosamine in mice. Int J Med Mushrooms 4:337–342.
- Lin ZB, Zhang HN. 2004. Anti-tumor and immunoregulatory activities of Ganoderma lucidum and its possible mechanisms. Acta Pharmacol Sin. 25:1387–1395.
- Liu LY, Chen H, Liu C, Wang HQ, Kang J, Li Y, Chen RY. 2014. Triterpenoids of Ganoderma theaecolum and their hepatoprotective activities. Fitoterapia 98:254–259.
- Lobo V, Patil A, Phatak A, Chandra N. 2010. Free radicals, antioxidants and functional foods: impact on human health. Pharmacogn Rev. 4:118–126.
- Lu YF, Liu J, Wu KC, Klaassen CD. 2014. Protection against phalloidin-induced liver injury by oleanolic acid involves Nrf2 activation and suppression of Oatp1b2. Toxicol Lett. 232:326–332.
- Mariee AD, Abd-Allah GM, El-Beshbishy HA. 2012. Protective effect of dietary flavonoid quercetin against lipemic-oxidative hepatic injury in hypercholesterolemic rats. Pharm Biol. 50:1019–1025.
- Peng XR, Liu JQ, Han ZH, Yuan XX, Luo HR, Qiu MH. 2013. Protective effects of triterpenoids from Ganoderma resinaceum on H2O2-induced toxicity in HepG2 cells. Food Chem. 141:920–926.
- Ramachandra Setty S, Quereshi AA, Viswanath Swamy AH, Patil T, Prakash T, Prabhu K, Veeran Gouda A. 2007. Hepatoprotective activity of Calotropis procera flowers against paracetamol-induced hepatic injury in rats. Fitoterapia 78:451–454.
- Sharma SK, Arogya SM, Bhaskarmurthy DH, Agarwal A, Velusami CC. 2011. Hepatoprotective activity of the Phyllanthus species on tert-butyl hydroperoxide (t-BH)-induced cytotoxicity in HepG2 cells. Pharmacogn Mag. 7:229–233.
- Shivananjappa MM, Mhasavade D, Joshi MK. 2013. Aqueous extract of Terminalia arjuna attenuates tert-butyl hydroperoxide-induced oxidative stress in HepG2 cell model. Cell Biochem Funct. 31:129–135.
- Videla LA. 2009. Oxidative stress signaling underlying liver disease and hepatoprotective mechanisms. World J Hepatol. 1:72–78.
- Wang CJ, Wang JM, Lin WL, Chu CY, Chou FP, Tseng TH. 2000. Protective effect of Hibiscus anthocyanins against tert-butyl hydroperoxide-induced hepatic toxicity in rats. Food Chem Toxicol. 38:411–416.
- Weng CJ, Chau CF, Chen KD, Chen DH, Yen GC. 2007. The anti-invasive effect of lucidenic acids isolated from a new Ganoderma lucidum strain. Mol Nutr Food Res. 51:1472–1477.
- Wu JG, Ma L, Zhang SY, Zhu ZZ, Zhang H, Qin LP, Wei YJ. 2011. Essential oil from rhizomes of Ligusticum chuanxiong induces apoptosis in hypertrophic scar fibroblasts. Pharm Biol. 49:86–93.
- Yang M, Wang X, Guan S, Xia J, Sun J, Guo H, Guo DA. 2007. Analysis of triterpenoids in Ganoderma lucidum using liquid chromatography coupled with electrospray ionization mass spectrometry. J Am Soc Mass Spectrom. 18:927–939.
- Yang UJ, Park TS, Shim SM. 2013. Protective effect of chlorophyllin and lycopene from water spinach extract on cytotoxicity and oxidative stress induced by heavy metals in human hepatoma cells. J Toxicol Environ Health Part A. 76:1307–1315.
- Zhang HN, He JH, Yuan L, Lin ZB. 2003. In vitro and in vivo protective effect of Ganoderma lucidum polysaccharides on alloxan-induced pancreatic islets damage. Life Sci. 73:2307–2319.