Abstract
Context: Morus nigra L. (Moraceae) has various uses in traditional medicine. However, the effect of M. nigra on cognitive impairment has not been investigated yet.
Objective: The objective of this study is to determine the phenolic acid content and DNA damage protection potential of M. nigra leaf extract and to investigate the extract effect on cognitive impairment and oxidative stress in aging mice.
Materials and methods: Phenolic acid content was determined by quantitative chromatographic analysis. DNA damage protection potential was evaluated on pBR322 plasmid DNA. Thirty-two Balb-C mice were randomly divided into four groups (control, d-galactose, d-galactose + M. nigra 50, and d-galactose + M. nigra 100). Mice were administered d-galactose (100 mg/kg, subcutaneous) and M. nigra (50 or 100 mg/kg, orally) daily for 8 weeks. Behavioral responses were evaluated with Morris water maze. Activities of antioxidant enzymes and levels of malondialdehyde (MDA) were assayed in serum, brain, and liver.
Results: In extract, vanillic (632.093 μg/g) and chlorogenic acids (555.0 μg/g) were determined. The extract between 0.02 and 0.05 mg/mL effectively protected all DNA bands against the hazardous effect of UV and H2O2. Morus nigra significantly improved learning dysfunctions (p < 0.01), increased memory retention (p < 0.01), reduced MDA levels (p < 0.05), and elevated SOD, GPx, and CAT activities (p < 0.05) compared with the d-galactose group.
Discussion and conclusion: These results show that M. nigra has the potential in improving cognitive deficits in mice and that M. nigra may be useful to suppress aging, partially due to its scavenging activity of free radicals and high antioxidant capacity.
Introduction
Aging is a natural phenomenon associated with diverse chronic diseases, including cancer, cardiovascular diseases and neurodegenerative diseases such as Parkinson disease or Alzheimer disease. As aging reduces mental, physical or social activities in human beings, anti-aging has become a major public issue (Bao et al. Citation2014). Accumulated evidence suggests that oxidative stress is an essential source for the process of biological aging, and reactive oxygen species (ROS) and reactive nitrogen species (RNS) play an important role by oxidizing DNA, lipid and protein macromolecules (Calabrese et al. Citation2010; Obrenovich et al. Citation2011; Fransen et al. Citation2013). There is a general reduction in different physiologic functions which lead to age-related diseases during aging in most organs. Brain is the most sensitive organ that gets affected by oxidative damage because of its high metabolic rate, high oxygen need and relatively low antioxidative defence mechanism. Brain aging has an important role in cognitive dysfunction, which is usually related with neurodegenerative diseases (Hélie et al. Citation2012; O'Neill Citation2013). d-Galactose is a reducing sugar which can be metabolized at the normal level. However, at high concentration, an aberrant biochemical mechanism launches and d-galactose changes into galactitol which accumulates in the cell and leads to the generation of ROS (Kumar et al. Citation2011; Wang et al. Citation2012). Long-term administration to d-galactose induces aging-related symptoms similar to those in normal aging span, such as neurodegeneration, significant drops in learning and memory levels, substantial decrease in antioxidant enzyme levels and excessive free radical formation (Li et al. Citation2012; Xian et al. Citation2014).
Morus nigra L. (Moraceae) is known as black mulberry. Almost all parts of this tree are used for pharmacological effects (Naderi et al. Citation2004; Singab et al. Citation2005; Imran et al. Citation2010). Morus nigra leaves have been used to stimulate insulin production for diabetes in Europe (Ode Citation2000) and has been reported to reduce incidence of internal anomalies in offspring from diabetic dams (Volpato et al. Citation2011). In addition, it is reported to have neuroprotective effects against cerebral ischemia (Kang et al. Citation2006), to improve inflammation-related hematological parameters in arthritic rats (Kim and Park Citation2006), to have potential antiobesity and antidiabetic effects in HF diet-induced obesity (Lim et al. Citation2013), and to have free radical scavenger effects (Isabelle et al. Citation2008). Recently, due to its high-bioactive content, M. nigra is believed to be a more healthy fruit among mulberry species (Orhan and Ercisli Citation2010).
Black mulberry contains bioflavonoids that are important natural antioxidants and also contains non-anthocyanin phenolics that are known to have many bioactive functions, including neuroprotective effects, which may be responsible for their medicinal properties (Ercisli and Orhan Citation2007; Shih et al. Citation2010). To the best of our knowledge, the effect of M. nigra on cognitive impairment has not been investigated yet. Therefore, the present study explores the potential role of M. nigra effect in oxidative stress and cognitive impairment in an aging mouse model.
Materials and methods
Chemical reagents and drugs
d-Galactose and the agents used for diagnostic purposes were obtained from Sigma Chemical Co. (St. Louis, MO). Commercial kits used for the determination of superoxide dismutase (SOD), glutathione peroxidase (GPx) and catalase (CAT) were purchased from Cayman Chemical Company, Ann Arbor, MI. The purity of all chemical reagents was at least of analytical grade.
Plant material and preparation of extract
The leaves of M. nigra were collected from southeast region of Turkey (Nizip, Gaziantep) in June 2012. Plant material was scientifically identified by a senior taxonomist, Dr. Erol Dönmez, from Department of Biology, Faculty of Science, Cumhuriyet University, Sivas, Turkey. The collected material was dried in an environment with no direct sunlight and good air flow and grounded by a blender. The air-dried and grounded samples were extracted using a method described by Sokmen et al. (Citation1999). The sample, weighing about 100 g, was extracted in a Soxhlet apparatus with methanol (MeOH) at 60 °C for 6 h. Because of the polar characteristics of the phenolic acids, the extract was further fractionated with chloroform and distilled water. Water sub-fraction was frozen and eventually lyophilized, then kept in the dark at 4 °C until tested.
Determination of phenolic acid levels of the extract
Phenolic acids analysis was performed according to the method described by Ozturk et al. (Citation2008) using an Agilent HPLC series 1200 (Agilent, Waldbronn, Germany) with a slight modification. The separation of gallic (GA), protocathechuic (protoCA), p-hydroxy benzoic (p-hydBA), vanillic (VA), caffeic (CA), chlorogenic (ChA), syringic (SA), p-coumaric (p-COU), ferulic (FA), o-coumaric (o-COU), rosemarinic (RMA), and trans-cinnamic (tr-CIN) acids was performed on an Agilent Zorbax Eclipse XDB-C18 column (Agilent Technologies Inc., Santa Clara, CA) (150 mm, 4.6 mm i.d., 5 μm particle size). The conditions of chromatography were as follows: flow rate of 1 mL/min, sample injection volume of 5 μL, operation temperature of 23 °C, UV detection at 280 nm, and mobile phase A [methanol:water:formic acid (10:88:2, (v/v)] and B [methanol:water: formic acid (90:8:2, (v/v)]. A gradient program was used as the following: 100% A; 0–20 min, changed to 80% A; 25–50 min, to 50% A; 50–54 min, followed by isocratic elution of 50% A; 54–64 min, 0% A; and 64–70 min, 100% A. The results were assessed in terms of the areas of the peaks and their retention times. Quantitation was based on calibration curves built for each of identified compounds in the samples.
Determination of DNA damage protection potential of the extract
DNA damage protection potential of the extract was assessed on pBR322 plasmid DNA (vivantis). In the presence of the extract, plasmid DNA was oxidized with H2O2 + UV treatment and controlled on 1% agarose gels according to a method described by Russo et al. (Citation2000) with some modifications. Briefly, the experiments were carried out in a 10 μL volumetric microfuge tube containing 3 μL pBR322 plasmid DNA (172 ng/μL), 1 μL of 30% H2O2, and 5 μL of extract in the concentrations of 0.005, 0.01, 0.02, 0.04, and 0.05 mg/mL, respectively. The reactions were started by UV irradiation and continued for 5 min on the surface of a UV transilluminator (DNR-IS) with an intensity of 8000 μW/cm2 at 302 nm at room temperature. After irradiation, the reaction mixture (10 μL) along with gel-loading dye (6×) was loaded on the agarose gel for electrophoresis. As a control-untreated pBR322 plasmid DNA was used in each run of the gel electrophoresis along with controls, i.e., only UV or only H2O2-treated plasmids. Gels were stained with EtBr and photographed with the Gel documentation system (DNR-IS, MiniBIS Pro, DNR Inc., Olympia, WA).
Animals and experimental design
Male BALB/c mice, 8-week-old (n = 32) were used in this work. All experiments were conducted at Cumhuriyet University. Eight mice were housed per cage under standardized conditions (12 h light/dark cycle, 24 ± 2 °C, 35 to 60% humidity) and allowed a commercial standard mice diet and water ad libitum. The experiments were performed in accordance with Guide for the Care and Use of Laboratory Animals [National Academy Press publication, Washington, DC, 1996]. The study protocol was approved by the Animal Ethical Committee of Cumhuriyet University (Turkey). After 1 week of adaptation, the mice were randomly divided into four groups (n = 8 in each group): control, d-galactose, d-galactose + M. nigra 50, and d-galactose + M. nigra 100. d-Galactose was dissolved in sterile saline (0.3 mL) and injected subcutaneously (100 mg/kg/d, s.c.) for 60 continuous days (Yi et al. Citation2009; Kumar et al. Citation2011) while mice in the control group were treated with the same volume of sterile saline. d-Galactose + M. nigra group mice were orally administered with lyophilized M. nigra extract at the doses of 50, 100 mg/kg/d, respectively, after injection of d-galactose (100 mg/kg/d, s.c.). At the end of drug administration period, behavioral test and biochemical analysis were performed.
Morris water maze test
Morris water maze was performed according to the methods described previously (Morris et al. Citation1982). The procedure of this test consisted of two learning and memory training trials per day for 4 consecutive days and, after that, a probe trial was performed on day 5 for each mouse. The pool was placed in a well-lighted room with a number of colored clues. The experimental apparatus consisted of a large circular pool with 150 cm diameter, 35 cm deep dimensions filled with water, and kept at 24 ± 1 °C incubation temperature. The pool was divided into four quadrants with equal area. These external clues were used as reference memory and remained unchanged during the experimental period. Acclimation of rats to the experimental environment was set up before the training trials during 2 d. Animals were allowed to swim freely for 1 min on the first day and they were let to swim in the pool divided in four quadrants on the 2nd day of these 2 d. In the training trials, rats were trained to escape from water by finding a hidden circular glass platform (4.5 cm in diameter) placed 1 cm below the water surface at the midpoint of one quadrant. One day before the first trial, each mouse received pre-training for four times. Mouse was first put on the platform for 20 s and then given 30 s free swim and then placed on the platform allowing resting for another 20 s for memorizing the platform location. For each trial, rat was released into water facing the wall of the pool from one of the four starting quadrant points. The time needed to find the hidden platform after being released to the pool was recorded during the training trials on days 1–4. A mouse that found the platform was allowed to stay on the platform for 20 s and then put back to its cage for an interval. If the mouse could not find the platform in 60 s, it was placed on the platform for 20 s and the escape latency (finding the hidden platform) was recorded as 60 s. The capability of learning was assessed with this test. The spatial probe test was performed on day 5 by removing the platform. Each mouse was allowed to swim for 60 s. The time required for the mouse to reach the target quadrant, the time that mouse spent swimming in the target quadrant and the number of crossing over the platform zone were recorded. Consolidated spatial memory was measured with this test.
Biochemical assessment
Biochemical tests were performed 24 h after the last behavioural test. Blood was collected from retro-bulbar venous plexus, then mice were sacrificed and the whole brain tissue and liver were excised immediately in an ice-cold condition, blotted free of blood and tissue fluids, weighed and stored at −80 °C for further analysis.
Assay of MDA level
Lipid peroxidation was monitored in terms of malondialdehyde (MDA) by the method of Ohkawa et al. (Citation1979). MDA level was determined by thiobarbituric acid reactive substances (TBARS) in brain tissue homogenate, based on the reaction between MDA and thiobarbituric acid. Thiobarbituric acid when allowed to react with MDA aerobically formed a coloured complex [MDA–(TBA) 2 complex] which was measured by the spectrophotometer (Shimadzu UV-1700, Shimadzu Corp., Tokyo, Japan) at 532 nm. MDA concentration (measured as TBARS) was calculated as “nmol/mL” for serum and “nmol/mg protein” for brain and liver tissues. Absorbance values were compared with a series of standard solutions of 1, 1, 3, 3-tetra ethoxy propane (TEP).
Measurement of SOD activity
Superoxide dismutase was determined using commercially available standard enzymatic kit (Cayman Chemical Company, Ann Arbor, MI) which utilizes a tetrazolium salt for the detection of superoxide radicals generated by xanthine oxidase and hypoxanthine. The SOD assay measures all three types of SOD (CU/Zn, Mn and Fe SOD). One unit of SOD activity is defined as the amount of enzyme needed to exhibit 50% dismutation of the superoxide radical. The absorbance was read at 440–460 nm using a plate reader (Thermo, Multiskan GO Microplate, Waltham, MA). The SOD activity was expressed as “U/mL” for serum and “U/mg protein” for brain and liver tissues.
Measurement of GSH-px activity
Glutathione peroxidase (GPx) activity was measured using a commercially available kit (Cayman Chemical Company, Ann Arbor, MI). Levels of GPx activity were expressed as “nmol/min/mL” for serum and “nmol/min/mg protein” for brain and liver tissues. The measurement of GPx activity is based on the principle of a coupled reaction with glutathione reductase (GR). The oxidized glutathione (GSSG) formed after the reduction of hydroperoxide by GPx is recycled to its reduced state by GR in the presence of NADPH is accompanied by a decrease in absorbance at 340 nm. One unit of GPx activity is defined as the amount of enzyme that catalyzes the oxidation of 1 nmol of NADPH per minute at 25 °C. Absorbance was read once every minute at 340 nm using a plate reader to obtain at least 5 time points.
Measurement of CAT activity
Catalase (CAT) activity was measured by using a commercially available kit (Cayman Chemical Company, Ann Arbor, MI). The measurement of CAT activity is based on the reaction of the enzyme with methanol in the presence of an optimal concentration of H2O2. The formaldehyde produced is measured colorimetrically with 4-amino-3-hydrazino-5-mercapto-1,2,4-triazole (purpald) as the chromogen. Purpald forms a bicyclic heterocycle with aldehydes, which after oxidation changes from colourless to a purple colour. One unit of CAT activity is defined as the amount of enzyme that will cause the formation of 1.0 nmol of formaldehyde per minute at 25 °C. The absorbance was read at 540 nm. Levels of CAT activity were expressed as “nmol/min/mL” for serum and “nmol/min/mg protein” for brain and liver tissues.
Estimation of total protein content
The total protein content was estimated by the method of Bradford (Citation1976). Bovine serum albumin was used as the standard.
Histopathological evaluation
For neuropathological observation, the brains were fixed in 4% paraformaldhyde in PBS (pH 7.4), embedded into paraffin and serially cut for 5 μm thick coronal sections through the dorsal hippocampus, and stained with hematoxylin and eosin (HE). Tissue sections were examined under light microscope by taking photomicrographs (Nikon Eclipse 80, Nikon Inc., Melville, NY).
Statistical analyses
Data were analysed using the SPSS for Windows, Version 15 (SPSS Inc., Chicago, IL). The results are expressed as means ± standard deviation (SD). The data obtained from the Morris water maze test training trials on days 1–4 were analyzed using two-way ANOVA followed by the Tukey–Kramer multiple comparison tests as post hoc test with repeated measures and the factors were groups and training days. The other data were analysed with one-way ANOVA followed by the Newman–Keuls or the Tukey–Kramer multiple comparison tests as post hoc test. Value of p < 0.05 or p < 0.01 was regarded as significant.
Results
Phenolic acid levels of the extract
Amounts of gallic, protocatechuic, p-hydroxybenzoic, vanillic, caffeic, chlorogenic, syringic, p-coumaric, ferulic, o-coumaric, rosmarinic, and trans-cinnamic acids were quantified in polar methanol extracts of M. nigra. The extracts were further fractionated with equal amounts of chloroform and distilled water in order to eliminate non-polar phytochemicals from the target extract. In the water sub-fraction, the polar phytochemicals were brought together and stored at 4 °C until tested. In M. nigra extract, chlorogenic and vanillic acids were determined (). In M. nigra extract, with respect to the results obtained from the quantitative chromatographic analysis, the major compound was vanillic acid (632.093 μg/g). This was closely followed by chlorogenic acid (555.0 μg/g) ().
DNA damage protection potential of extract
The electrophoretic pattern of DNA after UV-photolysis of H2O2 in the absence and the presence of the aqueous extract of M. nigra is shown in . DNA derived from pBR322 plasmid showed two bands on agarose gel electrophoresis (– lane 1) the faster moving band corresponded to the native form of supercoiled circular DNA (scDNA) and the slower moving band was the open circular form (ocDNA). The UV irradiation of DNA in the presence of H2O2 (column 3) resulted in the cleavage of ocDNA to a faint linear DNA and smears on the agarose gel, indicating that the OH· generated from UV-photolysis of H2O2 produced DNA strand scission.
Figure 2. Electrophoretic pattern of pBR322 plasmid DNA after treatment with UV and H2O2 in the presence of Morus nigra aqueous extract. Addition of 0.02, 0.04, and 0.05 mg/mL M. nigra to the reaction mixture conferred the significant protection to the damage of all DNA bands; lanes 7, 8, and 9. Lane 1: plasmid DNA (3 μL) + dH2O (6 μL), lane 2: plasmid DNA (3 μL) + dH2O (6 μL) + UV, lane 3: plasmid DNA (3 μL) + dH2O (6 μL) + UV + H2O2 (1 μL), lane 4: plasmid DNA (3 μL) + dH2O (6 μL) + H2O2 (1 μL), lane 5: plasmid DNA (3 μL) + aqueous extract (0.005 mg/mL) (5 μL) + + UV + H2O2 (1 μL), lane 6: plasmid DNA (3 μL) + aqueous extract (0.01 mg/mL) (5 μL) + + UV + H2O2 (1 μL), lane 7: plasmid DNA (3 μL) + aqueous extract (0.02 mg/mL) (5 μL) + + UV + H2O2 (1 μL), lane 8: plasmid DNA (3 μL) + aqueous extract (0.04 mg/mL) (5 μL) + + UV + H2O2 (1 μL), lane 9: plasmid DNA (3 μL) + aqueous extract (0.05 mg/mL) (5 μL) + + UV + H2O2 (1 μL).
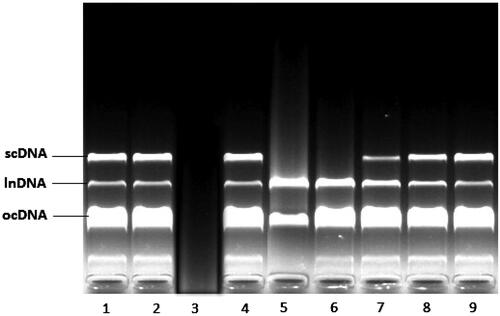
(lanes 5–9) shows the DNA damage protection potential of the aqueous extract of M. nigra. The addition of 0.02, 0.04, and 0.05 mg/mL M. nigra to the reaction mixture provided significant protection against the damage of all DNA bands (, lanes 7–9).
Effect of M. nigra on cognitive impairment induced by d-galactose
For investigating the effect of M. nigra on d-galactose-induced cognitive impairment, we preformed the Morris water maze test which is a sensitive test used for the determination of the impairment of spatial learning and memory (Kumar et al. Citation2011). Two-way repeated measures of ANOVA (day × group) showed that there was a significant difference in mean latency between the groups (F = 13.54, p = 0.001, p < 0.01). There was also a significant difference in mean latency between days (F = 56.73, p = 0.001, p < 0.01). The d-galactose model mice markedly needed more time to find the hidden platform than the control group mice in training days (day 2, p < 0.01; day 3, p < 0.01; day 4, p < 0.01). This result demonstrated that d-galactose model mice had significant cognitive impairment. Morus nigra 50 and 100 mg/kg/d-treated mice shortened the time for escape latency significantly when compared with d-galactose model mice from the second day (p < 0.01, p < 0.01, and p < 0.01) (). These results indicate that both group and day have significant effect on the escape latency and all group rats improved over the training trails. There was a significant interaction between day and group factors in escape latency. These results show that M. nigra prevented d-galactose-induced learning dysfunction in mice.
Figure 3. Effect of M. nigra on the escape latency of d-galactose-treated mice in the Morris water maze test. Escape latencies to find a hidden platform in the water maze during 4 consecutive days training. d-Galactose was dissolved in sterile saline (0.3 mL) and injected subcutaneously (100 mg/kg/d, s.c.) for 60 continuous days and control group were treated with the same volume of sterile saline. The d-galactose + M. nigra group mice were orally administered with lyophilized M. nigra extract at the doses of 50 and 100 mg/kg/d respectively after injection of d-galactose (100 mg/kg/d, s.c.). Data are expressed as mean ± S.D. (n = 8 in each group). **p <0.01 compared with the control group; ##p <0.01 to the d-galactose group.

Three measures were obtained for probe test performance on day 5. For mice in the control and M. nigra 50 and 100 mg/kg groups, the time spent to find the target quadrant was shorter than those in the d-galactose group (p < 0.01 versus the control group; p < 0.01 versus the M. nigra 50 group; p < 0.01 versus the M. nigra 100 group) (). Mice in the control, M. nigra 50 and 100 mg/kg groups spent longer time swimming in the target quadrant than the mice in the d-galactose group (p < 0.01 versus the control group; p < 0.01 versus the M. nigra 50 group; p < 0.01 versus the M. nigra 100 group) (). Again correlative results were obtained for the number of times the mice crossed the platform location. Mice treated with d-galactose crossed the platform location less frequently than mice in the control (p < 0.01) and M. nigra 50 (p < 0.05) and 100 mg/kg groups (p < 0.01) (). These results showed that M. nigra treatment significantly elevated memory retention compared with d-galactose-treated mice.
Figure 4. Effect of M. nigra on behavior of d-galactose-treated mice in the Morris water maze test. d-Galactose was dissolved in sterile saline (0.3 mL) and injected subcutaneously (100 mg/kg/d, s.c.) for 60 continuous days and the control group was treated with the same volume of sterile saline. d-galactose + M. nigra group mice were orally administered with lyophilized M. nigra extract at the doses of 50 and 100 mg/kg/d respectively after injection of d-galactose (100 mg/kg/d, s.c.). Data are expressed as mean ± S.D. (n = 8 in each group). (A) Comparison of the time required to reach the target quadrant on day 5, (B) comparison of the time spent in the target quadrant on day 5, (C) comparison of numbers of crossing over platform site on day 5. **p <0.01 compared with the control group; ##p <0.01, #p <0.05 compared with the d-galactose group.
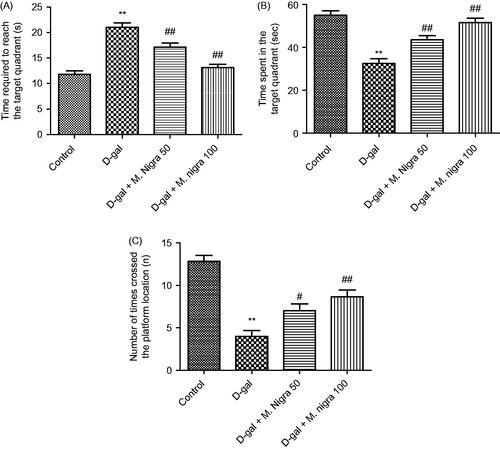
Effect of M. nigra on the levels of MDA
There was a significant increase in MDA levels, a well-known indicator of oxidative damage in mice given d-galactose in all serum (3.43 ± 0.82 nmol/mL), brain (6.08 ± 0.65 nmol/mg protein), and liver (4.50 ± 0.97 nmol/mg protein) compared with the control group (1.53 ± 0.49 nmol/mL, 3.55 ± 0.72 nmol/mg protein, and 2.47 ± 0.69 nmol/mg protein) (p < 0.01) (). Treating mice with M. nigra depressed MDA level augmentation in serum, brain and liver of d-galactose-treated mice significantly (p < 0.01 or p < 0.05) ().
Effect of M. nigra on SOD activity
SOD activities in serum (10.16 ± 2.04 U/mL), brain (8.50 ± 1.05 U/mg protein), and liver (17.67 ± 3.67 U/mg protein) of d-galactose-treated mice were reduced remarkably (p < 0.01, p < 0.01, and p < 0.05) when compared with the control group (15.33 ± 2.42 U/mL, 13.5 ± 2.07 U/mg protein and 30.17 ± 7.68 U/mg protein). Morus nigra treatment significantly increased SOD activity in serum, brain and liver of M. nigra 50 mg/kg-treated groups (14.17 ± 2.40 U/mL, 12.00 ± 2.61 U/mg protein and 28.67 ± 7.37 U/mg protein) (p < 0.05) and M. nigra 100 mg/kg-treated groups (14.33 ± 2.58 U/mL, 12.17 ± 2.32 U/mg protein and 29.67 ± 6.74 U/mg protein) (p < 0.05) compared with the d-galactose-treated group ().
Figure 6. Effect of M. nigra on the activities of SOD, GSH-Px, and CAT in serum, brain, and liver tissue of d-galactose-treated mice. Data are expressed as mean ± S.D. (n = 8 in each group). **p <0.01, *p <0.05 compared with the control group; ##p <0.01, #p <0.05 compared with the d-galactose group.
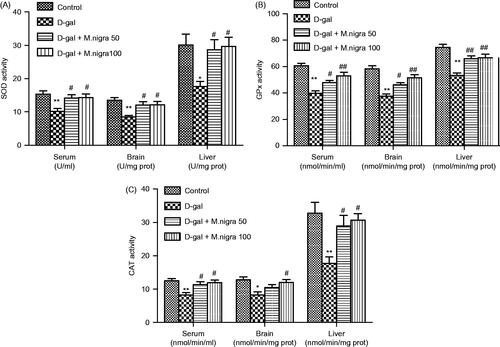
Effect of M. nigra on GPx activity
GPx activities in serum (39.67 ± 4.80 nmol/min/mL), brain (37.67 ± 3.93 nmol/min/mg protein) and liver (55.00 ± 4.20 nmol/min/mg protein) of d-galactose-treated mice were decreased significantly (p < 0.01) compared with the control group (60.67 ± 4.84 nmol/min/mL, 58.33 ± 5.75 nmol/min/mg protein and 74.67 ± 5.47 nmol/min/mg protein). Morus nigra treatment increased the GPx activity markedly in serum, brain and liver of M. nigra 50 mg/kg-treated group (48.17 ± 4.17 nmol/min/mL, 46.17 ± 4.02 nmol/min/mg protein, and 66.17 ± 4.88 nmol/min/mg protein) (p < 0.05, p < 0.05, and p < 0.01) and M. nigra 100 mg/kg-treated group (53.00 ± 6.48 nmol/min/mL, 51.5 ± 6.09 nmol/min/mg protein, and 67.00 ± 6.69 nmol/min/mg protein) (p < 0.01) compared with the d-galactose-treated group ().
Effect of M. nigra on CAT activity
The results showed that CAT activities in serum (8.31 ± 1.72 nmol/min/mL), brain (8.21 ± 2.38 nmol/min/mg protein) and liver (17.76 ± 4.63 nmol/min/mg protein) of d-galactose-treated mice were reduced markedly (p < 0.01) compared with the control group (12.38 ± 1.42 nmol/min/mL, 12.78 ± 2.10 nmol/min/mg protein, and 32.79 ± 7.92 nmol/min/mg protein). Morus nigra treatment increased significantly the GPx activity in serum, brain and liver of M. nigra 50 mg/kg-treated groups (11.37 ± 2.13 nmol/min/mL, 10.51 ± 2.11 nmol/min/mg protein and 28.95 ± 7.68 nmol/min/mg protein) (p < 0.05, non-significant and p < 0.05) and M. nigra 100 mg/kg-treated groups (11.96 ± 2.00 nmol/min/mL, 11.97 ± 2.16 nmol/min/mg protein, and 30.64 ± 4.83 nmol/min/mg protein) (p < 0.05) compared with the d-galactose-treated group ().
Histopathological changes
The histopathological architecture of the hippocampus in mice is shown in . The results indicated that there were typical neuropathological changes in hippocampus of mice induced by d-galactose. Widespread damage was evident in mice treated with d-galactose (). The neurons showed a densely stained shrunken appearance, neurofibrillary degeneration, or had disappeared. However, consecutive administration of M. nigra significantly attenuated these neuropathological changes and the majority of the neurons were rescued in mice treated with M. nigra (). The neuronal cells recovered with prolonged neurofibrillary in their characteristic shapes. These results suggested that the behavioral deficits induced by d-galactose may associate, in a large part, with structural abnormalities in hippocampus formation.
Figure 7. Protective effects of M. nigra against d-galactose induced cell loss in the hippocampus of mice revealed by hematoxylin and eosin (H&E, ×100) staining. (A) Control group: the neurons are full and arranged tightly, the nuclei are light stained; (B) d-galactose group: focal disappearance of neurons and red neurons, as the arrows pointed neurodegeneration was evident (neurons are shrunken, the nuclei are side moved and dark stained); (C) d-galactose + M. nigra 50 group: one red neuron observed; neurodegeneration reduced as the arrow marked; (D) d-galactose + M. nigra 100 group: one red neuron observed the neurons normalize, the nuclei are light stained and arranged tightly and neurodegeneration was attenuated significantly.
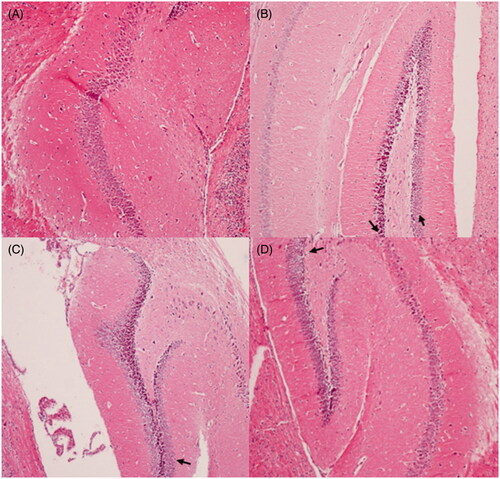
Discussion
The present study demonstrated that M. nigra leaf extract improved cognitive impairment and neurodegeneration induced by long-term application of d-galactose in mice, and this effect may be likely related to enhancement of activities of antioxidant enzymes and scavenging free radicals.
Aging and particularly brain aging is a risk factor for neurodegenerative diseases, such as Alzheimer’s disease and Parkinson’s disease. Oxidative stress and ROS are proposed to be major biochemical manifestations of the aging process and accumulating information suggests that ROS may have an important role for the development of age-related neuronal impairments in brain (Campos et al. Citation2014). As many anti-aging herbs reduce free radical formation and have antioxidant components, using these herbs may positively influence the biological process of aging.
Flavonoids are found in fruits, vegetables, nuts and certain beverages such as tea and are naturally occurring polyphenolic compounds that are good candidates for antioxidant therapy. Besides flavonoids, phenolic acids such as caffeic acid, chlorogenic acid and vanillic acid are also widely found in plant-based foods and seeds and they work as significant radical scavengers (Kancheva and Kasaikina Citation2013). The relation between the structures of phenolic acids and their antioxidant activities has been determined. The number and the position of hydroxyl groups that bound to the aromatic ring, the binding site, and the type of substituents affect the antioxidant activity (Sroka and Cisowski Citation2003; Göçer and Gülçin Citation2011). There are two groups of naturally occurring phenolic acids: hydroxycinnamic acids (caffeic acid, p-coumaric acid, rosmarinic acid, ferulic acid, sinapic acid, and chlorogenic acid) and hydroxybenzoic acids (gallic acid, p-hydrobenzoic acid, vanillic acid, syringic acid, and protocatechuic acid). It has been found that hydroxycinnamic acids have significantly higher antioxidant activity than hydroxybenzoic acids. It has been shown that the introduction of a second hydroxyl group in the ortho- or para-position increases the antioxidant activity and the effect of monophenols increase by one or two methoxy substituents (Gülçin Citation2007). The antioxidant activities of phenolic acids are maintained by inhibiting the formation of ROS or by scavenging them. Thus, phenolic acids may play beneficial role in the prevention of diseases caused due to oxidative stress. The beneficial health effects of phenolics may relate to interactions with signaling cascades including cytokines, key enzymes and transcripton factors.
In the present study, in methanol extracts of M. nigra, amounts of gallic, protocatechuic, p-hydroxybenzoic, vanillic, caffeic, chlorogenic, syringic, p-coumaric, ferulic, o-coumaric, rosmarinic and trans-cinnamic acids were investigated. Vanillic (632 093 μg/g) and chlorogenic acids (555.0 μg/g) were determined as the major compounds in the extract. Chlorogenic acid (3-O-caffeoylquinic acid), which is known to have various biological properties, is a major compound in coffee seeds and is also present in tobacco leaves, fruits, and vegetables. A recent study on chlorogenic acid suggested that its anti-amnestic effect is linked to inhibition of MDA and acetylcholinesterase (Kwon et al. Citation2010). It has been reported that chlorogenic acid reduces brain damage and oedema because of its free radical scavenging effect and its metalloproteinase-2 and 9 inhibition activities (Lee et al. Citation2012). Chlorogenic acid has been found effective at protecting PC12 cells against methylmercury-induced damage by suppressing the generation of ROS, decreasing GPx activity, decreasing GSH, and attenuating caspase-3 activation. These results suggest that chlorogenic acid may apply neuroprotective effects through its antioxidant actions (Li et al. Citation2008). Recent researches on chlorogenic acid suggest that it inhibits DNA damage and lipid peroxidation by increasing the resistance of low-density lipoprotein (Wan et al. Citation2013) and prevents carcinogenesis by reducing DNA damage (Rakshit et al. Citation2010; Ooi et al. Citation2011). It has also been reported to have analgesic (Hara et al. Citation2014), anti- inflammatory (Hwang et al. Citation2014), antidiabetic (Stefanello et al. Citation2014), and antibacterial effects (Ozçelik et al. Citation2011). Vanillic acid (4-hydroxy-3-methoxybenzoic acid) is a benzoic acid derivative found in plant and fruits that is being used as a preservative and antiseptic agent in cosmetic, food, and pharmaceutical industries. Vanillic acid also possess many pharmacological functions such as antihypertensive (Kumar et al. Citation2014), antioxidant (Stanely Mainzen Prince et al. Citation2011), and anticancer effects (Chou et al. Citation2010). Vanillic acid has significant antioxidant activity due to the presence of the carboxyl group (Chou et al. Citation2010). It has also been reported that vanillic acid ameliorates spatial learning and memory retention by preventing oxidative stress (Singh et al. Citation2015).
In the present study, DNA damage protection potential of M. nigra extract was also investigated. The lowest two extract concentrations (0.005 and 0.01 mg/mL) protected major bands named as the open circular DNA (ocDNA) and linearized DNA (InDNA) bands, while the application of 0.02, 0.04, and 0.05 mg/mL extract showed protective effect on all DNA bands. These results demonstrate that M. nigra extract has DNA damage protection potential at a significant level.
Long-term d-galactose injection induces changes that resemble natural aging in experimental animals, such as cognitive dysfunction, oxidative damage, and cholinergic dysfunction (Li et al. Citation2012; Xian et al. Citation2014). In this study we observed that, administration of d-galactose to mice caused cognitive deficit and showed deterioration of learning and memory capacity when Morris water maze was applied. This finding is in conformity with earlier studies reporting cognitive impairment by d-galactose administration in rodents (Kumar et al. Citation2011; Yang et al. Citation2013; Xian et al. Citation2014). d-Galactose-aging mice spent longer time to find the hidden platform during the training trials in the Morris water maze test. This shows impairment of memory. Morus nigra-attenuated d-galactose induced cognitive impairment as detected by decreased latency to reach the hidden platform. Further, we observed that M. nigra significantly raised the time spent in the target quadrant and the number of crossings over the platform location. The present study shows that M. nigra is able to reverse d-galactose-induced learning dysfunction in mice and also increases memory retention compared to d-galactose-treated mice, suggesting its potential neuroprotective role.
Many hypotheses have been introduced to explain the mechanism of the action of d-galactose in aging. One of the mechanisms that has been put forward is that, long-term administration of d-galactose enhances the formation of free radicals which attack lipids and proteins, produces several byproducts and also leads to decrement of antioxidant enzyme activities (Kumar et al. Citation2011; Li et al. Citation2012). According to the free radical theory of aging, free radicals play role in mediating accelerating aging and neurodegeneration (Harman Citation1956). Endogenous antioxidant enzymes such as CAT, SOD, and GSH-Px have an important part to scavenge free radicals or prevent their formation. SOD catalyzes the superoxide anion to hydrogen peroxide and oxygen, and next as electron donor GSH-Px and/or CAT catalyse. These antioxidant enzymes are substantial in fixing memory and learning deficits because there is a strong relation between oxidative stress and cognitive dysfunction (Obrenovich et al. Citation2011; Campos et al. Citation2014). The functions of liver, which is an important organ in the detoxification system, diminish due to structure atrophy in aging. Therefore, we detected the enzyme activities and MDA level not only in brain tissue but also in liver tissue and serum. Declined levels of SOD, GSH-Px, and CAT were observed in d-galactose-induced aging mice in serum, brain and liver tissues. Morus nigra improved the antioxidant activity by elevating the activities of SOD, GSH-Px, and CAT significantly in serum, brain, and liver tissues. The increased activities of these enzymes provided reversing d-galactose-induced cognitive deficits produced from oxidative damage by being effective in scavenging free radicals and their products.
MDA, a by-product of lipid peroxidation, is an important indicator of membrane damage and is used as a biomarker of oxidative stress. In agreement with previous studies on serum, brain and liver tissue of d-galactose-treated mice, there was a significant elevation in MDA levels (Zhang et al. Citation2008; Wang et al. Citation2012). Morus nigra significantly reduced the MDA level induced by d-galactose and it ameliorated the oxidative stress in serum, brain and liver tissues induced by long-term d-galactose exposure. The elevated activities of SOD, GSH-Px and CAT observed in M. nigra-fed mice could be at least partly through its inhibitory effect on lipid peroxidation. Morus nigra may improve learning and memory functions in d-galactose-treated rats by reducing oxidative stress.
During aging, neurons in the hippocampus, cerebellum, and cortex show age-related deficits. Hippocampus plays an important role in learning and memory, and spatial learning is particularly sensitive to hippocampus.
Earlier reports have shown that d-galactose can induce neurodegeneration in hippocampus (Zhong et al. Citation2009; Banji et al. Citation2013). In this current study with d-galactose treatment, we observed the loss of neurons and significant neuronal damage in the hippocampus of mice. This finding might be correlated to the deficits in learning and memory. Morus nigra significantly ameliorated neuronal damage in aging mice which parallels with behavioural changes and the results of biochemical assay.
Conclusions
In conclusion, the results indicate that M. nigra leaf extract significantly improves cognitive impairment in mice treated with d-galactose. The natural phenolic acids, chlorogenic acid and vanillic acid, that have potent antioxidant activity detected in the M. nigra extract may have an important role in this effect which is likely through attenuating oxidative stress by regulating the activities of antioxidant enzymes and scavenging free radicals. We think that the DNA damage protecting the effect of the extract also has an important role in this effect. The current findings suggest that M. nigra may be a beneficial supplement in reducing the speed of aging and delaying neurodegenerative processes. Further research with more detailed work is required to completely understand the molecular mechanisms behind the anti-aging action of M. nigra.
Declaration of interest
The authors report that they have no conflicts of interest. This study was supported by Cumhuriyet University Scientific Research Project V-012 (CUBAP, Sivas, Turkey).
Acknowledgements
The authors would like to thank to Dr. Erol Donmez from the Department of Biology, School of Science, Cumhuriyet University, Sivas, Turkey, for his kind help for the identification of plant material.
References
- Banji D, Banji OJ, Dasaroju S, Annamalai AR. 2013. Piperine and curcumin exhibit synergism in attenuating d-galactose induced senescence in rats. Eur J Pharmacol. 703:91–99.
- Bao Q, Pan J, Qi H, Wang L, Qian H, Jiang F, Shao Z, Xu F, Tao Z, Ma Q, et al. 2014. Aging and age-related diseases – from endocrine therapy to target therapy. Mol Cell Endocrinol. 394:115–118.
- Bradford MM. 1976. A rapid and sensitive method for the quantitation of microgram quantities of protein utilizing the principle of protein-dye binding. Anal Biochem. 72:248–254.
- Calabrese V, Cornelius C, Stella AM, Calabrese EJ. 2010. Cellular stress responses, mitostress and carnitine insufficiencies as critical determinants in aging and neurodegenerative disorders: role of hormesis and vitagenes. Neurochem Res. 35:1880–1915.
- Campos PB, Paulsen BS, Rehen SK. 2014. Accelerating neuronal aging in in vitro model brain disorders: a focus on reactive oxygen species. Front Aging Neurosci. 6:292. doi: 10.3389/fnagi.2014.00292.
- Chou TH, Ding HY, Hung WJ, Liang CH. 2010. Antioxidative characteristics and inhibition of alpha-melanocyte-stimulating hormone-stimulated melanogenesis of vanillin and vanillic acid from Origanum vulgare. Exp Dermatol. 19:742–750.
- Ercisli S, Orhan E. 2007. Chemical composition of white (Morus alba), red (Morus rubra) and black (Morus nigra) mulberry fruits. Food Chem. 103:1380–1384.
- Francisco V, Costa G, Figueirinha A, Marques C, Pereira P, Miguel Neves B, Celeste Lopes M, García-Rodríguez C, Teresa Cruz M, Teresa Batista M. 2013. Anti-inflammatory activity of Cymbopogon citratus leaves infusion via proteasome and nuclear factor-κB pathway inhibition: contribution of chlorogenic acid. J Ethnopharmacol. 148:126–134.
- Fransen M, Nordgren M, Wang B, Apanasets O, Van Veldhoven PP. 2013. Aging, age-related diseases and peroxisomes. Subcell Biochem. 69:45–65.
- Göçer H, Gülçin I. 2011. Caffeic acid phenethyl ester (CAPE): correlation of structure and antioxidant properties. Int J Food Sci Nutr. 62:821–825.
- Gülçin I. 2007. Comparison of in vitro antioxidant and antiradical activities of l-tyrosine and l-Dopa. Amino Acids 32:431–438.
- Hara K, Haranishi Y, Kataoka K, Takahashi Y, Terada T, Nakamura M, Sata T. 2014. Chlorogenic acid administered intrathecally alleviates mechanical and cold hyperalgesia in a rat neuropathic pain model. Eur J Pharmacol. 723:459–464.
- Harman D. 1956. Aging: a theory based on free radical and radiation chemistry. J Gerontol. 11:298–300.
- Hélie S, Paul EJ, Ashby FG. 2012. A neurocomputational account of cognitive deficits in Parkinson's disease. Neuropsychologia 50:2290–2302.
- Hwang SJ, Kim YW, Park Y, Lee HJ, Kim KW. 2014. Anti-inflammatory effects of chlorogenic acid in lipopolysaccharide-stimulated RAW 264.7 cells. Inflamm Res. 63:81–90.
- Imran M, Khan H, Shah M, Khan R, Khan F. 2010. Chemical composition and antioxidant activity of certain Morus species. J Zhejiang Univ Sci B 11:973–980.
- Isabelle M, Lee BL, Ong CN, Liu X, Huang D. 2008. Peroxyl radical scavenging capacity, polyphenolics, and lipophilic antioxidant profiles of mulberry fruits cultivated in southern China. J Agric Food Chem. 56:9410–9416.
- Kancheva VD, Kasaikina OT. 2013. Bio-antioxidants – a chemical base of their antioxidant activity and beneficial effect on human health. Curr Med Chem. 20:4784–4805.
- Kang TH, Hur JY, Kim HB, Ryu JH, Kim SY. 2006. Neuroprotective effects of the cyanidin-3-O-beta-d-glucopyranoside isolated from mulberry fruit against cerebral ischemia. Neurosci Lett. 391:122–126.
- Kim AJ, Park S. 2006. Mulberry extract supplements ameliorate the inflammation-related hematological parameters in carrageenan-induced arthritic rats. J Med Food. 9:431–435.
- Kumar A, Prakash A, Dogra S. 2011. Centella asiatica attenuates d-galactose induced cognitive impairment, oxidative and mitochondrial dysfunction in mice. Int J Alzheimers Dis. 2011:347569.
- Kumar S, Prahalathan P, Raja B. 2014. Vanillic acid: a potential inhibitor of cardiac and aortic wall remodeling in l-NAME induced hypertension through upregulation of endothelial nitric oxide synthase. Environ Toxicol Pharmacol. 38:643–652.
- Kwon SH, Lee HK, Kim JA, Hong SI, Kim HC, Jo TH, Park YI, Lee CK, Kim YB, Lee SY, et al. 2010. Neuroprotective effects of chlorogenic acid on scopolamine-induced amnesia via anti-acetylcholinesterase and anti-oxidative activities in mice. Eur J Pharmacol. 649:210–217.
- Lee K, Lee JS, Jang HJ, Kim SM, Chang MS, Park SH, Kim KS, Bae J, Park JW, Lee B, et al. 2012. Chlorogenic acid ameliorates brain damage and edema by inhibiting matrix metalloproteinase-2 and 9 in a rat model of focal cerebral ischemia. Eur J Pharmacol. 689:89–95.
- Li Y, Shi W, Li Y, Zhou Y, Hu X, Song C, Ma H, Wang C, Li Y. 2008. Neuroprotective effects of chlorogenic acid against apoptosis of PC12 cells induced by methylmercury. Environ Toxicol Pharmacol. 26:13–21.
- Li Z, Zhang Z, Zhang J, Jia J, Ding J, Luo R, Liu Z. 2012. Cordyceps militaris extract attenuates d-galactose-induced memory impairment in mice. J Med Food. 15:1057–1063.
- Lim HH, Lee SO, Kim SY, Yang SJ, Lim Y. 2013. Anti-inflammatory and antiobesity effects of mulberry leaf and fruit extract on high fat diet-induced obesity. Exp Biol Med (Maywood) 238:1160–1169.
- Morris RG, Garrud P, Rawlins JN, O'Keefe J. 1982. Place navigation impaired in rats with hippocampal lesions. Nature 297:681–683.
- Naderi GA, Asgary S, Sarraf-Zadegan N, Oroojy H, Afshin-Nia F. 2004. Antioxidant activity of three extracts of Morus nigra. Phytother Res. 18:365–369.
- Obrenovich ME, Li Y, Parvathaneni K, Yendluri BB, Palacios HH, Leszek J, Aliev G. 2011. Antioxidants in health, disease and aging. CNS Neurol Disord Drug Targets 10:192–207.
- Ode MP. 2000. The complete guide medicinal herbal, second ed. London: Dorling Kindersley.
- Ohkawa H, Ohishi N, Yagi K. 1979. Assay for lipid peroxides in animal tissues by thiobarbituric acid reaction. Anal Biochem. 95:351–358.
- O'Neill C. 2013. PI3-kinase/Akt/mTOR signaling: impaired on/off switches in aging, cognitive decline and Alzheimer's disease. Exp Gerontol. 48:647–653.
- Ooi KL, Muhammad TS, Tan ML, Sulaiman SF. 2011. Cytotoxic, apoptotic and anti-α-glucosidase activities of 3,4-di-O-caffeoyl quinic acid, an antioxidant isolated from the polyphenolic-rich extract of Elephantopus mollis Kunth. J Ethnopharmacol. 135:685–695.
- Orhan E, Ercisli S. 2010. Genetic relationships between selected Turkish mulberry genotypes (Morus spp.) based on RAPD markers. Genet Mol Res. 9:2176–2183.
- Ozçelik B, Kartal M, Orhan I. 2011. Cytotoxicity, antiviral and antimicrobial activities of alkaloids, flavonoids, and phenolic acids. Pharm Biol. 49:396–402.
- Ozturk N, Tuncel M, Tuncel NB. 2008. Determination of phenolic acids by a modified HPLC: its application to various plant materials. J Liq Chromatogr. 30:587–596.
- Rakshit S, Mandal L, Pal BC, Bagchi J, Biswas N, Chaudhuri J, Chowdhury AA, Manna A, Chaudhuri U, Konar A, et al. 2010. Involvement of ROS in chlorogenic acid-induced apoptosis of Bcr-Abl + CML cells. Biochem Pharmacol. 80:1662–1675.
- Russo A, Acquaviva R, Campisi A, Sorrenti V, Di Giacomo C, Virgata G, Barcellona ML, Vanella A. 2000. Bioflavonoids as antiradicals, antioxidants and DNA cleavage protectors. Cell Biol Toxicol. 16:91–98.
- Shih PH, Chan YC, Liao JW, Wang MF, Yen GC. 2010. Antioxidant and cognitive promotion effects of anthocyanin-rich mulberry (Morus atropurpurea L.) on senescence-accelerated mice and prevention of Alzheimer's disease. J Nutr Biochem. 21:598–605.
- Singab AN, El-Beshbishy HA, Yonekawa M, Nomura T, Fukai T. 2005. Hypoglycemic effect of Egyptian Morus alba root bark extract: effect on diabetes and lipid peroxidation of streptozotocin-induced diabetic rats. J Ethnopharmacol. 100:333–338.
- Singh JC, Kakalij RM, Kshirsagar RP, Kumar BH, Komakula SS, Diwan PV. 2015. Cognitive effects of vanillic acid against streptozotocin-induced neurodegeneration in mice. Pharm Biol. 53:630–636.
- Sokmen A, Jones BM, Erturk M. 1999. The in vitro antibacterial activity of Turkish medicinal plants. J Ethnopharmacol, 67:79–86.
- Sroka Z, Cisowski W. 2003. Hydrogen peroxide scavenging, antioxidant and anti-radical activity of some phenolic acids. Food Chem Toxicol. 41:753–758.
- Stanely Mainzen Prince P, Rajakumar S, Dhanasekar K. 2011. Protective effects of vanillic acid on electrocardiogram, lipid peroxidation, antioxidants, proinflammatory markers and histopathology in isoproterenol induced cardiotoxic rats. Eur J Pharmacol. 668:233–240.
- Stefanello N, Schmatz R, Pereira LB, Rubin MA, da Rocha JB, Facco G, Pereira ME, Mazzanti CM, Passamonti S, Rodrigues MV, et al. 2014. Effects of chlorogenic acid, caffeine, and coffee on behavioral and biochemical parameters of diabetic rats. Mol Cell Biochem. 388:277–286.
- Wan CW, Wong CN, Pin WK, Wong MH, Kwok CY, Chan RY, Yu PH, Chan SW. 2013. Chlorogenic acid exhibits cholesterol lowering and fatty liver attenuating properties by up-regulating the gene expression of PPAR-α in hypercholesterolemic rats induced with a high-cholesterol diet. Phytother Res. 27:545–551.
- Wang D, Liu M, Cao J, Cheng Y, Zhuo C, Xu H, Tian S, Zhang Y, Zhang J, Wang F. 2012. Effect of Colla corii asini (E'jiao) on d-galactose induced aging mice. Biol Pharm Bull. 35:2128–2132.
- Volpato GT, Calderon IM, Sinzato S, Campos KE, Rudge MV, Damasceno DC. 2011. Effect of Morus nigra aqueous extract treatment on the maternal-fetal outcome, oxidative stress status and lipid profile of streptozotocin-induced diabetic rats. J Ethnopharmacol. 138:691–696.
- Xian YF, Su ZR, Chen JN, Lai XP, Mao QQ, Cheng CH, Ip SP, Lin ZX. 2014. Isorhynchophylline improves learning and memory impairments induced by d-galactose in mice. Neurochem Int. 76:42–49.
- Yang WN, Han H, Hu XD, Feng GF, Qian YH. 2013. The effects of perindopril on cognitive impairment induced by d-galactose and aluminum trichloride via inhibition of acetylcholinesterase activity and oxidative stress. Pharmacol Biochem Behav. 114115:31–36.
- Yi ZJ, Fu YR, Li M, Gao KS, Zhang XG. 2009. Effect of LTA isolated from bifidobacteria on d-galactose-induced aging. Exp Gerontol. 44:760–765.
- Zhang X, Wang J, Zhang A, Jiang B, Bao Y, An L. 2008. Further pharmacological evidence of the neuroprotective effect of catalpol from Rehmannia glutinosa. Phytomedicine 15:484–490.
- Zhong SZ, Ge QH, Qu R, Li Q, Ma SP. 2009. Paeonol attenuates neurotoxicity and ameliorates cognitive impairment induced by d-galactose in ICR mice. J Neurol Sci. 277:58–64.