Abstract
Context: A mutant that exhibited increased melanin pigment production was isolated from Aspergillus nidulans fungus. This pigment has aroused biotechnological interest due to its photoprotector and antioxidant properties. In a recent study, we showed that melanin from A. nidulans also inhibits NO and TNF-α production.
Objective: The present study evaluates the mutagenicity and cytotoxicity of melanin extracted from A. nidulans after its exposure to liver S9 enzymes.
Materials and methods: The cytotoxicity of multiple concentrations of melanin (31.2–500 μg/mL) against the McCoy cell line was evaluated using the Neutral Red assay, after incubation for 24 h. Mutagenicity was assessed using the Ames test with the Salmonella typhimurium strains TA98, TA97a, TA100, and TA102 at concentrations ranging from 125 μg/plate to 1 mg/plate after incubation for 48 h.
Results: The cytotoxicity of A. nidulans melanin after incubation with S9 enzymes was less than (CI50 value= 413.4 ± 3.1 μg/mL) that of other toxins, such as cyclophosphamide (CI50 value = 15 ± 1.2 μg/mL), suggesting that even the metabolised pigment does not cause significant damage to cellular components at concentrations up to 100 μg/mL. In addition, melanin did not exhibit mutagenic properties against the TA 97a, TA 98, TA 100, or TA 102 strains of S. typhimurium, as shown by a mutagenic index (MI) <2 in all assays.
Discussion and conclusion: The significance of these results supports the use of melanin as a therapeutic reagent because it possesses low cytotoxicity and mutagenic potential, even when processed through an external metabolising system.
Introduction
A number of organisms, including fungi, produce a dark brown pigment known as melanin, which is present in cell walls associated with chitin and/or is excreted into culture medium as a soluble extracellular polymer (Butler and Day Citation1998; Pagano Citation2015). This pigment appears to be essential in conferring protection against environmental stress. In vitro studies have shown that melanised fungi are more resistant to UV light-induced and oxidant-mediated damage, temperature extremes, hydrolytic enzymes, heavy metal toxicity, and antimicrobial drugs compared with non-melanised fungi (Gómez and Nosanchuk Citation2003; Dadachova et al. Citation2007; Singaravelan et al. Citation2008; Gessler et al. Citation2014).
Melanin is frequently used in medicinal, pharmacological, and cosmetic preparations due to its broad spectrum of biological roles, including its antioxidant, antimicrobial, antiviral, anti-inflammatory, radio-protective, antitumor, and immuno-stimulating activities (Bell and Wheeler Citation1986; Montefiori and Zhou Citation1991; Avramidis et al. Citation1998; Solano Citation2014). Recently, natural melanin was proposed as a new nanocarrier for pH-responsive drug release with potential use in colon- and intestine-targeted drug delivery formulations (Araújo et al. Citation2014).
Melanised fungi can synthesise different types of melanin via the oxidative polymerisation of phenolic or indolic compounds. Some members of the genus Aspergillus produce DHN (1,8-dihydroxynaphthalene)-melanin, while others synthesise DOPA (3,4-dihydroxyphenylalanine)-melanin (Eisenman and Casadevall Citation2012; Pal et al. Citation2014). The biosynthesis of DOPA-melanin by tyrosinase transforms tyrosine into l-Dopa, which is further converted into dopachrome and indole-5,6-quinone and is spontaneously polymerised to produce a dark brown pigment (Mencher and Heim Citation1962).
In a recent study, our group characterised the pigment produced by Aspergillus nidulans mutants as DOPA-melanin based on its physical and chemical properties, UV and IR spectra, and the results obtained with specific inhibitors of DHN- and DOPA-melanin pathways (Gonçalves et al. Citation2012). In other studies, we showed that melanin extracted from A. nidulans had antioxidant activity against biological oxidants, such as HOCl, and led to a marked inhibition of NO and TNF-α production in lipopolysaccharide (LPS)-stimulated macrophages. In addition, this pigment also presented low toxicity in McCoy cells and macrophages (Gonçalves and Pombeiro-Sponchiado Citation2005; Gonçalves et al. Citation2013). These results suggest that A. nidulans melanin may be a promising material for use in cosmetic formulations designed to protect the skin against possible oxidative damage and inflammation.
Substances with known biological activities, such as melanin, are tested for their cytotoxic and mutagenic effects to determine possible adverse effects that may prevent their use as therapeutic agents. The Ames test (Salmonella/Mammalian Microsome Mutagenesis Assay), which can be used to evaluate chemically induced genotoxicity and mutagenicity, is the most accepted and widely used in vitro assay for this purpose. This test assesses the abilities of certain agents to induce gene mutations in different strains of Salmonella typhimurium (Maron and Ames Citation1983; Mortelmans and Zeiger Citation2000). In vitro cytotoxicity assays have been widely used for chemical screening due to the high costs, lengthy experimental times, significant laboratory space requirements, and socioethical concerns associated with testing on laboratory animals. These factors have led to the development of in vitro assays that serve as alternatives to acute in vivo toxicity testing. One in vitro approach has been the use of established cell lines in cytotoxicity assays to determine the acute potencies of chemical test agents (Eisenbrand et al. Citation2002; Ukelis et al. Citation2008).
The toxicity of many chemicals depends on their metabolic activation in the liver. One such example is cyclophosphamide, an anticancer agent that requires metabolic activation by the hepatic cytochrome P-450 system to exert antitumor activity. Varanda et al. (Citation1997) demonstrated that paepalantine (isocoumarin) can easily react via oxidative biotransformation to produce cytotoxic metabolites. Thus, the effects of the biotransformations of potential therapeutics into their metabolites must be evaluated prior to their in vivo administration. In vitro metabolic activation systems have been used to determine whether select compounds and their metabolites exert toxic effects on cellular components. One widely employed system uses the microsomal S9 fraction and associated cofactors to biotransform chemical substances and analyses the toxic potential of their metabolites (Barile Citation1994).
In view of the potential pharmacological applications of A. nidulans melanin, the purpose of the present study was to evaluate the mutagenicity and cytotoxicity of melanin extracted from the fungus A. nidulans in the presence and absence of the hepatic S9 microsomal fraction on McCoy fibroblasts.
Materials and methods
Materials
The McCoy cell line (ATCC CRL-1696b) and Eagle’s medium were obtained from Adolfo Lutz (São Paulo, Brazil). The Salmonella typhimurium tester strains TA98, TA100, TA97a, and TA102 were kindly provided by Dr. B. N. Ames (Berkeley, CA). Fetal bovine serum was obtained from Cultilab (Campinas, São Paulo, Brazil). The S9 fraction was purchased from Molecular Toxicology, Inc. (Annapolis, MD). Neutral red (NR) was obtained from Riedel-de-Haën AG (Seelze, Hannover, Germany). Dulbecco’s modified Eagle’s minimum essential medium (DMEM), RPMI-1640, LPS (Escherichia coli O111:B4), dimethyl sulfoxide, penicillin, streptomycin, l-glutamine, actinomycin D and 2-mercaptoethanol were obtained from Sigma Chemical Co. (St. Louis, MO). All other chemicals were obtained from Sigma Chemical Co. (St. Louis, MO).
Strains and growth conditions
The present study was carried out using the highly melanised mutant MEL1, which was isolated from A. nidulans fungus by Pombeiro (Citation1991). The mutant was grown in Erlenmeyer flasks containing 100 mL of liquid minimal medium as described by Cove (Citation1966), which was supplemented with 55 mM glucose as a carbon source, 70 mM sodium nitrate as a nitrogen source, and 20 μg/mL inositol as a nutritional requirement. The medium was inoculated with 106 conidia/mL and incubated at 37 °C in a rotary shaker at 220 rpm for 72 h. Mycelia were then collected by vacuum filtration, and the melanin present in the culture medium was extracted.
Melanin extraction and purification
Melanin present in the culture medium was extracted using a method previously described by Paim et al. (Citation1990). The culture medium was acidified to pH 1.5 with 6 M hydrochloric acid (HCl) and allowed to stand overnight. The precipitated pigment was then recovered by centrifugation at 4500 × g for 15 min. Melanin purification was performed according to the procedure of Sava et al. (Citation2001) with minor modifications. The precipitate obtained after centrifugation was washed with distilled water and subjected to acid hydrolysis with 7 M HCl and 5% phenol in a nitrogen atmosphere for 7 d to remove carbohydrates and proteins. The sample was then treated with an organic solvent (chloroform, ethyl acetate, and ethanol) to wash away lipids. The residue that was obtained was then dried at room temperature, re-dissolved in 2 M NaOH and centrifuged at 4000 × g for 15 min. The supernatant was precipitated by adding 1 M HCl, washed with distilled water and lyophilised. Approximately 175 μg of pigment was produced per mL of culture medium (Gonçalves et al. Citation2012).
S9 fraction (metabolic activation system)
The S9 fraction was prepared from the livers of Sprague–Dawley rats that were treated with the polychlorinated biphenyl mixture Aroclor 1254. The system consisted of 4% S9 fraction, 1% 0.4 M MgCl2, 1% 1.65 M KCl, 0.5% 1 M d-glucose-6-phosphate disodium, 4% 0.1 M β-nicotinamide adenine dinucleotide phosphate sodium, 50% 0.2 mM phosphate buffer, and 39.5% sterile distilled water. An S9 fraction with a final concentration of 10% (v/v) was prepared in DMEM.
Cytotoxicity assay
The metabolism-mediated cytotoxicity of melanin using the S9 system was evaluated with the Neutral Red (NR) assay. The mouse fibroblast McCoy cell line (ATCC CRL-1696b) was maintained in Eagle’s medium with 7.5% fetal bovine serum (FBS). The cells were harvested from culture by trypsinisation, and aliquots of 200 μL medium (104 cells/mL) were seeded into 96-well tissue culture plates and incubated at 37 °C in a 5% CO2 atmosphere for 24 h. The culture supernatants were removed and replaced with either the medium alone (controls) or with medium containing various concentrations of melanin (ranging from 31.2 to 500 μg/mL) that was previously incubated in the presence of the S9 activation system. The cells were incubated for an additional 24 h under the same conditions. The medium was removed, and the cells were subjected to the NR viability assay as described by Borenfreund and Puerner (Citation1985). The optical density of each well was measured on a microplate reader (Multiskan, Labsystem, MTX Lab Systems, Inc., Vienna, VA) at 540 nm, with 620 nm as a reference wavelength. Cyclophosphamide was used as a reference drug. All experiments were performed at least three times, with triplicate wells for each condition. Linear regression analysis with a 95% confidence limit was used to construct a dose–response curve and to estimate the concentration that reduced the absorbance by 50% (cytotoxic index CI50). The test was also performed with synthetic melanin (Sigma ref.: M-8631) for comparison.
Ames test
Mutagenic activity was evaluated by the Salmonella/microsome assay using the Salmonella typhimurium tester strains TA98, TA100, TA97a, and TA102 with (+ S9) and without (−S9) the presence of an external enzymatic metabolising system by a pre-incubation method (Maron and Ames Citation1983). The strains were grown overnight for 12–14 h in Oxoid Nutrient Broth No. 2. To determine mutagenic activity, four different concentrations of melanin (1 mg/plate, 500, 250, and 125 μg/plate) diluted in DMSO were tested; to accomplish this, either 0.5 mL of 0.2 mM phosphate buffer or 0.5 mL of 4% S9 mixture was added to 0.1 mL of bacterial culture before incubation at 37 °C for 20–30 min. Thereafter, 2 mL of top agar was added, and the mixtures were poured into plates containing minimal agar. The plates were incubated at 37 °C for 48 h, and His + revertant colonies were counted manually. All experiments were performed in triplicate. Toxicity was detected as either a reduction in the number of histidine revertants (His+) or as a thinning of the auxotrophic background (i.e., the background lawn). The data (revertants/plate) were assessed by analysis of variance (ANOVA) followed by linear regression. The mutagenic index (MI) was also calculated for each concentration tested, which represented the average number of revertants per plate with test compound divided by the average number of revertants per plate with negative (solvent) control. A test solution was considered mutagenic when a dose–response relationship was detected and a two-fold increase in the number of mutants (MI ≥ 2) was observed for at least one concentration (Santos et al. Citation2006). The standard mutagens used as positive controls in experiments without the S9 mix were 4-nitrophenylenediamine (10 μg/plate) for TA98 and TA97a, sodium azide (1.25 μg/plate) for TA100 and mitomycin (3 μg/plate) for TA102. In the experiments that included the S9 fraction, 2-anthramine (2.5 μg/plate) was used with TA98, TA97a, and TA100, and 2-aminofluorene (10 μg/plate) was used with TA102. DMSO (75 μL/plate) served as the negative (solvent) control.
Statistical analysis
Statistical analysis of the mutagenicity assay was performed with the software package Salanal 1.0 (U.S. Environmental Protection Agency, Monitoring Systems Laboratory, Las Vegas, NV, from Research Triangle Institute, RTP, NC) using the Bernstein et al. (Citation1982) model. The results of the assays are expressed as the mean ± standard deviation. Differences between groups were determined by ANOVA and were considered significant at p <0.05 and p <0.01. The experimental results of the cytotoxicity assay are expressed as the mean ± standard deviation of triplicate determinations. Differences between groups were also determined by ANOVA and were considered significant at p <0.05.
Results
A concentration–response curve to assess the cytotoxicity of melanin extracted from the MEL1 A. nidulans mutant was constructed in a range of 31.2–500 μg/mL in the presence and absence of an S9 mixture as an external metabolising system (). shows the CI50 values of melanin from the MEL1 mutant, synthetic melanin, and cyclophosphamide (control) in McCoy cells in the presence and absence of the S9 system. Similar dose–response curves were obtained for both melanins, with and without metabolic activation. Opposite results were observed for cyclophosphamide.
Figure 1. Dose–response effect of A. nidulans melanin (A) and synthetic melanin (B) on McCoy cells with (presence of S9) and without (absence of S9) the S9 metabolic activation system. Each point and bar represent the mean ± SD of at least three independent experiments carried out in triplicate.
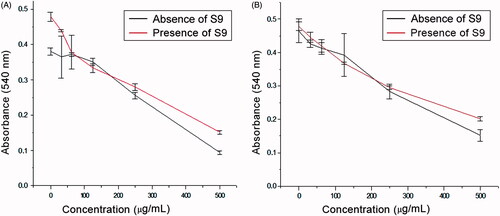
Table 1. Cytotoxic index (CI50) of A. nidulans melanin in the presence and absence of the S9 fraction in the presence of McCoy cells assessed using a neutral red assay.
The CI50 value for the pigment extracted from the MEL1 mutant after its exposure to the S9 fraction was 413.1 ± 3.4 μg/mL. This result differs from that obtained in the absence of the metabolising system, which led to a CI50 value of 373.5 ± 2.4 (Gonçalves et al. Citation2013). The difference between these CI50 values was statistically significant (p <0.05).
Synthetic melanin had CI50 values of 406.5 ± 2.2 μg/mL and 316.1 ± 1.4 μg/mL in the absence and presence of the external metabolising system, respectively (). Statistical analysis also showed that there were significant differences between the results. After activation by S9 (), cyclophosphamide produced a CI50 value of 15 ± 1.2 μg/mL, and viable cells were not observed at a concentration of 22 μg/mL. The exposure of the McCoy cells to cyclophosphamide with and without S9 confirms the efficacy of the hepatic microsomal fraction in the in vitro metabolic activation assay.
The mutagenicity results showed that the number of TA97 strain revertants in the presence of various concentrations of melanin without metabolic activation was not significantly different from the negative control, and the MI values were less than 2.0 (). Conversely, metabolic activation produced a significant difference in the number of revertants for this strain when compared with the negative control at all concentrations except 1 mg/mL; however, the MI values were less than 2.0. There was no linear relationship between the concentration of melanin and the number of revertants, because at the highest concentration tested (1 mg/mL), the number of revertants and the MI decreased compared with those obtained at lower concentrations ().
Table 2. Revertants/plate, standard deviation and MI for the TA97a strain of S. typhimurium after treatment with various doses of melanin with (+S9) and without (−S9) metabolic activation.
For the TA98 and TA100 strains ( and , respectively), the numbers of revertants were not significantly different compared with the negative control, and the MI values were less than 2.0 in both the presence and the absence of the metabolic activation system.
The results from the TA102 strain () indicate that the number of revertants was significantly different relative to the negative control at the lowest concentrations (0.125 and 0.25 μg/mL) in both the presence and the absence of metabolic activation. At higher concentrations (0.5 μg/mL and 1 mg/mL), there was a significant difference relative to the negative control only after metabolic activation. In both cases, the MIs were less than 2.0.
Table 3. Revertants/plate, standard deviation and MI for the TA98 strain of S. typhimurium after treatment with various doses of melanin with (+S9) and without (−S9) metabolic activation.
Table 4. Revertants/plate, standard deviation and MI for the TA100 strain of S. typhimurium after treatment with various doses of melanin with (+S9) and without (−S9) metabolic activation.
Table 5. Revertants/plate, standard deviation and MI for the TA102 strain of S. typhimurium after treatment with various doses of melanin with (+S9) and without (−S9) metabolic activation.
Discussion
Mutagenicity and cytotoxicity studies of natural products have increased due to a greater interest in their therapeutic use in diverse pharmacological fields and because their potential adverse effects are often not well documented in the literature. Various studies have revealed that many compounds isolated from microorganisms have mutagenic effects. Two fungal polyketides, aflatoxin (AF) and sterigmatocystin (ST), are among the most toxic, mutagenic, and carcinogenic natural products known. AFs are only produced by certain Aspergillus parasiticus, Aspergillus flavus, and Aspergillus nomius strains; however, ST is the penultimate intermediate in the AF pathway and is synthesised as an end product by numerous ascomycetes and deuteromycetes, including A. nidulans (Fujii et al. Citation1976; Wogan et al. Citation1992; Anda and Atena Citation2015). Thus, if fungal pigments are to be used as therapeutic agents in biological applications, dermatological systems, and for medical purposes, then the evaluation of their possible mutagenic and cytotoxic effects is necessary.
The Ames test is a widely accepted method of identifying chemical compounds, mixtures, and drugs that are mutagenic; furthermore, this test is highly predictive of in vivo carcinogenicity. Common tester strains include S. typhimurium TA97a, TA98, TA100, and TA102. TA97 and TA98 are used to detect mutagens that cause DNA base frameshifts, and TA100 and TA102 are used to detect mutagens that cause DNA base-pair substitutions (Zeiger et al. Citation1990; Kouvelis et al. Citation2011).
In toxicity and mutagenicity assays, biotransformation is one of the most important factors that can affect the toxic profile of a drug; it can lead to the detoxification and excretion of the drug, as well as its bioactivation. The hepatic microsomal S9 fraction is part of the cytochrome P-450 family and is associated with the endoplasmic reticulum membrane. The S9 fraction is rich in soluble enzymes that possess catalytic abilities against specific substrates. Accordingly, this fraction contains a wide variety of phase I and phase II enzymes (Xiaodong and Lee Citation2007). In vitro studies of metabolism-mediated cytotoxicity and mutagenicity have used cell viability assays that included the test mixture and the hepatic microsomal S9 fraction because the majority of cell lines are currently used in vitro (Brandon et al. Citation2003; Kouvelis et al. Citation2011; Kitagawa et al. Citation2015).
In comparing the activity of melanin in the absence (Gonçalves et al. Citation2013) and the presence of the S9 fraction, it was revealed that melanin extracted from A. nidulans (natural) and synthetic melanin had similar CI50 values, indicating that these compounds have low cytotoxic effects even after biotransformation (). Indeed, we found an increase in the CI50 value in the presence of the S9 fraction, suggesting that biotransformation reduces the toxicity of A. nidulans melanin. These results suggest that melanin may have undergone phase II metabolism, which generally increases the water solubility and decreases the toxicity of substances whose products present higher molecular weights than their corresponding substrates (Testa and Krämer Citation2008). Costa et al. (Citation2015) produced similar results when evaluating synthetic melanin derivatives of l-DOPA that were modified with amino acids; these derivatives did not lead to cytotoxicity in NIH/3T3 fibroblasts and had CI50 values > 500 mg/mL. Other studies have demonstrated that the presence of melanin pigment or melanogenesis decreases the toxicity of chemotherapeutics, such as cyclophosphamide, because melanin is a strong detoxifying agent and reactive oxygen species scavenger (Slominski et al. Citation2009).
The balance between the therapeutic and toxicological effects of a given compound is an extremely important measure of its usefulness as a pharmacological drug. Therefore, determining the mutagenic effect of any drug that is under development is mandatory (Munari et al. Citation2010). Our results demonstrated via the Ames test that the TA97a, TA98, TA100, and TA102 strains were not mutagenic, whether in the presence or absence of the S9 fraction (, respectively). Similar studies using the melanin present in human hair and the TA1535, TA1538, TA98, and TA100 strains showed that the pigment became mutagenic only after treatment with ultraviolet light in the absence of metabolic activation at high concentrations (ranging from 6.8 to 27.5 mg/plate) (Harsanyi et al. Citation1980). These observations may explain the increased incidence of skin cancer in humans due to sunlight exposure, particularly those with blond and red hair. Miranda et al. (Citation1997) also demonstrated that after irradiation with ultraviolet light the melanin synthesis intermediate 5,6-diidroxindol can lead to the formation of cross-linked DNA molecules.
Compounds that are chemically related to melanin have also demonstrated an absence of mutagenicity. In a study by Kitagawa et al. (Citation2015), 5-methoxy-3,4-dehydroxanthomegnin, a naphtho-quinone, produced results similar to those obtained with melanin, with MI values ranging from approximately 1.0–1.6. Additionally, in the presence of the hepatic S9 microsomal fraction, there was no significant change in mutagenicity. Various phenolic compounds are also considered genotoxically safe because they do not have mutagenic effects; furthermore, some of these compounds exhibit antimutagenic effects (Saraç and Sen Citation2014; Yoshida et al. Citation2015). The mutagenic effects of certain indole compounds have been studied by Curvall et al. (Citation1982) using the TA98 strain of S. typhimurium. They found that compounds with methyl substituents at positions 2 and 3 of the indole ring have mutagenic properties at concentrations equal to or greater than 3 mmol/plate.
Thus, the results of the present study demonstrated that at the concentrations used in our biological tests, melanin did not exert cytotoxic effects on the McCoy cell line in the presence of a metabolic activation system. Furthermore, melanin did not have mutagenic properties in the tested S. typhimurium strains. Our findings contribute to a better understanding of the biological effects of melanin and support its future pharmaceutical use.
Declaration of interest
This work was financially supported by Espirito Santo Federal University, Programa de Apoio a Pós-graduação (PROAP-UNESP) and Coordenacão de Aperfeiçoamento Pessoal de Nível Superior (CAPES).
References
- Anda SS, Atena BC. 2015. Mycotoxin effects in agriculture: a review. A&K Rev. 1:1–11.
- Araújo M, Viveiros R, Correia TR, Correia IJ, Bonifácio VD, Casimiro T, Aguiar-Ricardo A. 2014. Natural melanin: a potential pH-responsive drug release device. Int J Pharm. 469:140–145.
- Avramidis N, Kourounakis A, Hadjipetrou L, Senchuk V. 1998. Anti-inflammatory and immunomodulating properties of grape melanin. Inhibitory effects on paw edema and adjuvant induced disease. Arzneimittelforschung 48:764–771.
- Barile FA. 1994. Introduction to in vitro cytotoxicology: mechanisms and methods. Florida: CRC Press.
- Bell AA, Wheeler MH. 1986. Biosynthesis and functions of fungal melanins. Annu Rev Phytopathol. 24:411.
- Bernstein L, Kaldor J, Mccann J, Pike MC. 1982. An empirical approach to thestatistical analysis of mutagenesis data from the Salmonella test. Mutat Res. 97:267–281.
- Borenfreund E, Puerner JA. 1985. Toxicity determined in vitro by morphological alterations and neutral red absorption. Toxicol Lett. 24:119–124.
- Brandon EFA, Raap CD, Meijerman I, Beijnen JH, Schellens JH. 2003. An update on in vitro test methods in human hepatic drug biotransformation research: pros and cons. Toxicol Appl Pharmacol. 189:233–246.
- Butler MJ, Day AW. 1998. Fungal melanins: a review. Can J Microbiol. 44:115.
- Costa TG, Feldhaus MJ, Vilhena FS, Heller M, Micke GA, Oliveira AS, Brighente IMC, Monteiro FBF, Creczynski-PasaTB, Szpoganicz B. 2015. Preparation, characterization, cytotoxicity and antioxidant activity of DOPA melanin modified by amino acids: melanin-like oligomeric aggregates. J Braz Chem Soc. 26:273–281.
- Cove DJ. 1966. The induction and repression of nitrate reductase in the fungus Aspergillus nidulans. Biochim Biophys Acta. 113:51–56.
- Curvall M, Florin I, Jansson T. 1982. Mutagenicity of some indoles and related compounds in the Ames test. Toxicology. 23:1–10.
- Dadachova E, Bryan RA, Huang X, Moadel T, Schweitzer AD, Aisen P, Nosanchuk JD, Casadevall A. 2007. Ionizing radiation changes the electronic properties of melanin and enhances the growth of melanized fungi. PLoS One 2:e457.
- Eisenbrand G, Pool-Zobelb B, Bakerc V, Balls M, Blaauboer BJ, Boobis A, Carere A, Kevekordes S, Lhuguenot JC, Pieters R, et al. 2002. Methods of in vitro toxicology. Food Chem Toxicol. 40:193–236.
- Eisenman HC, Casadevall A. 2012. Synthesis and assembly of fungal melanin. Appl Microbiol Biotechnol. 93:931–940.
- Fujii K, Kurata H, Odashima S, Hatsuda Y. 1976. Tumor induction by a single subcutaneous injection of sterigmatocystin in newborn mice. Cancer Res. 36:1615–1618.
- Gessler NN, Egorova AS, Belozerskaya TA. 2014. Melanin pigments of fungi under extreme environmental conditions (Review). Appl Biochem Microbiol. 50:125–134.
- Gómez B, Nosanchuk JD. 2003. Melanin and fungi. Curr Opin Infect Dis. 16:91–96.
- Gonçalves RCR, Kitagawa RR, Raddi MSG, Carlos IZ, Pombeiro-Sponchiado SR. 2013. Inhibition of nitric oxide and tumour necrosis factor-α production in peritoneal macrophages by Aspergillus nidulans melanin. Biol Pharm Bull. 36:1915–1920.
- Gonçalves RCR, Lisboa HCF, Pombeiro-Sponchiado SR. 2012. Characterization of melanin pigment produced by Aspergillus nidulans. World J Microbiol Biotechnol. 28:1467–1474.
- Gonçalves RCR, Pombeiro-Sponchiado SR. 2005. Antioxidant activity of the melanin pigment extracted from Aspergillus nidulans. Biol Pharm Bull. 28:1129–1131.
- Harsanyi ZP, Post PW, Brinkmann JP, Chedekel MR, Deibel RM. 1980. Mutagenicity of melanin from human red hair. Experientia 36:291–292.
- Kitagawa RR, Vilegas W, Varanda EA, Raddi MSG. 2015. Evaluation of mutagenicity and metabolism-mediated cytotoxicity of the naphthoquinone 5-methoxy-3, 4-dehydroxanthomegnin from Paepalanthus latipes. Rev Bras Farmacogn. 25:16–21.
- Kouvelis VN, Wang C, Skrobek A, Pappas KM, Typas MA, Butt TM. 2011. Assessing cytotoxic and mutagenic effects of secondary metabolites produced by several fungal biological control agents with the AMES assay and the Vitotox® test. Mutat Res Genet Toxicol Environ Mutagen. 722:1–6.
- Maron DM, Ames BN. 1983. Revised methods for the Salmonella mutagenicity test. Mutat Res. 113:173–215.
- Mencher JR, Heim AH. 1962. Melanin biosynthesis by Streptomyces lavendulae. J Gen Microbiol. 28:665–670.
- Miranda M, Ligas C, Amicarelli F, D’Alessandro E, Brisdelli F, Zarivi O, Poma A. 1997. Sister chromatid exchange (SCE) rates in human melanoma cells as an index of mutagenesis. Mutagenesis 12:233–236.
- Montefiori DC, Zhou JY. 1991. Selective antiviral activity of synthetic soluble l-tyrosine and l-dopa melanins against human immunodeficiency virus in vitro. Antiviral Res. 15:11–25.
- Mortelmans K, Zeiger E. 2000. The Ames Salmonella/microsome mutagenicity assay. Mutat Res. 455:29–60.
- Munari CC, Alves JM, Bastos JK, Tavares DC. 2010. Evaluation of the genotoxic and antigenotoxic potential of Baccharis dracunculifolia extract on V79 cells by the comet assay. J Appl Toxicol. 30:22–28.
- Pagano MC. 2015. Fungal pigments: an overview. In: Gupta VK, Sreenivasaprasa S, Mach RL, editors. Fungal bio-molecules: sources, applications and recent developments. New Jersey: John Wiley & Sons. p. 173–179.
- Paim S, Linhares LF, Mangrich AS, Martin JP. 1990. Characterization of fungal melanins and soil humic acids by chemical analysis and infrared spectroscopy. Biol Fert Soils. 10:72–76.
- Pal AK, Devarshi UG, Abhay RV. 2014. DOPA and DHN pathway orchestrate melanin synthesis in Aspergillus species. Med Mycol. 52:10–18.
- Pombeiro SRC. 1991. Control of gene expression of the transport and metabolism of sources of nitrogen in Aspergillus nidulans: Influence of the concentration nitrite, of the pH and of the citrate presence [doctor thesis]. Ribeirão Preto: University of São Paulo.
- Santos FV, Colus IMS, Silva MA, Vilegas W, Varanda EA. 2006. Assessmentof DNA damage induced by extracts and fractions of Strychnos pseudoquina, a Brazilian medicinal plant with antiulcerogenic activity. Food Chem Toxicol.. 44:1585–89.
- Saraç N, Sen B. 2014. Antioxidant, mutagenic, antimutagenic activities, and phenolic compounds of Liquidambar orientalis Mill. var. orientalis. Ind Crops Prod. 53:60–64.
- Sava VM, Galkin BN, Hong MY, Yang PC, Huang GS. 2001. A novel melanin-like pigment derived from black tea leaves with immuno-stimulating activity. Food Res Intern. 34:337–343.
- Singaravelan N, Grishkan I, Beharav A, Wakamatsu K, Ito S, Nevo E. 2008. Adaptive melanin response of the soil fungus Aspergillus niger to UV radiation stress at “Evolution Canyon”, Mount Carmel, Israel, PLoS One. 3:e2993.
- Slominski A, Zbytek B, Slominski R. 2009. Inhibitors of melanogenesis increase toxicity of cyclophosphamide and lymphocytes against melanoma cells. Int J Cancer. 124:1470–1477.
- Solano F. 2014. Melanins: skin pigments and much more-types, structural models, biological functions, and formation routes. New J Sci. 1–28.
- Testa B, Krämer SD. 2008. The biochemistry of drug metabolism – an introduction: part 4. reactions of conjugation and their enzymes. Chem Biodivers. 5:2171–2336.
- Ukelis U, Kramer PJ, Olejniczak K, Mueller SO. 2008. Replacement of in vivo acute oral toxicity studies by in vitro cytotoxicity methods: opportunities, limits and regulatory status. Regul Toxicol Pharmacol. 1:108–118.
- Varanda EA, Raddi MSG, Dias FL, Araújo MC, Gibran SC, Takahashi CS, Vilegas W. 1997. Mutagenic and cytotoxic activity of an isocoumarin (paepalantine) isolated from paepalanthus vellozioides. Teratog Carcinog Mutagen. 17:85–95.
- Wogan GN, Househam KC, Hundt HK. 1992. Aflatoxins as risk factors for hepatocellular carcinoma in humans: aflatoxin exposure and its relationship to kwashiorkor in African children. Cancer Res. 52:2114s–2118s.
- Xiaodong L, Lee J. 2007. The conduct of drug metabolism studies considered good practice (II): in vitro experiments. Curr Drug Metab. 8:822–829.
- Yoshida EH, Ferraz MC, Tribuiani N, Tavares RVS, Cogo JC, Santos MG, Franco LM, Dal-Belo CA, Grandis RA, Resende FA, et al. 2015. Evaluation of the safety of three phenolic compounds from Dipteryx alata Vogel with antiophidian potential. Chin Med. 6:1–12.
- Zeiger E, Haseman JK, Shelby MD, Margolin BH, Tennant RW. 1990. Evaluation of four in vitro genetic toxicity tests for predicting rodent carcinogenicity: confirmation of earlier results with 41 additional chemicals. Environ Mol Mutagen. 16:1–14.