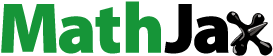
Abstract
Context Identification of bioactive components from complex natural product extracts can be a tedious process that aggravates the use of natural products in drug discovery campaigns.
Objective This study presents a new approach for screening antimicrobial potential of natural product extracts by employing a bioreporter assay amenable to HPLC-based activity profiling.
Materials and methods A library of 116 crude extracts was prepared from fungal culture filtrates by liquid–liquid extraction with ethyl acetate, lyophilised, and screened against Escherichia coli using TLC bioautography. Active extracts were studied further with a broth microdilution assay, which was, however, too insensitive for identifying the active microfractions after HPLC separation. Therefore, an assay based on bioluminescent E. coli K-12 (pTetLux1) strain was coupled with HPLC micro-fractionation.
Results Preliminary screening yielded six fungal extracts with potential antimicrobial activity. A crude extract from a culture filtrate of the wood-rotting fungus, Pycnoporus cinnabarinus (Jacq.) P. Karst. (Polyporaceae), was selected for evaluating the functionality of the bioreporter assay in HPLC-based activity profiling. In the bioreporter assay, the IC50 value for the crude extract was 0.10 mg/mL. By integrating the bioreporter assay with HPLC micro-fractionation, the antimicrobial activity was linked to LC-UV peak of a compound in the chromatogram of the extract. This compound was isolated and identified as a fungal pigment phlebiarubrone.
Discussion and conclusion HPLC-based activity profiling using the bioreporter-based approach is a valuable tool for identifying antimicrobial compound(s) from complex crude extracts, and offers improved sensitivity and speed compared with traditional antimicrobial assays, such as the turbidimetric measurement.
Introduction
Natural products have been a good resource for antimicrobial drug leads throughout the history (Cragg & Newman Citation2013). A high number of antimicrobial scaffolds originate in particular from moulds and other fungi (Aly et al. Citation2011). Both terrestrial and aquatic fungi are especially known to be highly biodiverse resources (Zhong & Xiao Citation2009). Estimate of existing fungal species is around 1.5 million; however, only 7% have been described and only a fraction of this number has been chemically investigated. Higher fungi produce a great variety of secondary metabolites, such as heterocyclines, polyketides, and terpenes, which differ structurally from plant metabolites. Commercial production of fungi is still limited due to the difficulties in cultivation, and especially in reproducibility of the yields of metabolites (Wasser Citation2011). About 5–10% of the known fungal species can be artificially cultured (Zhong & Xiao Citation2009). The most attractive option in the production of secondary metabolites is the submerged cultivation of fungal mycelia in liquid cultures.
Fungi have been used traditionally in Asia for medicinal purposes and they possess great potential for discovering new bioactive compounds (Dai et al. Citation2010; Aly et al. Citation2011). For instance, they have been shown to produce metabolites with antibacterial, antiviral, antifungal, antineoplastic, immune-stimulatory, and anti-inflammatory activities (Aly et al. Citation2011; Wasser Citation2011; Alves et al. Citation2012). Polyporales under the phyla Basidiomycota in particular have been reported to hold interesting antimicrobial activities but most of the studies were only conducted with crude extracts (Suay et al. Citation2000; Aqueveque et al. Citation2010; Alves et al. Citation2012). Traditional way of identifying active compounds from a bioactive crude extract is a tedious process usually requiring high amounts of starting material. This hampers the use of natural products, and in particular fungal extracts, in drug discovery campaigns.
Activity profiling of natural product extracts by coupling bioassays to HPLC-based fractionation can significantly accelerate the process. HPLC micro-fractionation can be combined with a variety of miniaturised bioassays on micro-plates (Potterat & Hamburger Citation2013), such as enzymatic (Oinonen et al. Citation2006), antiprotozoal (Hata et al. Citation2013), and cell-based (Tammela et al. Citation2004) assays. Only a limited number of cases have been published on antimicrobial field, e.g., a cell viability assay using MTT formazan and Bacillus subtilis as the target organism (Lang et al. Citation2006; Santiago et al. Citation2012). Another off-line approach to study the bioactivities of micro-fractions is to transfer the separated compounds on thin layer chromatographic (TLC) plates and use TLC bioautography for analysis, which has been applied successfully in antifungal studies (Queiroz et al. Citation2002; Favre-Godal et al. Citation2013). The localisation of antimicrobial compounds in extracts can be problematic due to the lack of sensitivity of the assays applied to the extract micro-fractions, particularly if the amount of the active compound in the extract is low.
Bioluminescence-based assays, utilizing different luciferase species, have become important tools for several research fields due to their high sensitive and dynamic range (Galluzzi & Karp Citation2006). A well-known example is Photinus pyralis (firefly) luciferase, which is widely used as a reporter gene in mammalian cell-based screening assays. Bacterial luciferases, such as Luxαβ from Photorhabdus luminescens, have gained less attention as screening tools despite their clear advantages over eukaryotic luciferases. If the whole bacterial operon (luxCDABE) is utilised in the set-up, the bioluminescence is autonomous and no addition of exogenous substrate is needed. Recently, antibacterial screening assays for identifying DNA gyrase inhibitors as well as translational and transcriptional inhibitors have been developed and utilized in screening campaigns (Moir et al. Citation2007; Nybond et al. Citation2013).
In this study, we employed a fast and sensitive antimicrobial assay based on a whole-cell bioreporter, Escherichia coli K-12 (pTetLux1) in a novel way by coupling the assay with HPLC micro-fractionation of a crude natural product extract. Following approaches were made to identify the active compound from little amount of Pycnoporus cinnabarinus (Jacq.) P. Karst. (Polyporaceae) crude extract: (i) HPLC micro-fractionation combined with the 120-min bioassay to localise the active principle in the crude extract; (ii) targeted isolation by HPLC; (iii) complete identification by UV, NMR, and MS spectroscopy; (iv) quantisation of the isolated compound in the crude extract.
Materials and methods
General experimental procedures
HPLC analysis was performed with an HPLC-UV (Perkin Elmer Inc., Norwalk, CT) (detected at 255 and 330 nm) coupled with a fraction collector (Gilson FC 204, Middleton, WI). NMR spectroscopic data were recorded on a 500 MHz Varian Inova spectrometer (Varian, Palo Alto, CA). Chemical shifts are reported in parts per million (δ) using the residual CDCl3 signal (δH 7.26; δC 77.2) as internal standards for 1H and 13C NMR, and coupling constants (J) are reported in Hz. Complete assignment was performed based on 2D experiments (COSY, edited-HSQC, and HMBC). HR-ESI-MS data were obtained on a Micromass LCT Premier time-of-flight mass spectrometer from Waters with an electrospray ionisation (ESI) interface (Waters, Milford, MA). Compound detection was performed in the positive-ion mode (PI) with an m/z range of 100–1000 Da and a scan time of 0.5 s in the W-mode. The MS was calibrated using sodium formate. Leucine enkephalin (Sigma-Aldrich, Steinheim, Germany) was used as an internal reference at 2 μg/mL and infused through a Lock Spray™ probe at a flow rate of 10 μL/min aided by a second LC pump.
Fungal liquid cultures and extraction
The studied fungi (Polyporaceae) were obtained from the Fungal Biotechnology Culture Collection (FBCC) maintained at the University of Helsinki, Department of Food and Environmental Sciences. Altogether 116 fungal strains representing 13 genera were studied in the primary screening. Pycnoporus cinnabarinus (Jacq.) P. Karst., strain FBCC130, has been collected from Finland by Professor A. Hatakka’s group (University of Helsinki, Department of Food and Environmental Sciences) and the identification was confirmed by Centraalbureau voor Schimmelcultures (CBS-KNAW), Utrecht, The Netherlands. The other fungi studied further after the primary screening were the following: Gloeophyllum trabeum (Pers.) Murrill (Gloeophyllaceae), strain FBCC 328; Phellinus ferrugineofuscus (P. Karst.) Bourdot & Galzin (Hymenochaetaceae), strain FBCC 945; Piptoporus betulinus (Bull.) P. Karst. (Fomitopsidaceae), strain FBCC 3; P. betulinus (Bull.) P. Karst., strain FBCC 10; and Trametes pubescens (Schumach.) Pilát (Polyporaceae), strain FBCC 592. The stock cultures are maintained on malt agar plates. Defined succinate-buffered (10 mM, pH 4.5, Sigma-Aldrich, St. Louis, MO) liquid medium with 0.49 mM ammonium nitrate (Merck, Darmstadt, Germany) and 0.52 mM l-asparagine (Sigma-Aldrich, St. Louis, MO) as nitrogen sources and glucose (0.5% w/v) as carbon source was used to grow the liquid cultures (Hatakka & Uusi-Rauva Citation1983). The total nitrogen concentration was 2 mM. The fungus was cultivated in 15 parallel Roux bottles, 200 mL medium/bottle. The bottles were inoculated with 10 agar pieces (∅ 7 mm) from actively growing malt agar plates, and incubated for 8 weeks at 28 °C. Mycelium was separated from the liquor by filtration (Miracloth, Merck Millipore, Darmstadt, Germany). pH of the liquor was measured and adjusted to 3 with HCl before liquid–liquid extraction with ethyl acetate (Rathburn, Walkerburn, Scotland). Ethyl acetate fractions were collected, combined, and evaporated to dryness using a rotavapor. Dried extract was re-dissolved in a small aliquot of ethyl acetate and transferred into a vial for lyophilisation.
HPLC-UV-based micro-fractionation of the crude extract
The ethyl acetate crude extract of the culture filtrate of P. cinnabarinus FBCC130 was micro-fractionated into 96-well plates using HPLC-UV (Perkin Elmer Inc., Norwalk, CT) coupled with a fraction collector (Gilson FC 204, Middleton, WI). The separation was carried out in a Zorbax SB-C18 column (150 × 4.6 mm i.d., 3.5 μm, Agilent Technologies, Santa Clara, CA). The gradient was performed at a flow rate of 1 mL/min with the following solvent system: water (0.02% HCOOH) (A) and acetonitrile (B) (Rathburn, Walkerburn, Scotland). The eluent profile was the following: 0–30 min linear gradient from 5 to 95% B; 30–35 min isocratic 95% B. The compound was detected at 255 and 280 nm. Injection volume was 20 μL (200 μg). The fractions from two runs were combined (each 0.15 min/well). The 96-well plates containing the micro-fractions were dried and submitted to the biological assay.
Isolation of the active compound
The ethyl acetate crude extract of the culture filtrate of P. cinnabarinus FBCC130 was suspended in water and partitioned with CHCl3 (Rathburn, Walkerburn, Scotland) to obtain enriched chloroform fraction. The chloroform fraction was dried and re-dissolved in acetonitrile (Rathburn, Walkerburn, Scotland). Compound 1 was isolated by HPLC-UV using a Luna RP18 column (250 × 4.6 mm i.d., 5 μm, Phenomenex, Torrance, CA). The mobile phase consisted of water (0.02% HCOOH) (A) and acetonitrile (B) (Rathburn, Walkerburn, Scotland). The eluent profile was the following: 0–30 min linear gradient from 5 to 95% B; 30–35 min isocratic 95% B, at flow rate 1 mL/min. The compound was detected at 255 and 330 nm. Injection volume was 60 μL. By using these procedures, 2 mg of compound 1 was isolated from 160 mg of the ethyl acetate crude extract of P. cinnabarinus FBCC130 culture filtrate. The purity of compound 1 was determined to be >98% on the basis of HPLC-UV and 1H NMR data.
Quantification of the active compound
The amount of compound 1 in the crude extract of the culture filtrate of P. cinnabarinus FBCC130 was quantified using HPLC-UV (detected at 330 nm) (Supplementary material). Five concentrations of the isolated compound 1 was utilized (Vinj 30 μL, 1–12 μg/inj., n = 3) and then plotted against the area of the peak to determine the calibration curve (y = 171 013x− 40 465, R2 = 0.9996). The peak area of compound 1 in the crude extract of P. cinnabarinus FBCC130 was determined (Vinj 30 μL, 120 μg/inj., n = 3), and then, the amount of the compound in the extract [% (w/w)] was calculated using the equation acquired for the isolated compound.
Antimicrobial assays on E. coli (ATCC 25922) strain
TLC bioautography
TLC bioautography of the fungal extracts was carried out in the preliminary screening phase to evaluate their antimicrobial activity. Overnight grown E. coli was diluted with the Muller–Hinton broth to 105 CFU/ml concentration. The suspension was further grown for 5 h to make sure that the bacteria were in the metabolically active growth phase to ensure good contrast on the plates. TLC plates (Kieselgel 60 F254, aluminium, 20 × 20 cm, Merck KGaA, Darmstadt, Germany) and eluent mixture (65:35:5) of methanol (Rathburn Chemicals Ltd, Walkerburn, Scotland), chloroform (Rathburn Chemicals Ltd, Walkerburn, Scotland) and 0.1% acetic acid (Riedel-de Haën, Seelze, Germany) in Milli-Q water (Millipore, Darmstadt, Germany) were used. The extracts (2 mg/mL in ethyl acetate) were applied on the plates with Linomat IV (Camag, Muttenz, Switzerland), 20 μL/band (40 μg/band). Positive control used was ampicillin 0.02 mg/mL in methanol, 20 μL/band. The eluent was evaporated for 1 h, and then the developed TLC plates were dipped into the bacteria suspension for 10 s and incubated for 3 h at 37 °C in a humified chamber. The plates were sprayed with a mixture of MTT (2.5 mg/mL, Sigma, St. Louis, MO) and Triton X-100 (0.1%, Sigma, St Louis, MD) in Milli-Q water (Waters, Milford, MA) and further incubated for 1 h in the same conditions to visualise the bacterial growth.
Broth microdilution assay
The antimicrobial activity of the ethyl acetate crude extracts of fungi was studied by using a broth microdilution assay based on E. coli (ATCC 25922) strain. Bacteria in the Muller–Hinton broth medium were plated at 5 × 105 CFU/ml with the extracts in DMSO. The final concentrations were 1 mg/mL for the extracts and 0.5% for DMSO. Antibacterial activity was evaluated by turbidimetric measurement at 620 nm (Varioskan Flash plate reader, Thermo Fisher Scientific, Vantaa, Finland) after 24 h incubation.
Antimicrobial assay on E. coli K-12 (pTetLux1) strain
The antimicrobial activity of the ethyl acetate crude extract of P. cinnabarinus FBCC130 was studied by using a screening assay based on recombinant, bioluminescent E. coli K-12 (pTetLux1) strain. The bacterial strain, the assay optimisation steps, and developed protocol for pure compounds have previously been described in Korpela et al. (Citation1998) and Nybond et al. (Citation2013). Briefly, the assay was carried out in white 96-well culture plates (CulturePlate-96, Perkin Elmer Inc., Norwalk, CT) in a total assay volume of 200 μL. Rifampicin, at a concentration 12.5 μg/mL, was used as a positive control. The extract and the positive control in DMSO were diluted into LB broth, yielding a final DMSO concentration of 0.5%. The DMSO tolerance of the assay has been previously investigated and based on the results, the ideal DMSO content should be ≤0.5% (Nybond et al. Citation2013). In the assay, maximum and background wells were compensated with the same DMSO content as the test wells. Bacteria in LB medium were plated at 5 × 105 CFU/well and luminescence was induced after pre-incubation for 30 min at 37 °C. The plate was further incubated for 90 min (37 °C) after which the luminescence was measured using a Varioskan Flash plate reader (Thermo Fisher Scientific, Vantaa, Finland). The protocol was slightly modified for the fraction screening: the extract was pre-fractionated into 96-well, evaporated to dryness in a lyophilisator, then DMSO (1 μL/well) and LB broth (100 μL/well) were added to dissolve the fractions. Then, bacteria suspension was plated, and the protocol was continued as described above.
Ampicillin sodium (AppliChem, Darmstadt, Germany) was used as selective agent in overnight cultures and tetracycline hydrochloride (CalBiochem, La Jolla, CA) as luminescence inducer at a sublethal concentration of 100 ng/mL. Stocks were prepared in Milli-Q water (Waters, Milford, MA) and sterilised through 0.2 μm syringe filters (VWR International, San Diego, CA). The positive reference antibiotic rifampicin (purity ≥ 97%, Sigma-Aldrich, St. Louis, MO) was dissolved in DMSO. The inhibition-% of rifampicin at a concentration of 10 μM was 98%. The antibiotic stocks were stored at −20 °C.
Data analysis
The inhibition percentages in the bioluminescence assay were calculated with the following formula, where Ltest is the luminescence from test wells, while Lmin corresponds to the background luminescence from non-induced wells and Lmax is the maximum luminescence value obtained from control wells:
The assay quality parameters (Z′, S/N, S/B) were calculated based on the average luminescence values and standard deviations from the maximum (induced bacteria) and background (non-induced) controls, each control was included in triplicate within the assay plates. The formulae used for the parameter calculations are presented below, originally reported by Zhang et al. (Citation1999) and Bollini et al. (Citation2002)
The IC50 value of the fungal extract was determined by fitting the results into dose–response curves in Origin software (OriginLab Corp., Northampton, MA). A hit limit for fraction plates was defined as average-3SDs.
Results and discussion
Coupling of HPLC micro-fractionation of a crude extract with a cell-based E. coli K-12 (pTetLux1) bioreporter assay was employed in this study to accelerate the process of identifying bioactive component(s) from extracts. The ethyl acetate crude extract of culture filtrates of P. cinnabarinus (strain FBCC103) was selected to represent an example of a crude extract, which had been identified to demonstrate interesting antimicrobial activity in the preliminary screening campaign on TLC bioautography () and in the follow-up assays conducted on the six most interesting extracts (). The discovery of bioactive compounds from natural products can be challenging due to the fact that the active metabolite can be present in very small amounts. Using traditional broth dilution antibacterial assay based on turbidity measurement, only modest growth inhibition of E. coli (ATCC 25922) by the crude extract of P. cinnabarinus could be detected (14.4 ± 2.0%) () due to the insensitivity of the assay. The inhibition of E. coli K-12 (pTetLux1) by the same crude extract was 98.1 ± 0.6%. In the broth microdilution method, the bacterial growth is detected by the turbidity of the microbial mass and needs to be quite high to achieve a good signal separation between the background and the maximum well in order to properly evaluate the activity of the samples. Therefore, within the first hours of bacterial growth, the signal window is not good enough to detect any activity. In most cases, the endpoint measurement is set after 24 h of growth. The natural product fractions might contain interesting active metabolites, but are present in such low concentrations that the bioactivity is missed using the standard methods. Thus, in this work, the aim was to overcome the issue by using a bioreporter-based assay. The crude extract showed a dose-dependent response in the E. coli K-12 (pTetLux1) bioreporter assay with an IC50 value of 0.10 mg/mL (Supporting information Figure S1). The extract was micro-fractionated by HPLC-UV in order to localise the compound responsible for the bioactivity. The micro-fractions were collected to a micro-plate, evaporated to dryness and the 120 min bioassay was subsequently carried out. Using this approach, it was possible to correlate the inhibitory activity to one peak (compound 1) in the chromatogram, displaying activity above the set hit limit of 26% (average-3SDs) (). The hit limit in screening assays is usually set at 3SDs away from the mean of the sample signals to gain 99.73% confidence limit to the sample data (Zhang et al. Citation1999).
Figure 1. (A) HPLC-UV chromatogram of the ethyl acetate crude extract of P. cinnabarinus FBCC130 at 255 nm. (B) Biological activity of the HPLC micro-fractions against the bioluminescent E. coli K-12 (pTetLux1) strain. The activity in the micro-fractionated P. cinnabarinus FBCC130 extract was associated with one peak (1) in the chromatogram. Each dark grey column represents a well on a 96-well plate. The hit limit (26%), defined as average-3SDs, is shown in light grey. Statistical parameters for the assay plate were Z′ = 0.92, S/N = 40.16, and S/B = 62.18.
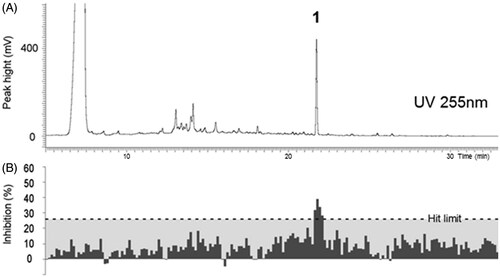
Table 1. Preliminary screening results for fungal extracts against E. coli (ATCC 25922) on TLC bioautography.
Table 2. The fungal extracts selected for a follow-up screening campaign, their activities on TLC bioautography against E. coli (ATCC 25922) and in 96-well plate assays against E. coli (ATCC 25922) and E. coli K-12 (pTetLux1).
Using the HPLC-based activity profile, a targeted isolation of compound 1 was performed using HPLC-UV with a larger reverse phase analytical column (250 × 4.6 mm, I.D.) and multiple injections. Compound 1 was isolated as a red amorphous powder. The high-resolution (HR)-ESI-MS displayed an [M + H]+ ion at m/z 305.0808, which is in agreement with the molecular formula C19H13O4 (calcd for C19H13O4, 305.0814, Δppm = 2). The 1H NMR of compound 1 showed a singlet at δH 6.14 characteristic for a methylene dioxy group based on the edited-HSQC (δC 102.7). The integration ratio between this methylene and the aromatic signals at δH 7.63 (4H, d, J = 7.4 Hz, H-2′,6′), 7.45 (4H, t, J = 7.4 Hz, H-5′), and 7.39 (2H, t, J = 7.4 Hz, H-4′) indicated the presence of two equivalent mono-substituted phenyl groups. The 3JCH HMBC correlations between the methylene group and the carbon at δC 156.2 (C-1) and between the phenyl group (H-2′,3′) and the carbon at δC 113.9 (C-2) suggested the presence of a central symmetrical unsaturated ring. The combination of these data with the molecular formula allowed the identification of compound 1 as phlebiarubrone (), previously isolated from the culture of the fungus Phlebia strigosozonata (Schwein.) Lloyd (Corticiaceae) (syn. Punctularia strigosozonata) (McMorris & Anchel Citation1963, Citation1967; Hayakawa et al. Citation2008). The NMR data were in good agreement with those described in the previous reports.
The amount of compound 1 in the ethyl acetate crude extract of P. cinnabarinus (strain FBCC103) was quantified using HPLC-UV (330 nm) (Supporting information, Table S1). Linear regression of the amount (μg/inj.) against the peak area showed an excellent correlation (R2 = 0.9996) (Supporting information, Figure S2). The extract was estimated to contain 2.4% (w/w) of phlebiarubrone, and since it was the only active principle, its approximated IC50 value would be 8 μM. A direct dose–response study was not possible because of the very restricted amount of compound obtained. Basidiomycetous fungi are known to produce antimicrobial metabolites (Alves et al. Citation2012), which was also shown in the preliminary screening campaign as numerous detected activities (). The antibacterial properties of phlebiarubrone, isolated from Punctularia atropurpurascens (Berk. & Broome) Petch (Corticiaceae), was previously studied by Anke et al. (Citation1984), for example, against Acinetobacter and Bacillus species [minimum inhibitory concentrations (MIC) in the range of 10–60 μg/mL]. Pycnoporus cinnabarinus is known as a medicinal mushroom in Nepal (Jha et al. Citation2011). Maceration of fruiting bodies in mustard oil produces a red-coloured ointment used for ear infection in children and for wound healing. Culture filtrate of P. cinnabarinus has been shown to inhibit growth of E. coli, S. aureus, and Pseudomonas aeruginosa in broth microdilution and agar diffusion assays (Imtiaj & Lee Citation2007), but the reported biological activities found in P. cinnabarinus have not been linked to specific active principles.
As described above, this study demonstrates the compatibility of the bioreporter-based assay with the HPLC micro-fractionation strategy for a rapid detection and identification of the active compounds present in complex matrices such as fungal extracts. In addition, the luciferase genes in E. coli (pTetLux1) are under strict transcriptional control of the tetA promoter ensuring low level of background bioluminescence in the assay (Nybond et al. Citation2013), which was also the case in our experiments shown as excellent statistical parameters (Z′ > 0.9, S/N > 40, S/B > 30) (see figure legends in and S1). It has also been demonstrated that transcriptional, translational, and DNA replication inhibitors display strong inhibitory effects (IC50 values < 10 μM) in the assay, whereas agents affecting the cell wall synthesis are in general inactive (Nybond et al. Citation2013). Because phlebiarubrone displayed an effect comparable with transcriptional, translational, and DNA replication inhibitors in this assay, this may suggest that its mode of action could be related to inhibition of protein synthesis or DNA replication. However, since the assay is cell-based, the effect may as well be generally biocidal and non-specific, and thus more detailed studies would be required to delineate the mode of action.
The number of natural products included in drug discovery programs decreased in the past decades when automated high throughput platforms were introduced due to the compatibility problems of complex extracts with bioassays, and laborious identification processes of the active principles following the activity profiling. In this context, HPLC-based activity profiling (Cesari et al. Citation2013; Queiroz et al. Citation2014) which can further be coupled with hyphenated spectroscopic methods for dereplication (Queiroz et al. Citation2002, Citation2005) provides a valuable tool for drug discovery of bioactive compounds from complex natural extracts. On the contrary, the field of antimicrobial drug discovery has lacked sensitive assays compatible with automated environments. Traditional assays, such as measurement of turbidity, require 20–24 h incubation times, and more importantly, are too insensitive to detect activities at low concentrations.
Conclusions
The bioreporter assay described in this work showed its sensitivity for detecting antimicrobial compounds in HPLC micro-fractions, which have low concentrations. Further, the assay required only 120 min incubation time and was compatible with automated environment, which increases the throughput of the discovery process. In conclusion, our approach to use the sensitive bioreporter assay with HPLC-based activity profiling expedites and enhances the discovery of new antimicrobial compounds from complex natural extracts.
Declaration of interest
The authors report that they have no conflicts of interest. This work was financially supported by personal grants from the Finnish Cultural Foundation (P. J., S. N., and M. K.) and the Swedish Cultural Foundation in Finland (S. N.) as well as by the Research Funds of University of Helsinki (S. N. and P. T.).
Supplementary material available online Supplementary Table S1 Supplementary Figure S1 and S2
Acknowledgements
Karoliina Sainio is acknowledged for conducting the preliminary screening of the extracts.
References
- Alves MJ, Ferreira IC, Dias J, Teixeira V, Martins A, Pintado M. 2012. A review on antimicrobial activity of mushroom (Basidiomycetes) extracts and isolated compounds. Planta Med. 78: 1707–1718.
- Aly AH, Debbab A, Proksch P. 2011. Fifty years of drug discovery from fungi. Fungal Divers. 50:3–19.
- Anke H. 1984. Neue terphenylchinone aus mycelkulturen von Punctularia atropurpurascens (Basidiomycetes). Z Naturforsch. 39c:695–698.
- Aqueveque P, Anke T, Saez K, Silva M, Becerra J. 2010. Antimicrobial activity of submerged cultures of Chilean basidiomycetes. Planta Med. 76:1787–1791.
- Bollini S, Herbst JJ, Gaughan GT, Verdoorn TA, Ditta J, Dubowchik GM, Vinitsky A. 2002. High-throughput fluorescence polarization method for identification of FKBP12 ligands. J Biomol Screen. 7:526–530.
- Cesari I, Queiroz EF, Favre-Godal Q, Marcourt L, Caccialanza G, Moundipa PF, Brusotti G, Wolfender JL. 2013. Extensive phytochemical investigation of the polar constituents of Diospyros bipindensis Gürke traditionally used by Baka pygmies. Phytochemistry 96:279–287.
- Cragg GM, Newman DJ. 2013. Natural products: a continuing source of novel drug leads. Biochim Biophys Acta 1830:3670–3695.
- Dai YC, Zhou LW, Cui BK, Chen YQ, Decock C. 2010. Current advances in Phellinus sensu lato: medicinal species, functions, metabolites and mechanisms. Appl Microbiol Biotechnol. 87:1587–1593.
- Favre-Godal Q, Queiroz EF, Wolfender JL. 2013. Latest developments in assessing antifungal activity using TLC-bioautography: a review. J AOAC Int. 96:1175–1188.
- Galluzzi L, Karp M. 2006. Whole cell strategies based on lux genes for high throughput applications toward new antimicrobials. Comb Chem High Throughput Screen. 9:501–514.
- Hata Y, Raith M, Ebrahimi SN, Zimmermann S, Mokoka T, Naidoo D, Fouche G, Maharaj V, Kaiser M, Brun R, et al. 2013. Antiprotozoal isoflavan quinones from Abrus precatorius ssp. africanus. Planta Med. 79:492–498.
- Hatakka AI, Uusi-Rauva AK. 1983. Degradation of 14C-labelled poplar wood lignin by selected white-rot fungi. Eur J Appl Microbiol Biotechnol. 17:235–242.
- Hayakawa I, Watanabe H, Kigoshi H. 2008. Synthesis of ustalic acid, an inhibitor of Na+, K+-ATPase. Tetrahedron 64:5873–5877.
- Imtiaj A, Lee TS. 2007. Screening of antibacterial and antifungal activities from Korean wild mushrooms. World J Agric Sci. 3:316–321.
- Jha SK, Kumar N, Tripathi NN. 2011. Survey of ethnomedicinal macrofungi of Nagarjun and Phulchowki areas of Kathmandu valley Nepal. Int J Pharm Sci Rev Res. 11:147–151.
- Korpela MT, Kurittu JS, Karvinen JT, Karp MT. 1998. A recombinant Escherichia coli sensor strain for the detection of tetracyclines. Anal Chem. 70:4457–4462.
- Lang G, Mitova MI, Ellis G, van der Sar S, Phipps RK, Blunt JW, Cummings NJ, Cole AL, Munro MH. 2006. Bioactivity profiling using HPLC/microtiter-plate analysis: application to a New Zealand marine alga-derived fungus, Gliocladium sp. J Nat Prod. 69:621–624.
- McMorris TC, Anchel M. 1963. Phlebiarubrone, a basidiomycete pigment related to polyporic acid. Tetrahedron Lett. 4:335–337.
- McMorris TC, Anchel M. 1967. The structure of the basidiomycete ortho quinone, phlebiarubrone, and of its novel acylation product. Tetrahedron 23:3985–3991.
- Moir DT, Ming D, Opperman T, Schweizer HP, Bowlin TL. 2007. A high-throughput, homogeneous, bioluminescent assay for Pseudomonas aeruginosa gyrase inhibitors and other DNA-damaging agents. J Biomol Screen. 12:855–864.
- Nybond S, Karp M, Tammela P. 2013. Antimicrobial assay optimization and validation for HTS in 384-well format using a bioluminescent E. coli K-12 strain. Eur J Pharm Sci. 49:782–789.
- Oinonen PP, Jokela JK, Hatakka AI, Vuorela PM. 2006. Linarin, a selective acetylcholinesterase inhibitor from Mentha arvensis. Fitoterapia 77:429–434.
- Potterat O, Hamburger M. 2013. Concepts and technologies for tracking bioactive compounds in natural product extracts: generation of libraries, and hyphenation of analytical processes with bioassays. Nat Prod Rep. 30:546–564.
- Queiroz EF, Ioset JR, Ndjoko K, Guntern A, Foggin CM, Hostettmann K. 2005. On-line identification of the bioactive compounds from Blumea gariepina by HPLC-UV-MS and HPLC-UV-NMR, combined with HPLC-micro-fractionation. Phytochem Anal. 16:166–174.
- Queiroz EF, Wolfender JL, Atindehou KK, Traore D, Hostettmann K. 2002. On-line identification of the antifungal constituents of Erythrina vogelii by liquid chromatography with tandem mass spectrometry, ultraviolet absorbance detection and nuclear magnetic resonance spectrometry combined with liquid chromatographic micro-fractionation. J Chromatogr A 974:123–134.
- Queiroz MM, Queiroz EF, Zeraik ML, Ebrahimi SN, Marcourt L, Cuendet M, Castro-Gamboa I, Hamburger M, da Silva Bolzani V, Wolfender JL. 2014. Chemical composition of the bark of Tetrapterys mucronata and identification of acetylcholinesterase inhibitory constituents. J Nat Prod. 77:650–656.
- Santiago C, Fitchett C, Munro MH, Jalil J, Santhanam J. 2012. Cytotoxic and antifungal activities of 5-hydroxyramulosin, a compound produced by an endophytic fungus isolated from Cinnamomum mollisimum. Evid Based Complement Alternat Med. 2012:689310.
- Suay I, Arenal F, Asensio FJ, Basilio A, Cabello MA, Diez MT, Garcia JB, del Val AG, Gorrochategui J, Hernandez P, et al. 2000. Screening of basidiomycetes for antimicrobial activities. Antonie Van Leeuwenhoek 78:129–139.
- Tammela P, Wennberg T, Vuorela H, Vuorela P. 2004. HPLC micro-fractionation coupled to a cell-based assay for automated on-line primary screening of calcium antagonistic components in plant extracts. Anal Bioanal Chem. 380:614–618.
- Wasser SP. 2011. Current findings, future trends, and unsolved problems in studies of medicinal mushrooms. Appl Microbiol Biotechnol. 89:1323–1332.
- Zhang JH, Chung TD, Oldenburg KR. 1999. A simple statistical parameter for use in evaluation and validation of high throughput screening assays. J Biomol Screen.4:67–73.
- Zhong JJ, Xiao JH. 2009. Secondary metabolites from higher fungi: discovery, bioactivity, and bioproduction. In: Scheper T, editor. Advances in biochemical engineering/biotechnology, vol. 113. Berlin: Springer-Verlag. p. 79–150.