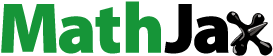
Abstract
Context Biotransformation systems are profitable tools for structural modification of bioactive natural compounds into valuable biologically active terpenoids.
Objective This study determines the biological effect of (R)-(+)-limonene and (−)-α-pinene, and their oxygenated derivatives, (a) perillyl alcohol and (S)-(+)- and (R)-(−)-carvone enantiomers and (b) linalool, trans-verbenol and verbenone, respectively, on human colon tumour cells and normal colonic epithelium.
Materials and methods Biotransformation procedures and in vitro cell culture tests were used in this work. Cells were incubated for 24 h with terpenes at concentrations of 5–500 μg/mL for NR, MTT, DPPH, and NO assays. IL-6 was determined by ELISA with/without 2 h pre-activation with 10 μg/mL LPS.
Results trans-Verbenol and perillyl alcohol, obtained via biotransformation, produced in vitro effect against tumour cells at lower concentrations (IC50 value = 77.8 and 98.8 μg/mL, respectively) than their monoterpene precursors, (R)-(+)-limonene (IC50 value = 171.4 μg/mL) and (−)-α-pinene (IC50 value = 206.3 μg/mL). They also showed lower cytotoxicity against normal cells (IC50 > 500 and > 200 μg/mL, respectively). (S)-(+)-Carvone was 59.4% and 27.1% more toxic to tumour and normal cells, respectively, than the (R)-(−)-enantiomer. (R)-(+)-limonene derivatives decreased IL-6 production from normal cells in media with or without LPS (30.2% and 13.9%, respectively), while (−)-α-pinene derivatives induced IL-6 (verbenone had the strongest effect, 60.2% and 29.1% above control, respectively). None of the terpenes had antioxidative activity below 500 μg/mL.
Discussion and conclusions Bioactivity against tumour cells decreased in the following order: alcohols > ketones > hydrocarbons. (R)-(+)-limonene, (−)-α-pinene, and their derivatives expressed diverse activity towards normal and tumour cells with noticeable enantiomeric differences.
Introduction
In the past few years, chemoprevention has become a promising and widely discussed anticancer strategy. It is mainly directed against cancer initiation, but anti-promotion and even anti-progression strategies are being tested. There are also many areas of potential chemopreventive intervention. Among others, an anti-inflammatory action, free radicals scavenging, modulations of cellular metabolism, enhanced detoxification, and an immunomodulatory activity have been described (Patel et al. Citation2007). These activities are inseparably connected with a large branch of knowledge called phytomedicine, which deals with the therapeutic benefits which can be obtained from the use of plant-derived substances. Many phytochemical constituents are known to exert diverse biological activities including cancer preventive action (Wargovich Citation2001). This potential can be precisely detected using well-defined active agents and technologies which enable reproducible preparation (Kelloff et al. Citation1999).
Phytochemicals contain numerous dietary compounds, including essential oils. Many of those concentrated hydrophobic liquids can be effectively used in the treatment of cancer as antitumour and supportive care agents (Saller et al. Citation2008). The interest in essential oils as potential remedies for cancer originates from the fact that their activity differs from that of classic chemotherapeutics. They are low-toxic to normal tissues, highly tolerable and, therefore, relatively safe. Current research shows that essential oils exert both chemopreventive and chemotherapeutic activities in tumour models; however, clinical trials with these components have yielded conflicting results. While in some cases, essential oils have been shown to exert a significant anticancer activity in vitro (Edris Citation2007; Patel et al. Citation2007), in others, no evidence has been found to support their beneficial effects (Lis-Balchin Citation1999; Halcón Citation2002).
In comparison with the extensive knowledge of crude essential oils, little is known about the bioactivity of their respective constituents (e.g., terpenes), their synergistic or antagonistic interactions and other chemicals which sometimes contaminate essential oils, negatively influencing their precious properties. For this reason, it seems to be a better approach to use single enantiomeric terpenoids or well-defined terpene compositions for tumour treatments.
Since many interesting natural molecules are present only in very small amounts in plant oils, there is justifiable interest in their selective synthesis or production by transformation of other chemicals. Classic chemical synthesis, however, is either not effective in producing terpenes or has many adverse effects on product purity and the environment. Moreover, for numerous valuable terpenoids with an oncotherapeutic potential, especially those which are enantiomerically pure and volatile, no chemical methods of synthesis have been developed so far. Potentially, some desirable unique terpenoids can be selectively produced by enzymatic or microbial transformation of abundant and low-priced terpenes, such as (R)-(+)-limonene and (−)-α-pinene (Bicas et al. Citation2009; Schewe et al. Citation2009; Trytek et al. Citation2009; Fiedurek et al. Citation2012). The antimicrobial activity of some of those compounds has been extensively studied but their action on human normal and tumour cells is still poorly understood.
The aim of the present study was to find out whether biocatalytic oxidation of monoterpenes could result in the formation of terpenoids with valuable bioactive properties. We investigated the biological activity of (a) (R)-(+)-limonene and its derivatives, perillyl alcohol and (S)-(+)- and (R)-(−)-carvone enantiomers, and (b) (−)-α-pinene and its derivatives, linalool, trans-verbenol, and verbenone, toward human colon tumour cells and the normal human colonic epithelium in vitro.
Moreover, mixtures of appropriate derivatives were prepared, and their toxic, free-radical-scavenging, nitric-oxide-inducing, and immunomodulatory activities, expressed as interleukin-6 (IL-6) levels, were analysed.
Materials and methods
General
Analytical-grade chemicals were used as received. (R)-(+)-Limonene (97% and 98% ee), (1S)-(−)-α-pinene (98%), (R)-(−)-carvone (98%), and (S)-(+)-carvone (96%) were purchased from Sigma-Aldrich, St. Louis, MO. (1S)-(−)-Linalool (> 97%) and (1S)-(−)-verbenone at a purity of 97% were obtained from Fluka, St. Gallen, Switzerland. (1S,4R,5S)-(−)-trans-Verbenol (96%) and R-(+)-perillyl alcohol (97%) were obtained in our laboratory by biocatalytic oxidation of (1S)-(−)-α-pinene and (R)-(+)-limonene, respectively.
GC-MS and GC-FID analyses were performed by using non-chiral ZB-5 ms fused silica (Phenomenex, Torrance, CA) or chiral 20% permethylated β-cyclodextrin capillary column (Supelco, Bellefonte, PA).
Neutral Red, 3-(4,5-dimethylthiazole-2-yl)-2,5-diphenyltetrazolium bromide (MTT), stable dark violet radical 1,1-diphenyl-2-picrylhydrazyl (DPPH•), Griess reagent (1% sulphanilamide/0.1% N-(1-naphthyl)ethylenediamine dihydrochloride), (±)-6-hydroxy-2,5,7,8-tetramethylchromane-2-carboxylic acid (Trolox), and lipopolysaccharide (LPS) from Escherichia coli, serotype 0111:B4, were obtained from Sigma-Aldrich Co. LLC, St. Louis, MO. ELISA kits for tests were purchased from Diaclone, Besançon-cedex, France.
General biotransformation procedure
The biotransformation process was carried out in submerged cultures using the psychrotrophic fungi Mortierella minutissima van Tieghem (Mortierellaceae) and Chrysosporium pannorum (Link) S. Hughes (Onygenaceae) isolated from soils collected in the Arctic tundra (Spitsbergen). Fungal cultures and bioconversion experiments were performed in a liquid medium (BM) consisting of malt extract 1%, peptone 0.5%, glucose 1%, and yeast extract 0.5%.
Erlenmeyer flasks containing 50 mL of sterile BM media were inoculated uniformly with 2 mL of fungal spore suspensions (about 4 × 105 spores) and cultivated at 20 °C on a rotary shaker (150 rpm). For biotransformation of (−)-α-pinene with C. pannorum, the substrate was added to the medium sequentially (at a total of 1.5% v/v). Bioconversions were started by adding 0.2% of (−)-α-pinene to a 1-d-old fungal culture and then in doses of 0.3, 0.5, and 0.5% every 12 h, after which bioconversion was continued for 36 h at 20 °C. Total bioconversion time was 72 h. In the case of M. minutissima-based biotransformation, after 72 h of fungal growth, 500 μL of 50% (R)-(+)-limonene solution in methanol was added to the medium and converted for further 48 h at 15 °C.
To identify and quantify all products formed (including phase-partitioned species, which have limited water solubility), after the specified time of biotransformation, the biomass was removed by centrifugation, washed with water and ether, and the liquid obtained was extracted twice in a separatory funnel with an equal volume of diethyl ether. The ether fraction was separated, dried over anhydrous sodium sulphate, and concentrated on rotary vacuum evaporators at a water bath temperature of 40 °C. The residues obtained were dissolved in hexane and analysed by GC-FID and GC-MS. Terpenoid compounds were identified by comparing their retention indexes (RI) with those of standard compounds and by fitting their mass spectra to those from the NIST 2004 Library and the MassFinder3 Library.
Isolation of the main reaction products
In order to obtain sufficient amounts of crude reaction products for their purification and isolation of the final compounds, the ether terpene fractions were collected from 20 bioconversion runs. Combined solutions (containing trans-verbenol and verbenone, or perillyl alcohol) were concentrated to about 600 μL in a rotary evaporator under reduced pressure.
The crude mixture of products was fractionated, using a flash silica gel column (Fluka 60, 70–230 mesh). Columns were typically run using a gradient method, beginning with 100% hexane and gradually increasing the polarity using ethyl acetate or dichloromethane to elute trans-verbenol and perillyl alcohol, respectively. Fractions of 5 ml were collected and analysed by GC. The fractions which contained desired terpenoids were combined.
The elution of the column with a gradient of 100:0–60:40 hexane/EtOAc gave trans-verbenol [0.482 g; (3.7%) based on (−)-α-pinene]. The use of the gradient 100:0–75:25 hexane/CH2Cl2 yielded enantiopure (+)-perillyl alcohol [0.326 g; (7.7%) based on (R)-(+)-limonene]. Individual terpenes were isolated from the fraction by concentration on a vacuum evaporator and re-crystallisation from methanol. The purity of the final products was found to be > 96% and 97%, which was determined by GC/MS analysis for trans-verbenol and perillyl alcohol, respectively, and the identity of the products was confirmed by 1H and 13C NMR.
For 1H NMR, the following symbols were used: J coupling constant, δ chemical shift, s singlet, d doublet, t triplet and m multiplet.
Trans-verbenol (as a liquid); [m/z (% of base peak)] 41 (88), 55 (67), 59 (49), 67 (61), 69 (54), 79 (45), 81 (68), 91 (69), 94 (66), 109 (100), 119 (32), 134 [M+ − H2O (10)], 137 [M+ − CH3 (9)], 152 [M+(2.0)]
1H NMR (500 MHz, CDCl3) δ 5.32 (m,1H, J = 3.1, 1.5 Hz, H-3), 4.24 (m, 1H, J = 3.1, 1.5 Hz, H-4), 2.23 (m, 1H, J = 9.1, 5.5 Hz, H-7a), 2.15 (tdd, 1H, J = 5.7, 1.8 Hz, H-5), 2.00 (td, 1H, J = 5.4, 1.3 Hz, H-1) 1.70 (t, 3H, J = 1.6 Hz, H-10), 1.30 (s, 3H, H-8), 1.26 (m, 1H, H-7b), 0.85 (s, 3H, H-9).
13C NMR (100 MHz, CDCl3) δ 148.7 (C-2), 118.8 (C-3), 70.4 (C-4), 48.1 (C-1), 47.1 (C-5), 46.2 (C-6), 28.6 (C-7), 26.6 (C-8), 22.5 (C-10), 20.4 (C-9).
(+)-Perillyl alcohol (as a liquid); [m/z (% of base peak)] 41.00 (65), 53.00 (41), 55.00 (56), 67.00 (95), 68.00 (100), 77.00 (34.20), 79.00 (91), 91 (48), 93 (55), 105 (17), 109 (18), 119 (27), 121 (41), 134 [M+ − H2O (11)], 152 [M+(6)]
1H NMR (300 MHz, CDCl3) δ 5.70 (t, 1H, H-2), 4,73 (d, 2H, J = 2.0 Hz H-9), 4,00 (s, 2H, H-7), 2.27-2.08 (m, 7H) 1,75 (s, 3H, H-10), 1.60 (t, 1H, J = 4.8 Hz, –OH).
13C NMR (75 MHz, CDCl3) δ 150.2 (C, C-8), 137.6 (C, C-1), 122.8 (CH, C-2), 109.3 (CH2, C-9), 67.5 (CH2-OH, C-7), 41.5 (CH, C-4), 30.8 (CH2, C-5), 27.9 (CH2, C-3) 26.5 (CH2, C-6), 21.2 (CH3, C-10)
Identification of different diasteromers was corroborated by comparing known standards using GC-MS analysis and a chiral column. The chemical purity and the ee (stereoisomerism) of each terpene were respectively determined by gas chromatography using a non-chiral (GC-MS) and a chiral (20% permethylated β-cyclodextrin) column. The chemical purity and the ee of (1S,4R,5S)-(−)-trans-verbenol and R-(+)-perillyl alcohol were 96%, 74% ee and 97%, 95% ee, respectively.
Cell cultures
Human colon adenocarcinoma cell line HT29 (ATCC no. HTB-38), derived from a grade I tumour, was cultured in RPMI 1640 medium supplemented with 10% foetal calf serum (FCS) (GibcoTM, Paisley, UK) and antibiotics (100 U/mL penicillin and 100 μg/mL streptomycin) (Sigma, St. Louis, MO) at 37 °C in a humidified atmosphere with 5% CO2.
Human normal colon epithelial cells CCD 841 CoTr (ATCC no. CRL-1807) were cultured in RPMI 1640 + DMEM (1:1) medium (Sigma, St. Louis, MO) supplemented with 10% FCS and antibiotics at 34 °C in a 5% CO2/95% air atmosphere.
Test substances
(R)-(+)-Limonene and (1S)-(−)-α-pinene were used as terpene precursors. The following derivatives of (R)-(+)-limonene were tested: perillyl alcohol (obtained by biocatalytic oxidation in our laboratory) and carvone enantiomers, (R)-(−) and (S)-(+). (−)-α-Pinene derivatives were as follows: trans-verbenol (obtained by biocatalytic oxidation in our laboratory), (1S)-(−)-verbenone, and (1S)-(−)-linalool. Moreover, the set of material tested consisted of mixtures (MIX) of (R)-(+)-limonene derivatives: perillyl alcohol and (S)-(+) and (R)-(−)-carvone enantiomers (1:1:1 v/v/v), and (−)-α-pinene derivatives: linalool, trans-verbenol, and verbenone (1:1:1 v/v/v). Both initial terpenes and their derivatives were used for activity testing. The purpose of testing additional compounds was to analyse whether they express more advantageous biological activity against tumour cells than initial terpene.
Predetermined amounts of the terpenes were dissolved in dimethyl sulphoxide (DMSO, Sigma, St. Louis, MO) to obtain stock solutions (100 mg/mL). Working solutions were prepared by dissolving the stock solutions in the culture medium. A 5–500 μg/mL range of concentrations was used in the experiments. During incubation of cells with the terpenes, culture plates were sealed with parafilm.
Neutral red (NR) uptake assay
The NR cytotoxicity assay is based on the uptake and lysosomal accumulation of the supravital dye, neutral red. Dead or damaged cells do not take up this dye (Ganbold et al. Citation2010). Cells were grown for 24 h in 96-well multiplates in 100 μL of culture medium with appropriate concentrations of essential oils. Subsequently, the medium was discarded, and 0.4% NR solution medium was added to each well. The plate was incubated for 3 h at 37 °C in a humidified 5% CO2/95% air incubator. After incubation, the dye-containing medium was removed and the cells were fixed with 1% CaCl2 in 4% paraformaldehyde. Next, the incorporated dye was solubilised using 1% acetic acetate in a 50% ethanol solution (100 μL). The plates were gently shaken for 20 min at room temperature, and the absorbance of the extracted dye was measured spectrophotometrically at 540 nm using a microplate reader (Molecular Devices Corp., Emax, Menlo Park, CA).
MTT assay
Cell sensitivity of the terpenes tested was determined in a standard spectrophotometric (MTT) assay according to method of Mosmann (Citation1983). MTT is based on the conversion of yellow tetrazolium salt by viable cells to purple crystals of formazan. The reaction is catalysed by mitochondrial succinyl dehydrogenase.
After 24 h incubation of cells with the terpenes in 100 μL of culture medium, MTT solution (5 mg/mL, 25 μL/well) was added and incubated for additional 3 h. The purple crystals of formazan which formed in the medium were solubilised overnight in 10% sodium dodecyl sulphate (SDS) in a 0.01 M HCl mixture. The product was quantified spectrophotometrically by measuring its absorbance at 570 nm using an Emax Microplate Reader (Molecular Devices Corporation, Menlo Park, CA).
DPPH free radical scavenging test
Free radical scavenging activity of terpene was measured by the DPPH assay. This method is based on the ability of antioxidants to reduce the stable dark violet radical DPPH• (Sigma, St. Louis, MO) to the yellow coloured diphenyl-picrylhydrazyl. Briefly, 100 μL of DPPH• solution (0.2 mg/mL in ethanol) was added to 100 μL of the tested terpene concentrations (5–500 μg/mL). Hundred microliters of increasing concentrations (1–50 μg/mL) of Trolox (Sigma, St. Louis, MO) was used as a standard. After 20 min of incubation at room temperature, the absorbances of the solutions were measured at 515 nm. The lower the absorbance, the higher was the free radical scavenging activity of the terpene. The activity of each terpene was determined by comparing its absorbance with that of a control solution (reagents without the terpene) and the standard.
The ability of the terpenes to scavenge the DPPH• radical was calculated by the following formula:
Xcontrol is the absorbance of the control and Xterpene is the absorbance in the presence of essential oils (Paduch et al. Citation2008).
Nitric oxide (NO) measurement
Nitrite, a stable end product of NO conversion, was determined in culture supernatants by a spectrophotometric method based on the Griess reaction. The level of nitrite reflects NO production (Muzitano et al. Citation2011).
Briefly, tumour and normal cells were incubated for 24 h with the terpenes tested, and then culture supernatants were collected. Next, 100 μL of each supernatant was plated in 96-well flat-bottomed plates in triplicate and incubated with 100 μL of Griess reagent in 3% H3PO4 (POCH, Gliwice, Poland) at room temperature for 10 min. The optical density was measured at 550 nm using a microplate reader (Molecular Devices Corp., Emax, Menlo Park, CA). A standard curve was obtained using 0.5–25 μM sodium nitrite (NaNO2) for calibration.
ELISA assay
The level of human IL-6 was tested immunoenzymatically (ELISA) in culture supernatants using commercially available kits (Diaclone, Ann Arbor, MI) according to the manufacturer’s instruction. The optical density of each ELISA sample was determined using a microplate reader set to 450 nm with a correction wavelength of 570 nm. IL-6 concentrations were calculated on the basis of a standard curve; 2 pg/mL of the cytokine was the detection limit.
In parallel experiments, similar to nitric oxide analysis by the Griess method, cells were pre-activated for 2 h with 10 μg/mL LPS from Escherichia coli, serotype 0111:B4 (Sigma, St. Louis, MO), and then incubated with the investigated terpenes at a concentration of 25 μg/mL for 24 h. After the incubation, supernatants were collected and stored at −80 °C until the IL-6 assay was performed.
Statistical analysis
Results are presented as means ± SD from three experiments. Data were analysed using one-way ANOVA with Dunnett’s post hoc test. Differences of p ≤ 0.01 were considered significant.
Results
Biotransformation and reaction products
To find out whether monoterpene bioconversion could be used as a potential method for obtaining substances with an anticancer activity, (R)-(+)-limonene and (−)-α-pinene were subjected to biotransformation using the psychrotrophic fungi Mortierella minutissima and Chrysosporium pannorum, respectively. These biocatalysts had previously been found to be very active and display a high degree of regiospecificity (Trytek et al. Citation2009, Citation2014). A GC-MS analysis of the products obtained indicated that the main terpenoids produced during the biotransformation of (−)-α-pinene by C. pannorum were compounds, trans-verbenol and verbenone, of a high commercial value. In turn, the biooxidation of (R)-(+)-limonene in mycelial cultures of M. minutissima yielded a high accumulation of perilla alcohol with a minor amount of perillaldehyde. Determination of product stereochemistry (chiral GC analysis) revealed that these bioconversions showed a high enantioselectivity.
Typical chromatograms resulting from the biotransformation of (R)-(+)-limonene and (−)-α-pinene are presented in , respectively. Two main products of the biotransformation of the monoterpenes were successfully isolated and purified from the post-reaction medium with a yield that allowed them to be used in this study. These valuable terpenoids occur in plant oils only in very small amounts, insufficient for large-scale use. The amounts of other minor terpenoid products, such as verbenone, linalool, and perillaldehyde were too low for the compounds to be investigated without prior optimisation of their bioproduction. Due to this, commercially available chemicals were employed as potential biotransformation products.
Figure 1. A GC-FID chromatogram and structures of main metabolites recovered after biotransformation of (R)-(+)-limonene by M. minutissima; IS, internal standard (A). Main products and a GC-FID chromatogram of the post-reaction mixture after (−)-α-pinene biotransformation by C. pannorum. IS, internal standard (B).
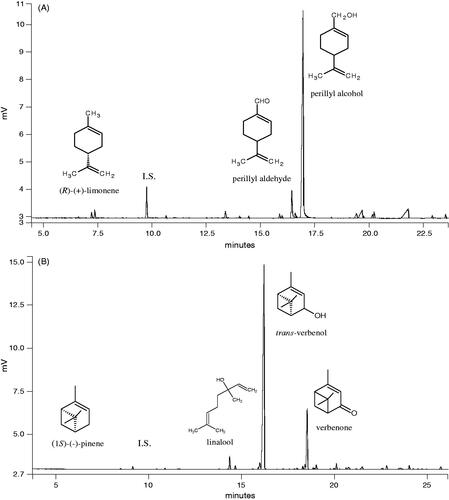
Biological activity of the precursors
Of the two monoterpenic precursors tested, (R)-(+)-limonene (at concentrations of 0–200 μg/mL), in general, showed a slightly higher cytotoxic activity toward normal cells than (−)-α-pinene when analysed by MTT (, respectively). On the other hand, in the NR assay, (R)-(+)-limonene was observed to stimulate the metabolic activity of normal cells. Neither of the precursors was found to exert any action against tumour cells in the MTT assay. Using the NR method, more pronounced differences between (−)-α-pinene and (R)-(+)-limonene anticancer activity were revealed ().
Figure 2. The effect of 24 h treatment of HT29 (A and C) and CCD 841 CoTr (B and D) cells with (R)-(+)-limonene and its derivatives. MTT assay (A and B) and Neutral Red assay (NR) (C and D). The results are presented as a percentage of the controls, arbitrarily set to 100%. The figure shows an average of three independent experiments.
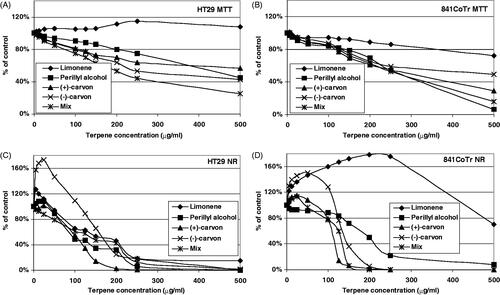
Figure 3. The effect of 24 h treatment of HT29 (A and C) and CCD 841 CoTr (B and D) cells with (−)-α-pinene and its derivatives. MTT assay (A and B) and Neutral Red assay (NR) (C and D). The results are presented as a percentage of the controls, arbitrarily set to 100%. The figure shows an average of three independent experiments.
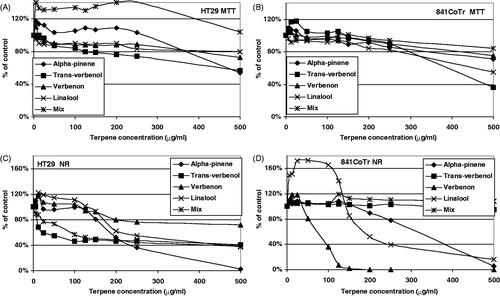
(−)-α-Pinene decreased NO in relation to the control (without pre-incubation with LPS) and increased NO when (normal and tumour) cells were incubated with LPS for 2 h (). An opposite behaviour was observed for the precursor of (R)-(+)-limonene in both normal and tumour cells (). Moreover, (R)-(+)-limonene significantly decreased the IL-6 level after pre-incubation of the cells with LPS as compared with (−)-α-pinene, which decreased the amount of this inflammatory mediator only slightly (). An MTT analysis of cellular metabolism revealed that (R)-(+)-limonene derivatives exerted a stronger effect on both normal and tumour cells than (−)-α-pinene derivatives (, respectively). Moreover, both the initial terpenes displayed a lower activity against tumour cells than their derivatives. An interesting observation was that the mixtures of the derivatives of the two precursors had opposite actions on tumour cells. While the mixture of (R)-(+)-limonene derivatives strongly inhibited cellular metabolism, (−)-α-pinene derivatives slightly induced succinyl dehydrogenase activity as compared with the control. Similar observations were made for normal cells (MTT method). Also, a reverse relationship between the NO level and the action of (R)-(+)-limonene and (−)-α-pinene derivatives was found for these cells, both when they were and were not pre-incubated with LPS ( and ). The toxicity of the terpenes was evaluated using the Neutral Red (NR) uptake assay ().
Figure 4. IL-6 secretion in culture of CCD 841 CoTr cells during 24 h incubation with (R)-(+)-limonene or (−)-α-pinene and their derivatives. ELISA test. *p ≤0.01 – a culture treated with terpenes compared with a non-treated control culture. #p ≤0.01 – a culture treated with terpenes compared with a non-treated control culture after pre-incubation with LPS.
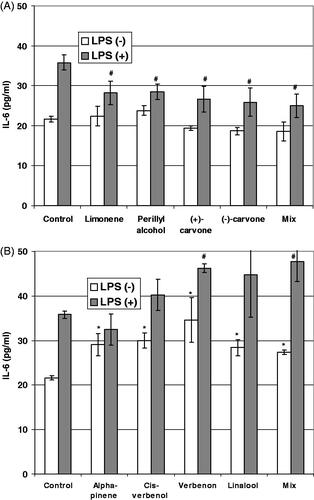
Table 1. Nitric oxide production by human colon tumor cell (HT29) and normal colonic epithelial cell (CCD 841 CoTr) after 24 h incubation with (R)-(+)-limonene and its derivatives.
Table 2. Nitric oxide production by human colon tumour cell (HT29) and normal colonic epithelial cell (CCD 841 CoTr) after 24 h incubation with (−)-α-pinene and its derivatives.
(R)-(+)-Limonene and its derivatives
A significant and intense decrease in the viability of normal cells was observed when the terpenes were used at concentrations exceeding 100–125 μg/mL. One exception was (R)-(+)-limonene, which had no disadvantageous effect on normal cells even at concentrations exceeding 400 μg/mL. Terpenes (the two carvone enantiomers tested and the mix of all of the tested (R)-(+)-limonene derivatives) at a concentration of 200 μg/mL caused the death of 100% normal cells; additionally, at 300 μg/mL, perillyl alcohol reduced cell viability below 20.0%.
There was a gradual decrease in tumour cell viability after the application of (R)-(+)-limonene and its derivatives. However, similarly, as in the case of normal cells, (R)-(+)-limonene was the least toxic compound. All the terpenes tested decreased tumour cell viability below 20.0% when their concentrations were higher than 250 μg/mL. (R)-(−)-Carvone expressed a weaker toxic activity compared with its (S)-(+) enantiomer. This relationship was observed both in tumour and in normal cell cultures using the NR method. A noticeable enantiomeric discrepancy between the two types of cells was found in the MTT assay. Tumour cells showed a much higher sensitivity to (R)-(−)-carvone, whereas normal cells were a little more susceptible to (S)-(+)-carvone. IC50 values in the tested range of concentrations (5–500 μg/mL) are presented in .
Table 3. IC50 values obtained after human colon tumour cell (HT29) and human normal colonic epithelial cell (CCD 841 CoTr) after 24 h incubation with (R)-(+)-limonene and (−)-α-pinene and their derivatives (MTT and Neutral Red analysis).
Within the applied, initially specified, range of concentrations (5–500 μg/mL), none of the terpenes had an antioxidative activity as measured by the DPPH scavenging method. Therefore, higher concentrations (1, 2.5, 5, and 10 mg/mL) were tested. (R)-(+)-Limonene and perillyl alcohol, similar to (−)-α-pinene and its derivatives, still failed to reduce the expression of reactive oxygen species (ROS). A slight linear increase in scavenging action was only observed for the two carvone enantiomers and the mix of (R)-(+)-limonene derivatives when used at a very high concentration (10 mg/mL). The increase relative to a control (pure methanol, 0% of scavenging) was 39.0% for (S)-(+)-carvone (which corresponded to 25.0 μg/mL of Trolox), 7.0% for (R)-(−)-carvone (6.0 μg/mL of Trolox), and 16.0% for the mix of (R)-(+)-limonene derivatives (10.0 μg/mL of Trolox).
The next step of this study was to investigate the potential immunomodulatory activity of the test compounds (). The levels of interleukin-6 (IL-6) were analysed by ELISA. Two parallel experiments were performed. One relied on the direct influence of the terpenes on IL-6 production by normal and tumour cells; the other was based on the analysis of the cytokine levels after a 2 h pre-incubation of the cells with LPS from E. coli followed by incubation with the terpenes. The aim of the pre-incubation was to implement an in vitro inflammatory state into our model, which could not only change the level of IL-6 but also modulate the activity of the terpenes. Human colon tumour cells did not produce significant amounts of IL-6 either with or without pre-incubation with LPS. Moreover, the terpenes did not induce IL-6 production by tumour cells. (R)-(+)-Limonene and its derivatives had no significant influence on the production of this cytokine by normal cells but decreased its level when the cells were pre-incubated with LPS.
(−)-α-Pinene and its derivatives
(−)-α-Pinene and its derivative linalool exhibited a low toxicity at concentrations below 150 μg/mL. The mix of (−)-α-pinene derivatives showed a limited toxicity toward normal cells while significantly reducing the viability (NR method) of tumour cells. A similar activity was observed when trans-verbenol and verbenone were compared. Our results showed that trans-verbenol significantly reduced the viability of tumour cells, having no influence on normal cells. Inversely, verbenone had a weak impact on tumour cell cultures, but significantly reduced the viability of normal cells already at low concentrations (50 μg/mL). IC50 values in the tested concentration range (5–500 μg/mL) are presented in .
(−)-α-Pinene and its derivatives increased IL-6 production in normal human colon epithelial cells. Pre-incubation of the cells with LPS additionally increased the level of this cytokine.
Discussion
Pharmaceutical properties of aromatic plants are closely linked with the action of essential oils (EOs) (volatile oils), which are complex, multi-component mixtures composed of mono- and sesquiterpenoids and non-terpene molecules (Edris Citation2007; Adorjan & Buchbauer Citation2010). As they contain these substances, EOs can exert many biological actions on animals and humans. In the present study, an attempt was made to determine whether individual ingredients of EOs, particularly (R)-(+)-limonene and (−)-α-pinene, and their oxygenated derivatives, had an influence on normal and tumour human colon cells. We also analysed differences in the action of mixtures of the derivatives versus initial terpenes used as precursors in a biotransformation reaction. Moreover, the enantiosensitivity of tumour and normal cells was tested using two enantiomers of carvone.
It is known that most therapeutically applied cytostatics not only have a significant impact on tumour cell proliferation but also affect the viability of normal cells, e.g., those of the alimentary canal walls. Therefore, there is a need to search for new substances characterised by selective action against cancer. It is a difficult conception, but natural substances may act differently than cytostatics. Their mechanism of action enables them to induce an effect on pathways other than those affected by currently applied drugs; one of the most important of those effects is the influence on mevalonate metabolism, which may raise the hope of creating new tumour growth suppressors (Elson Citation1995).
Among some of the most promising substances are essential oils, which have not only been proposed as chemopreventive but also as chemotherapeutic agents. It has already been demonstrated that essential oils are able to inhibit tumour cell growth (Búfalo et al. Citation2010; Sertel et al. Citation2011; Döll-Boscardin et al. Citation2012). In the present study, we also showed that some ingredients of EOs had a cytotoxic activity, but, apart from that, we demonstrated that initial terpene hydrocarbons, i.e., (R)-(+)-limonene and (−)-α-pinene, had a relatively low effect compared with their oxidised derivatives. Similar observations have been made by Sun (Citation2007), who reported that (+)-limonene had a fairly low toxicity. In our study, however, higher concentrations of (R)-(+)-limonene and (−)-α-pinene did influence cell viability and metabolism, which could have been a result of their verified inhibitory activity on the NF-κB signalling pathway, where they inhibit DNA binding and p65 translocation. In consequence, proliferation of cells and even metastasis of a tumour is limited (Salminen et al. Citation2008). Moreover, d-limonene can partially influence farnesyl protein transferase (FTPase) activity and, via this action, it may inhibit the expression of plasma-membrane-associated P21ras and its subsequent isoprenylation (Edris Citation2007). (R)-(+)-Limonene seems to act better than (−)-α-pinene, probably due to differences in their chemical structure; the former is a monocyclic and the latter a bicyclic terpene hydrocarbon. It is known that biological effects of mono- and bicyclic monoterpenes depend on the number of cyclic skeletons and the presence of specific groups (Loza-Tavera Citation1999; Hwang & Hagiwara Citation2008). The presence of at least one hydroxyl group in monoterpenes affects their anticancer and chemopreventive activities much stronger than the absence of such a group (Loza-Tavera Citation1999).
In the present study, oxidised derivatives of (−)-α-pinene and (R)-(+)-limonene produced an effect at lower concentrations than their precursors. This may be associated with their different bioavailabilities to cells, which, in turn, may follow from better solubility in a polar environment as well as the tendency of cells to bind specific receptors by means of hydroxyl or carbonyl groups. Moreover, oxidised derivatives may act more selectively on cells or, more precisely, on specific metabolic pathways, than initial compounds. It has been shown that perillyl alcohol acts on proteins by controlling various aspects of cell viability, e.g. by increasing growth factor receptors, decreasing Ras protein prenylation and ubiquinone synthesis, or inducing cellular detoxification systems (Belanger Citation1998). The differences in the activity of the derivatives may also be responsible for their specific influence on normal and tumour cells when they (the derivatives) are administered as properly prepared mixtures. In general, alcohols show a higher activity against tumour cells but a much weaker activity toward normal cells as compared to ketones. We found that the mixture of (R)-(+)-limonene derivatives demonstrated interesting tumour cell growth-inhibiting properties. However, it needs to be emphasised that such findings cannot be analysed one way and accepted without further investigation. As it was revealed in this study, a mixture of (−)-α-pinene derivatives may, admittedly, have decreased the viability of tumour cells but it simultaneously induced their metabolism. Therefore, experiments in this area need to be continued.
In the present study, a specific relationship was also found between the stereoisomers of carvone. (S)-(+)-Carvone had a stronger impact on the viability of both normal and tumour cells than its (R)-(−) enantiomer. It is known that stereoisomers may differ in their pharmacokinetics and their profiles of undesirable effects. Therefore, application of only one enantiomer makes it possible to increase effectiveness, reduce the recommended dose and decrease the potential toxicity connected with the presence of non-active stereoisomers in the racemic mixture. Moreover, the biological activities of stereoisomers may be radically different, e.g. with one having a medicinal effect, while the other does not express any activity or is a potential mutagen (Smith Citation2009). It was shown in the present study that (S)-(+)-carvone was more toxic to cells than its (R)-(−) enantiomer. This may be associated with their differing affinity for receptors, different potential transporters, different impact on metabolic pathways and a different strength of action (Stammati et al. Citation1999; Zienowicz et al. Citation2003). On the other hand, the two carvone enantiomers exhibited a similar action when succinyl dehydrogenase activity was tested, although tumour cells were much more sensitive to (R)-(−)-carvone. We also found differences in toxicity between trans-verbenol (with a hydroxyl group) and verbenone (with a ketone group). Trans-verbenol showed a significantly weaker beneficial activity toward normal cells than verbenone, having a relatively strong effect on tumour cells. Such results may be due to the different chemical activities of the two groups, but also the activity of the derivatives themselves could play an essential role here. In connection with the above, Wolski et al. (Citation2001) have shown that a high concentration of terpenes, among others verbenone, may be connected with a significant microbiological activity of plant extracts.
In the present study, the terpenes tested showed no free radical scavenging activity. This may have been an effect of the application of relatively low concentrations of these compounds, because when higher concentrations were used, antioxidant activity was detected. Similar to our results, Yang et al. (Citation2010) have found that α-pinene had an only slight DPPH-radical scavenging activity compared with limonene. The mechanism of the action of perillyl alcohol, which is a limonene derivative, is based not only on its antioxidant properties but also on its tumour-modifying effects and its general effects on cell biology (Wargovich Citation2001). It is also worth mentioning that in this present study, the (S)-(+)-stereoisomer of carvone had a 5.5-fold higher antioxidative activity than the (R)-(−)-stereoisomer. This finding indicates that steric limitations may also influence the ROS scavenging potential of compounds.
The immunomodulatory activity of the terpenes studied was evaluated by testing the levels of the most important pro-inflammatory cytokine, IL-6. Increased levels of this cytokine are found in patients with colon carcinoma, and can be used as a prognostic marker of tumour progression (Atreya & Neurath Citation2005). However, IL-6 originates mainly from normal colonic cells and not from colonic tumour cells. In our study, terpenes had diverse effects on IL-6 production by normal colon epithelial cells, but when tumour cells were analysed, no IL-6 or only trace amounts of it were detected. Monocyclic (R)-(+)-limonene and bicyclic (−)-α-pinene influenced IL-6 levels differently. A similar effect could also be observed when NO was analysed. (R)-(+)-Limonene and its derivatives, in contrast to (−)-α-pinene derivatives, reduced in vitro production of IL-6 by lipopolysaccharide (LPS)-activated normal human colon cells. This is consistent with the results of Hart et al. (Citation2000), who have made similar observations concerning the production of pro-inflammatory cytokines by activated human blood monocytes. Essential oils are used to restrict inflammation by influencing molecular pathways, inhibiting nuclear translocation of NF-κB or suppressing pro-inflammatory mRNA expression in cells (Juhás et al. Citation2009). There are, however, reports demonstrating that cells treated with EOs can induce IL-6 production (Sforcin et al. Citation2009). Therefore, it is important to carefully select terpenes for induction of cellular effects. In our study, we observed an insignificant increase in IL-6 production by the normal human colonic epithelium after incubation of the cells with linalool. Sforcin et al. (Citation2009) have revealed that lemongrass essential oil, rich in linalool oxide and epoxy-linalool oxide, reduced IL-1β but induced IL-6 production by mouse macrophages. Nevertheless, the authors have stated in conclusion that a water extract of this natural product exhibits an anti-inflammatory action (Sforcin et al. Citation2009).
Conclusions
Oxidative biotransformation of (R)-(+)-limonene and (−)-α-pinene by psychrotrophic fungi provides an opportunity to obtain oxygenated products, generally regarded as safe, with a considerably higher bioactivity against human colonic cells as compared with their precursors.
(R)-(+)-Limonene, (−)-α-pinene and their derivatives express diverse activities towards normal and tumour colon cells. The detailed analysis of the cytotoxic experiments with terpenic compounds has shown that
Monocyclic terpenoids generally have a higher impact on colonic tumour cells than bicyclic terpenoids; however, bicyclic-oxygenated derivatives should be considered when selectivity for tumour cells is in question.
There is a general correlation between the chemical structures of terpenes and their bioactivity. Bioactivity against tumour cells decreases in the following order: alcohols > ketones > hydrocarbons.
Due to their cytotoxic activity, selected individual ingredients or balanced terpene mixtures may be considered as useful supplements to conventional therapies. Moreover, they could also be taken into account as immunoregulatory factors owing to their capacity to modulate pro-inflammatory cytokines. Generally, EOs that contain the tested terpenes as natural products may positively affect human health, exerting slight or no side effects. Studies on the biological effects of selected terpenes found in currently applied substances with antitumour activity are in progress.
Declaration of interest
The authors report that they have no conflict of interest. The authors would like to thank Maria Curie-Skłodowska University in Lublin, Poland, for providing institutional funds to support this research.
References
- Adorjan B, Buchbauer G. 2010. Biological properties of essential oils: an updated review. Flavour Fragr J. 25:407–426.
- Atreya R, Neurath MF. 2005. Involvement of IL-6 in the pathogenesis of inflammatory bowel disease and colon cancer. Clin Rev Allergy Immunol. 28:187–195.
- Belanger JT. 1998. Perillyl alcohol: applications in oncology. Altern Med Rev. 3:448–457.
- Bicas JL, Dionìsio AP, Pastore GM. 2009. Bio-oxidation of terpenes: an approach for the flavor industry. Chem Rev. 109:4518–4531.
- Búfalo MC, Candeias JM, Sousa JP, Bastos JK, Sforcin JM. 2010. In vitro cytotoxic activity of Baccharis dracunculifolia and propolis against Hep-2 cells. Nat Prod Res. 24:1710–1718.
- Döll-Boscardin PM, Sartoratto A, de Noronha Sales Maia BHL, de Paula JP, Nakashima T, Farago PV, Kanunfre CC. 2012. In vitro cytotoxic potential of essential oils of Eucalyptus benthamii and its related terpenes on tumor cell lines. Evid Bas Complement Altern Med. Article ID 342652, 8 pages.
- Edris AE. 2007. Pharmaceutical and therapeutic potentials of essential oils and their individual volatile constituents: a review. Phytother Res. 21:308–323.
- Elson C. 1995. Suppression of mevalonate pathway activities by dietary isoprenoids: protective roles in cancer and cardiovascular disease. J Nutr. 125:1666S–1672S.
- Fiedurek J, Trytek M, Skowronek M. 2012. Strategies for improving the efficiency of bioprocesses involving toxic compounds. Curr Org Chem. 16:2946–2960.
- Ganbold M, Barker J, Ma R, Jones L, Carew M. 2010. Cytotoxicity and bioavailability studies on a decoction of Oldenlandia diffusa and its fractions separated by HPLC. J Ethnopharmacol. 131:396–403.
- Halcón LL. 2002. Aromatherapy: therapeutic applications of plant essential oils. Minn Med. 85:42–46.
- Hart PH, Brand C, Carson CF, Riley TV, Prager RH, Finlay-Jones JJ. 2000. Terpinen-4-ol, the main component of the essential oil of Melaleuca alternifolia (tea tree oil), suppresses inflammatory mediator production by activated human monocytes. Inflamm Res. 49:619–626.
- Hwang J, Hagiwara H. 2008. Effect of mono-and bicyclic monoterpenoids upon Dictyostelium minutum and Dictyostelium macrocephalum. J Essent Oil Res. 20:91–95.
- Juhás Š, Bukovská A. Čikoš Š, Czikková S, Fabian D, Koppel J. 2009. Anti-inflammatory effects of Rosmarinus officinalis essential oil in mice. Acta Vet Brno. 78:121–127.
- Kelloff GJ, Crowell JA, Steele VE, Lubet RA, Boone ChW, Malone WA, Hawk ET, Lieberman R, Lawrence JA, Kopelovich L, et al. 1999. Progress in cancer chemoprevention. Ann N Y Acad Sci. 889:1–13.
- Lis-Balchin M. 1999. Possible health and safety problems in the use of novel plant essential oils and extracts in aromatherapy. J R Soc Promot Health. 119:240–243.
- Loza-Tavera H. 1999. Monoterpenes in essential oils. Biosynthesis and properties. Adv Exp Med Biol. 464:49–62.
- Mosmann T. 1983. Rapid colorimetric assay for cellular growth and survival: application to proliferation and cytotoxicity assays. J Immunol Methods. 65:55–63.
- Muzitano MF, Bergonzi M, De Melo GO, Lage CLS, Bilia AR, Vincieri FF, Rossi-Bergmann B, Costa SS. 2011. Influence of cultivation conditions, season of collection and extraction method on the content of antileishmanial flavonoids from Kalanchoe pinnata. J Ethnopharmacol. 133:132–137.
- Paduch R, Matysik G, Wójciak-Kosior M, Kandefer-Szerszeń M, Skalska-Kamińska A, Nowak-Kryska M, Niedziela P. 2008. Lamium album extracts express free radical scavenging and cytotoxic activities. Polish J Environ Stud. 17:569–580.
- Patel R, Garg R, Erande S, Maru GB. 2007. Chemopreventive herbal anti-oxidants: current status and future perspectives. J Clin Biochem Nutr. 40:82–91.
- Saller R, Reichling J, Rostock M, Iten F, Melzer J. 2008. Gastointestinale Symptome bei tumorkranken Menschen–phytotherapeutische Behandlungsmöglichkeiten. Schweiz Zschr GanzheitsMed. 20:221–230.
- Salminen A, Lehtonen M, Suuronen T, Kaarniranta K, Huuskonen J. 2008. Terpenoids: natural inhibitors of NF-kappaB signaling with anti-inflammatory and anticancer potential. Cell Mol Life Sci. 65:2979–2999.
- Schewe H, Holtmann D, Schrader J. 2009. P450(BM-3)-catalyzed whole-cell biotransformation of alpha-pinene with recombinant Escherichia coli in an aqueous-organic two-phase system. Appl Microbiol Biotechnol. 83:849–857.
- Sertel S, Eichhorn T, Plinkert PK, Efferth T. 2011. Krebshemmende Wirkung des ätherischen Salbei-Öls auf eine Plattenepithelzellkarzinom-Zelllinie der Mundhöhle (UMSCC1). HNO 59:1203–1208.
- Sforcin JM, Amaral JT, Fernandes Jr A, Sousa JPB, Bastos JK. 2009. Lemongrass effects on IL-1beta and IL-6 production by macrophages. Nat Prod Res. 23:1151–1159.
- Smith SW. 2009. Chiral toxicology: it's the same thing ... only different. Toxicol Sci. 110:4–30.
- Stammati A, Bonsi P, Zucco F, Moezelaar R, Alakomi H-L, von Wright A. 1999. Toxicity of selected plant volatiles in microbial and mammalian short-term assays. Food Chem Toxicol. 37:813–823.
- Sun J. 2007. D-Limonene: safety and clinical applications. Altern Med Rev. 12:259–264.
- Trytek M, Fiedurek J, Skowronek M. 2009. Biotransformation of (R)-(+)-limonene by the psychrotrophic fungus Mortierella minutissima in H2O2-oxygenated culture. Food Technol Biotechnol. 47:131–136.
- Trytek M, Jędrzejewski K, Fiedurek J. 2014. Bioconversion of α-pinene by a novel cold-adapted fungus Chrysosporium pannorum. J Ind Microbiol Biotechnol. 42:181–188.
- Wargovich MJ. 2001. Herbals and cancer. Adv Exp Med Biol. 492:195–202.
- Wolski T, Hołderna-Kędzia E, Ludwiczuk A. 2001. Ocena składu chemicznego oraz aktywności przeciwdrobnoustrojowej olejków eterycznych i preparatów galenowych otrzymywanych z liści rozmarynu i szałwi lekarskiej. Postępy Fitoter. 4:6–11.
- Yang S-A, Jeon S-K, Lee E-J, Shim Ch-H, Lee I-S. 2010. Comparative study of the chemical composition and antioxidant activity of six essential oils and their components. Nat Prod Res. 24:140–151.
- Zienowicz M, Wisłowska A, Turzyńska D, Płaźnik A. 2003. Enancjomery i ich rola w psychofarmakologii na przykładzie escitalopramu. Farmakoter Psych Neurol. 4:71–83.