Abstract
Context C-6-Geranylated flavonoids possess promising biological activities. These substances could be a source of lead compounds for the development of therapeutics.
Objective The study was designed to evaluate their antibacterial and antileishmanial activity.
Materials and methods C-6-Geranylated flavanones were tested in micromolar concentrations against promastigote forms of Leishmania brazilensis, L. donovani, L. infantum, and L. panamensis against methicillin-resistant Staphylococcus aureus (MRSA); and synergistic potential with antibiotics was analyzed. IC50 values (after 72 h) were calculated and compared with that of miltefosine. Flow cytometry and DNA fragmentation analysis were used the mechanism of the effect. Geranylated flavanones or epigallocatechin gallate were combined with oxacillin, tetracycline, and ciprofloxacin, and the effects of these two-component combinations were evaluated. Minimal inhibitory concentrations (MICs) and minimal bactericidal concentrations (MBCs) were established (after 24 h), the synergy was measured by the checkerboard titration technique, and the sums of the fractional inhibitory concentrations (∑FICs) were computed.
Results 3′-O-Methyl-5′-O-methyldiplacone and 3′-O-methyldiplacone showed good antileishmanial activities (IC50 8–42 μM). 3′-O-Methyl-5′-hydroxydiplacone activates the apoptotic death at leishmanias, the effect of 3′-O-methyl-5′-O-methyldiplacone has another mechanism. The test of the antibacterial activity showed good effects of 3′-O-methyldiplacol and mimulone against MRSA (MIC 2–16 μg/mL), and in six cases, the results showed synergistic effects when combined with oxacillin. Synergistic effects were also found for the combination of epigallocatechin gallate with tetracycline or oxacillin.
Conclusion This work demonstrates anti-MRSA and antileishmanial potential of geranylated flavanones and uncovers their promising synergistic activities with antibiotics. In addition, the mechanism of antileishmanial effect is proposed.
Keywords:
Introduction
Pathogens that have become resistant to antibiotics, represent a critical threat in many hospitals and in communities around the world, and the development of drugs that can combat such infections is urgently needed (Alanis Citation2005; French Citation2010). Staphylococci are the most common causes of life-threatening diseases such as hemodialysis-related bacteraemia, endocarditis, peritonitis, pyelonephritis, sepsis, and toxic shock syndrome (Casey et al. Citation2007). Strains of the methicillin-resistant Staphylococcus aureus (MRSA) are especially virulent agents. Most of them are cross-resistant to all β-lactam antibiotics, tetracyclines and macrolides or azalides, but they are generally relatively susceptible to other antibiotics (Andremont et al. Citation2011). Their resistance to other classes of antibiotics is however growing rapidly (Bassetti et al. Citation2009). One strategy for combating infectious diseases takes account of the fact that many diseases can have multiple causes and can be treated most effectively by combining natural drugs with commonly used antibiotics (Wagner & Ulrich-Merzenich Citation2009).
Leishmaniasis is a severe disease most commonly spread in Africa, Asia, and Latin America, and threatening about 350 million people worldwide. The genus Leishmania, a protozoan of the Hemoflagellatae group, is responsible for leishmaniasis. The rather small number of drugs currently available in the clinics makes the advent of drug resistance a problem in the treatment of leishmaniasis, and a search for new antileishmanial remedies is therefore needed (Rocha et al. Citation2005).
Some phenolic compounds are believed to be the promising agents for the treatment of some bacterial infections. They have shown strong ability to bind to different proteins and great affinity for cell membranes (Wagner & Ulrich-Merzenich Citation2009). Combinations of tetracycline or β-lactam antibiotics with baicalein (5,6,7-trihydroxyflavone) exhibit synergistic effects against MRSA (Fujita et al. Citation2005). The synergy of combinations of another flavonoid, taxifolin-7-O-α-rhamnopyranoside, with ampicillin, levofloxacin, ceftazidime or azithromycin against 10 clinical isolates of MRSA has also been evaluated (An et al. Citation2011). Recently, epigallocatechin gallate has been studied extensively. It has been reported that it is synergistically active in combination with β-lactams, tetracycline (TTC) (Abreu et al. Citation2012) or oxytetracycline against various strains of methicillin and tetracycline-resistant S. aureus (Nový et al. Citation2013). Our previous research showed a promising potential of geranylated flavanones to inhibit growth of MRSA (Navrátilová et al. Citation2013).
Plant products with antileishmanial activity have been reviewed by Rocha et al. (Citation2005). More recently, some plant-derived flavonoids have been shown to exert antileishmanial activity. Quercetin has shown selective activity against leishmanial topoisomerase (Jean-Moreno et al. Citation2006; Cortázar et al. Citation2007). Some quercetin and kaempferol glycosides have been found to be active against Leishmania spp. (Muzitano et al. Citation2006). Luteolin has also been reported to induce cell death in both the promastigote and amastigote forms of Leishmania (Sen et al. Citation2006). Prenylated flavonoids isolated from Cannabis sativa have displayed promising activity against L. donovani promastigotes (Radwan et al. Citation2008a,Citationb). Prenylated isoflavonoids obtained from Psorothamnus arborescens were found to have higher activity than their non-prenylated analogs (Salem & Werbovetz Citation2006). A prenylated flavonoid obtained from Lonchocarpus spp. affected different Leishmania spp. (Borges-Argáez et al. Citation2007). Lipophilic polymethoxylated flavonoids isolated from Ageratum conyzoides were active at micromolar concentrations (Nour et al. Citation2010).
Geranylated flavonoids seem to be promising compounds due to the presence of terpenoid chain and a phenolic part. Therefore, we tested the activity of C-6 geranyl flavonoids against four leishmanias: L. brazilensis, L. donovani, L. infantum, and L. panamensis, as putative antileishmanial agents, and they showed activity comparable with the standard used. Based on the previous research, we also decided to confirm the hypothesis that antibacterially active 3′-O-methyldiplacol (3) and mimulone (5) obtained from Paulownia tomentosa Thunb. Sieb. & Zucc. ex Steud. (Navrátilová et al. Citation2013) should show synergistic effects when combined with oxacillin (OXA), TTC or ciprofloxacin (CIP). Positive results of the screening for the antibacterial activity of geranylated flavonoids from P. tomentosa against different types of strains of MRSA also led us to this idea. Based on previous results, epigallocatecin gallate (EGCG, 7) was used as a positive control and to confirm its potential to act synergically with antibiotics.
Materials and methods
Chemicals
3′-O-Methyl-5′-hydroxydiplacone (1), 3′-O-methyl-5′-O-methyldiplacone (2), 3′-O-methyldiplacol (3), 3′-O-methyldiplacone (4), mimulone (5) and diplacone (6) () were isolated from P. tomentosa fruits and identified as described in a previous report (Šmejkal et al. Citation2008). A voucher specimen (PT-04O) has been deposited at the herbarium of the Department of Natural Drugs, Faculty of Pharmacy, University of Veterinary and Pharmaceutical Sciences Brno (UVPS Brno), Brno, Czech Republic. The compounds are saved in the compound library of the department. The purity of isolated compounds exceeded 95% according to HPLC analysis. Compounds 1–6 and epigallocatechin gallate (EGCG, 7, Sigma-Aldrich, Praha, Česká republika) were dissolved in dimethyl sulphoxide (DMSO) (Sigma-Aldrich, Praha, Česká republika) for antibacterial assays. For antileishmanial assays, compounds 1–6 were dissolved in 40% DMSO with 10% 0.1 M NaOH and used to prepare stock solutions of concentrations 0.025, 0.25, 0.625, 1.25, 2.5, 5, and 12.5 mM. In our experiments, the final concentration of DMSO in the culture medium never exceeded 0.2%, which did not affect the viability of the parasitic cells as was confirmed by cultivation with DMSO only. Deionised water and 96% ethanol were used to dissolve the standard antibiotics oxacillin (OXA), tetracycline (TTC) and ciprofloxacin (CIP) (Sigma-Aldrich, Praha, Česká republika). Bacteria were grown in the Mueller–Hinton broth (MHB) and Mueller–Hinton agar (MHA) (Oxoid, Hampshire, UK). MHB was used for susceptibility testing. MBCs were determined using MHA plates.
Bacterial strains
Eleven strains of staphylococcus were used for the tests. Staphylococcus intermedius MRSI 82110 was obtained during surgery on a dog (on deposit at the Department of Infectious Diseases and Microbiology, UVPS Brno). Four methicillin-resistant strains of S. aureus: MRSA 67755, MRSA 63718, MRSA 62097, and MRSA 62059 are deposited at the Department of Infectious Diseases and Microbiology, UVPS Brno; MRSA 1098, MRSA 1679, and MRSA 1903 were obtained from the National Institute of Public Health Brno. MRSA strains, MRSA 630, MRSA 3202, and MRSA 6975 are clinical isolates deposited at the National Reference Laboratory for Antibiotics, National Institute of Public Health Prague.
Parasite cultivation
The slightly modified method of Varela-M et al. (Citation2012) was used for the cultivation and further work with leishmanias. Promastigotes of four species, L. braziliensis (MHOM/CO/88/UA301), L. donovani (MHOM/IN/80/DD8), L. infantum (MCAN/ES/96/BCN150), and L. panamensis (MHOM/CO/87/UA140), were continually cultured at 27 °C by shaking in Schneier’s medium (Sigma-Aldrich, Madrid, Spain) supplemented with NaHCO3 (0.4 g/L), CaCl2 (0.6 g/L), 10% fetal bovine serum (Sigma-Aldrich, Spain), and penicillin 10 000 U/mL–streptomycin 10 000 μg/mL (Sigma-Aldrich, Madrid, Spain). Parasites were transferred to a fresh medium every 2–4 d; their viability was checked periodically under a microscope. Parasites in the exponential growth phase were centrifuged at 2500 rpm for 10 min, re-suspended in a fresh medium and divided onto 96-well test plates in the amount of 105 per 200 μL with DMSO as a control or with the tested compound at final concentrations from 0.25 to 125 μM, incubated for 72 h at 27 °C with shaking, and analysed by XTT assay, or incubated for 24 or 48 h under the same conditions in the amount of 2 × 106 cells/mL for flow cytometry evaluation.
Cytotoxicity assay
Prior to testing of the selected C-geranylated flavanones on leishmania promastigotes, their effect against native rat alveolar macrophages using the MTT method was tested (Nešuta et al. Citation2011).
XTT assay
The cell viability was evaluated by measuring the activity of mitochondrial dehydrogenase. XTT reagent (50 μL) activated with PMS (at a ratio 49:1, v/v) was added and incubated at 27 °C for 4 or 12 h and the absorbance of the coloured formazan formed was measured at 490 nm (with 655 nm as a reference). The IC50 value was then calculated. All samples were measured in independent triplicates.
Flow cytometry measurement
For the analysis of a cell cycle, we used flow cytometry. Promastigote cells of L. donovani treated for 24 or 48 h with compounds 1 and 2 at concentrations of 12.5 and 25 μM, respectively, were centrifuged and denatured with ethanol. RNAse was added, and propidium iodide was used as a fluorescent marker of DNA. After 1 h incubation in darkness at laboratory temperature, the fragmentations of the nuclei were measured on a fluorescence-activated cell sorting (FACS) Calibur flow cytometer (Becton Dickinson, San Jose, CA) equipped with a 488 nm argon laser, using CELLQUEST software. The received data were analysed using WinMDI 2.8 (Biosciences PharMingen, San Diego, CA) and expressed as a percentage of cells in apoptosis.
Susceptibility testing
MIC and MBC values were determined by the broth microdilution method in accordance with the guidelines of the Clinical and Laboratory Standards Institute (CLSI Citation2009), using 96-well microtiter plates. Bacterial suspension was prepared from 24 h old bacterial cultures and adjusted to 0.5 McFarland standard using Densi-La-Meter (Lachema, Brno-Řečkovice, Česká republika), and subsequently diluted with sterile distilled water to get the final concentration of 5 × 106 CFU/mL. Serial two-fold dilutions of the compounds tested were inoculated with a suspension of bacteria and incubated at 37 °C for 24 h. MIC values were defined as the lowest concentrations of an antimicrobial substance that inhibited the visible growth of a micro-organism. MBC values were determined by transferring 10 μL aliquots from wells without visible signs of growth into MHA plates and incubated at 37 °C for a further 24 h. MBC values were the lowest concentrations of an antibacterial agent resulting in the growth of not more than two CFU. All tests were carried out in duplicate in each of the two independent experiments. MIC and MBC values were calculated as an average from all tests (data not shown.)
Synergy testing
Potential anti-MRSA synergistic effects of compounds 3 and 5 with OXA, TTC, and CIP were measured by the checkerboard titration technique, and the fractional inhibitory concentrations (FICs) were evaluated according to the guidelines set by the European Committee on Antimicrobial Susceptibility Testing (EUCAST Citation2000). The FIC results were interpreted as follows: ∑FIC ≤ 0.5 = synergy; ∑FIC > 0.5 ≤ 1 = additivity; ∑FIC > 1 to < 2 = indifference; ∑FIC ≥ 2 = antagonism.
Results and discussion
The C-6 geranylated compounds 3′-O-methyl-5′-hydroxydiplacone (1), 3′-O-methyl-5′-O-methyldiplacone (2), 3′-O-methyldiplacol (3), 3′-O-methyldiplacone (4), mimulone (5), and diplacone (6), isolated from P. tomentosa as described previously (Šmejkal et al. Citation2008), were tested for their ability to kill the promastigote forms of four Leishmania species: L. braziliensis, L. donovani, L. infantum and L. panamensis. Prior to testing, the cytotoxicity of compounds 1–6 was evaluated using alveolar rat macrophages (Nešuta et al. Citation2011). Compounds tested showed no substantial toxicity at concentrations tested. By using XTT assays, we found that compounds 2 and 4 achieved significant antileishmanial activity, showing IC50 values against L. donovani and L. braziliensis comparable with that of miltefosine, which was used as a positive control (). These compounds also showed notable activity against L. infantum and L. panamensis, but at higher concentrations than miltefosine. As can be seen, the above-mentioned compounds (2 and 4) showed some structure–activity relation specifity in their toxic effect against certain Leishmania species. The most active compounds tested here were 3′-O-methyl substituted at the flavanone ring B. The activity of 3′-O-methyl substituted dihydroflavonol 3 was diminished probably due to the presence of 3-OH substitution.
Table 1. Leishmania species were treated 72 h with concentrations ranging from 0.25 to 125 μM of compounds 1–6 obtained from Paulownia tomentosa and with miltefosine as a control.
C-6-Substituted compounds isolated from C. sativa such as cannflavin C are structurally very similar to compound 4 (except for the double bond between C-2 and C-3), and they have been reported to show an IC50 value of 4.5 μg/mL (cannflavin C, close to 10 μM) against L. donovani (Radwan et al. Citation2008a). Antileishmanial activity for cannflavin B (the double bond between C-2 and C-3, C-6 prenyl, 5 μM) has also been observed (Radwan et al. Citation2008b). Radwan et al. (Citation2008a) also reported a moderate activity of cannflavin A (17 μg/mL, 39 μM). Salem et al. (Citation2011) have published some data about the antileishmanial activity of the C-geranyl flavonoids that are in good agreement with the data presented here. Thus, we suggest that the presence of a prenyl side chain at C-6 could be an important structural feature for antileishmanial activity. For example, highly methoxylated lipophylic flavonoids obtained from Ageratum conyzoides have shown an effect against L. donovani (Nour et al. Citation2010). As could be deduced from the literature and our results, a higher degree of lipophility and better entrance to the parasite cell could play important roles.
For the next stage of the evaluation of the cell cycle, compounds 1 and 2 were chosen based on the XTT assay on L. donovani. The analysis of flow cytometry experimental data brought us a result showing a high percentage of DNA fragmentation after treatment of the parasitic cells with 3′-O-methyl-5′-hydroxydiplacone (1), which hints at the probable mechanism of effect by induction of apoptotic death (). On the other hand, almost no DNA fragmentation after treatment of L. donovani promastigote cells with 3′-O-methyl-5′-O-methyldiplacone (2) has been observed. Comparing these results with the results obtained by XTT assay, it is obvious that the death of parasitic cells is caused in a non-apoptic way in the case of 3′-O-methyl-5′-O-methyldiplacone (2) and that the presence of the additional methoxy group at the ring B of the flavanone significantly changes the mechanism of action. Galzi et al. (Citation2010) showed a connection between anti-inflammatory activity and the antileishmanial effect. The programmed cell death is closely connected to the protozoan mechanism of killing action (Bruchhaus et al. Citation2007). Our other experiments testing the anti-inflammatory activity of C-geranylated flavanones showed their ability to affect gene expression and protein synthesis in cellular model (Hošek et al. Citation2010) and an effect on the cell-cycle mediated by changes in the cyclins (Kollár et al. Citation2011), which confirms the ability of compounds 1 and 2 affect cell cycle and which support the connection of anti-inflammatory activity and the antileishmanial effect.
Figure 2. Apoptogenic effect of compounds 1 and 2 on L. donovani promastogote cells. Percentage of cells in apoptosis calculated from flow cytometry data. Experiments were run in independent duplicates.
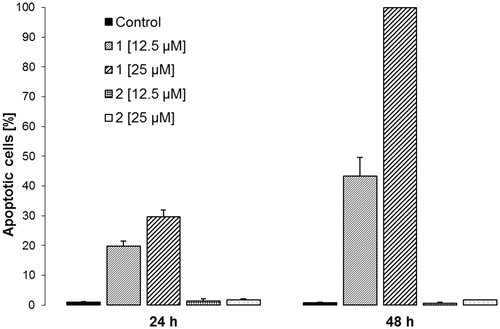
In our previous research, the antibacterial activity of different geranylated flavonoids against five MRSA strains (287, 4211, 6975, 630, and 62059) was shown (Navrátilová et al. Citation2013), where the most active compound 5 showed MIC values ranging from 2 to 4 μg/mL (4.9–8.8 μM), followed by compound 3 with MIC values ranging from 4 to 8 μg/mL (8.8–17.6 μM) towards all strains tested. To support the results of our research, MIC values against the other six different strains of MRSA for compounds 1–6 were screened and compounds 3 and 5 were confirmed as strongly active (). All the MRSA and MRSI strains used in assays have been considered as to be resistant to TTC with MICs ≥ 16 μg/mL (≥36 μM) (EUCAST Citation2000). Compounds 3, 5, and 7 in binary combinations with the standard antibiotics OXA, TTC, and CIP were tested for their antimicrobial activities against several methicillin-resistant strains of S. aureus. The results of the MIC and MBC assays for compounds 3, 5, and 7 are summarized in .
Table 2. MIC values (μg/mL/μM) of 1–6.
Table 3. MIC and MBC values (μg/mL) of compound 3 or 5, ∑FIC and resultant effect of combination with OXA against various strains of MRSA and MRSI.
Table 4. MIC and MBC values (μg/mL) of compound 3 or 5, ∑FIC and resultant effect of combination with TTC against various strains of MRSA and MRSI.
Table 5. MIC and MBC values (μg/mL) of compound 3 or 5, ∑FIC and resultant effect of combination with CIP against various strains of MRSA.
Table 6. MIC and MBC values (μg/mL) of compound 7, ∑FIC and resultant effect of combination with TTC or OXA against various strains of MRSA.
shows the values obtained from the testing of 3 and 5, with the β-lactam antibiotic OXA. The individual values of MIC or MBC for each agent against different strains of staphylococcus are displayed alone with the values of the FICs, the ∑FICs and the effect resulting from the agent or combination of agents. In six cases, our results showed overall synergistic effects (∑FIC 0.266–0.5) for combinations of flavonoids 3 or 5 with OXA. Compound 5 combined with OXA demonstrated synergy: against MRSI 82110 (∑FIC = 0.375, MIC 8-fold reduction), MRSA 1903 (∑FIC = 0.266, MIC 4-fold reduction) and MRSA 3202 (∑FIC = 0.5, MIC 4-fold reduction). A synergistic effect of compound 3 combined with OXA against three strains of MRSA was also observed: MRSA 6975 (∑FIC = 0.313, MIC 16-fold reduction); MRSA 3202 (∑FIC = 0.375, MIC 8-fold reduction); and MRSA 1679 (∑FIC = 0.266, MIC 4-fold reduction). Additive effects were found for combinations of compound 5 and OXA against four strains of MRSA: 6975, 62097, 1679, and 630 (∑FIC 0.531–0.625). The values obtained for the additivity effects observed for compound 3 combined with OXA against MRSI 82110, MRSA 1903, MRSA 67755, and MRSA 630 (∑FIC 0.508–0.625) were barely above the upper limit for synergy. Based on the publication of Eumkeb et al. (Citation2012), we postulated three hypothetical mechanisms for the antibacterial action of flavonoids 3 and 5 and their possible mechanism of synergy: (1) inhibition of the synthesis of the peptidoglycan layer of the bacterial cell wall, (2) alteration of the permeability of the cell wall, and (3) inhibition of β-lactamase. We also suggest that more than one mechanism of synergistic action is possible. The obvious example is baicalein (5,6,7-trihydroxyflavone), which exhibits synergistic effects with tetracycline and β-lactam antibiotics against MRSA (Fujita et al. Citation2005). The mechanism of its action is caused probably by the inhibition of efflux pumps and PBP2a or with the interaction with membrane peptidoglycan (Wagner & Ulrich-Merzenich Citation2009; Chan et al. Citation2011).
Several recent studies have reported synergistic antibacterial effects for combinations of flavonoids with β-lactams or cephalosporins, including effect on MRSA (Eumkeb et al. Citation2010; An et al. Citation2011; Eumkeb et al. Citation2012; Eumkeb & Chukrathok Citation2013). Synergistic and additive effects have also been observed for some prenylated or geranylated flavonoids. In combination with mupirocin, bidwillon B, a prenylated isoflavanone isolated from Erythrina variegata L. Merr. has been tested against 12 strains of MRSA, and in 11 strains, it showed synergy or additivity, acting by inhibiting the incorporation of thymidine, uridine, glucose, and isoleucine, into the cells of MRSA (Sato et al. Citation2004). Binary combinations of sophoraflavanone G, a geranylated flavanone isolated from Sophora spp., with vancomycin, fosfomycin, methicillin, cefzonam, gentamicin, minocycline, or levofloxacin, have inhibited 27 strains of MRSA. An increase in the inhibitory activity of vancomycin during the second stage of the synthesis of the bacterial cell wall has been postulated as a mechanism fostering the synergy that results from combining it with sophoraflavanone G (Sakagami et al. Citation1998).
The effects of combining compound 3 or 5 with TTC were designated as additive (∑FIC 0.563–1) for five strains of staphylococcus (four of them resistant to methicillin) as shown in . Synergistic effects have been observed for combinations of baicalein with TTC or CIP. It has been suggested that some strains of MRSA were inhibited by blocking the Tet(K) efflux system. Previously obtained results showed clearly that not only the inhibition of efflux but also interference with PBP or inhibition of an enzyme such as methyltransferase might contribute to the mechanism of action (Chan et al. Citation2011). Unfortunately, we found no significant synergy for combinations of compound 3 or 5 with CIP as shown in , where MRSA 3202 (∑FIC 0.625) and MRSA 6975 (∑FIC 0.75) were the most sensitive bacterial strains.
Synergy between compound 7 and antibiotics has been shown in many cases, especially for the β-lactams benzylpenicillin, oxacillin, methicillin, ampicillin, and cefalexin (Zhao et al. Citation2001); the tetracyclines (Sudano Roccaro et al. Citation2004); and the macrolids (Kurinčič et al. Citation2012). Mechanisms for the antibacterial action of compound 7 are thought to be similar to those previously described for compounds 3 and 5. In particular, the disruption of the integrity of the cell wall has been clearly established using MHB supplemented with external peptidoglycan and compound 7. When linked to peptidoglycan, EGCG (7) loses its antibacterial activity (Zhao et al. Citation2001). Experiments with strains of MRSA containing efflux pumps have shown compound 7 to be a promising agent for inhibiting Tet(K) and Tet(B) efflux systems (Rodríguez-Rojas et al. Citation2013). As shows, a synergistic effect was found in two cases: compound 7 combined with TTC and used against MRSA 6975 (∑FIC 0.375) and compound 7 combined with OXA and used against MRSA 3202 (∑FIC 0.5). The other results showed additivity (∑FIC 0.531-1) for the strains of MRSA 1903, MRSA 63718, MRSA 62097, MRSA 62059, MRSA 67755, and MRSA 1679.
The present work confirms the potential of geranylated phenolic compounds for the use in controlling the growth of antibiotic-resistant micro-organisms and several Leishmania species. Geranylated flavonoids were effective in lowering the MIC values of the antibiotics OXA and TTC tested on several MRSA strains. The results show the presence of the unmodified geranyl side chain as a structural fragment important for activity. The most active compound 5 possesses the 3-OH hydroxyl group. Compounds 3 and 5 alone, or combined with antibiotics, have demonstrated antibacterial activity in vitro that is potentially useful in combinatory therapy targeted at MRSA infections. According to our best knowledge, this is the first report of the possible synergistic effects of compounds 3 and 5 combined with oxacillin and their additive effects with tetracycline, however, on some of the strains only. The antileishmanial activity of compounds 2 and 4 was comparable with that of miltefosine. The most active compounds tested here were 3′-O-methyl substituted at the flavanone ring B. The activity of 3′-O-methyl substituted dihydroflavonol 3 was diminished probably due to the presence of 3-OH substitution; however, it is difficult to predict a structure–activity relationship for the compounds tested, because further assay showed that a small structural change of geranylated flavonoid at the ring B can affect the mechanism of antileishmanial effect. It is now clear that further studies to evaluate the mechanism of action and efficacy of these compounds both in vitro and in vivo are needed.
Acknowledgement
The authors thank to Frank Thomas Campbell for the language editing of the manuscript.
Declaration of interest
The authors express their thanks to Erasmus scholarship for Ondřej Nešuta. The work was supported by 93/2012/FaF, 63/2014/FaF, and 94/2013/FaF of UVPS Brno. Authors also thank the Project CIETUS 2010 for support.
References
- Abreu AC, McBain AJ, Simoes M. 2012. Plants as sources of new antimicrobials and resistance-modifying agents. Nat Prod Rep. 29:1007–1021.
- Alanis AJ. 2005. Resistance to antibiotics: are we in the post-antibiotic era? Arch Med Res. 36:697–705.
- An J, Zuo GY, Hao XY, Wang GC, Li ZS. 2011. Antibacterial and synergy of a flavanonol rhamnoside with antibiotics against clinical isolates of methicillin-resistant Staphylococcus aureus (MRSA). Phytomedicine 18:990–993.
- Andremont A, Bonten M, Kluytmans J, Carmeli Y, Cars O, Harbarth S. 2011. Fighting bacterial resistance at the root: need for adapted EMEA guidelines. Lancet Infect Dis. 11:6–8.
- Bassetti M, Nicco E, Mikulska M. 2009. Why is community-associated MRSA spreading across the world and how will it change clinical practice? Int J Antimicrob Agents. 34:S15–S19.
- Borges-Argáez R, Balnbury L, Flowers A, Giménez-Turba A, Ruiz G, Waterman PG, Peña-Rodríguez LM. 2007. Cytotoxic and antiprotozoal activity of flavonoids from Lonchocarpus spp. Phytomedicine 14:530–533.
- Bruchhaus I, Roeder T, Rennenberg A, Heussler VT. 2007. Protozoan parasites: programmed cell death as a mechanism of parasitism. Trends Parasitol. 23:376–383.
- Casey AL, Lambert PA, Elliot TS. 2007. Staphylococci. Int J Antimicrob Agents. 29:S23–S32.
- Chan BCL, Ip M, Lau CBS, Lui SL, Jolivalt C, Ganem-Elbaze C, Litaudon M, Reiner NE, Gong HS, See RH, et al. 2011. Synergistic effects of baicalein with ciprofloxacin against NorA over-expressed methicillin-resistant Staphylococcus aureus (MRSA) and inhibition of MRSA pyruvate kinase. J Ethnopharmacol. 137:767–773.
- Clinical and Laboratory Standards Institute (CLSI). 2009. Methods for dilution antimicrobial susceptibility tests for bacteria that grow aerobically: approved standard. 8th ed. Wayne (PA): CLSI. (DocumentM7-A8).
- Cortázar TM, Coombs GH, Walker J. 2007. Leishmania panamensis: comparative inhibition of nuclear DNA topoisomerase II enzymes from promastigotes and human macrophages reveals anti-parasite selectivity of fluoroquinolones, flavonoids and pentamidine. Exp Parasitol. 475–482.
- Eumkeb G, Chukrathok S. 2013. Synergistic activity and mechanism of action of ceftazidime and apigenin combination against ceftazidime-resistant Enterobacter cloacae. Phytomedicine 20:262–269.
- Eumkeb G, Sakdarat S, Siriwong S. 2010. Reversing β-lactam antibiotic resistance of Staphylococcus aureus with galangin from Alpinia officinarum Hance and synergism with ceftazidime. Phytomedicine 18:40–45.
- Eumkeb G, Siriwong S, Thumanu K. 2012. Synergistic activity of luteolin and amoxicillin combination against amoxicillin-resistant Escherichia coli and mode of action. J Photochem Photobiol B Biol. 117:247–253.
- European Committee on Antimicrobial Susceptibility Testing (EUCAST). 2000. Terminology relating to methods for determination of susceptibility of bacteria to antimicrobial agents. Clin Microbiol Infect. 6:503–508.
- French GL. 2010. The continuing crisis in antibiotic resistance. Int J Antimicrob Agents. 36:S3–S7.
- Fujita M, Shiota S, Kuroada T, Hatano T, Yoshida T, Mizushima T, Tsuchiya T. 2005. Remarkable synergies between baicalein and tetracycline, and baicalein and beta-lactams against methicillin-resistant Staphylococcus aureus. Microbiol Immunol. 49:391–396.
- Galzi JL, Hachet-Haas M, Bonnet D, Daubeuf F, Lecat S, Hibert M, Haiech J, Frossard N. 2010. Neutralizing endogenous chemokines with small molecules. Principles and potential therapeutic applications. Pharmacol Therapeut. 126:39–55.
- Hošek J, Závalová V, Šmejkal K, Bartoš M. 2010. Effect of diplacone on LPS-induced inflammatory gene expression in macrophages. Folia Biol. (Praha) 56:124–130.
- Jean-Moreno V, Rojas R, Goyeneche D, Coombs GH, Walker J. 2006. Leishmania donovani: differential activities of classical topoisomerase inhibitors and antileishmanials against parasite and host cells at the level of DNA topoisomerase I and in cytotoxicity assays. Exp Parasitol. 112:21–30.
- Kollár P, Bárta T, Závalová V, Šmejkal K, Hampl A. 2011. Geranylated flavanone tomentodiplacone B inhibits proliferation of human monocytic leukaemia (THP-1) cells. Br J Pharmacol. 162:1534–1541.
- Kurinčič M, Klančnik A, Smole Možina S. 2012. Epigallocatechin gallate as a modulator of Campylobacter resistance to macrolide antibiotics. Int J Antimicrob Agents. 40:467–471.
- Muzitano MF, Tinoco LW, Guette C, Kaiser CR, Rossi-Bergmann B, Costa SS. 2006. The antileishmanial activity assessment of unusual flavonoids from Kalanchoe pinnata. Phytochemistry 67:2071–2077.
- Navrátilová A, Schneiderová K, Veselá D, Hanáková Z, Fontana A, Dall'Acqua S, Cvačka J, Innocenti G, Novotná J, Urbanová M, et al. 2013. Minor C-geranylated flavanones from Paulownia tomentosa fruits with MRSA antibacterial activity. Phytochemistry 89:104–113.
- Nešuta O, Lopez-Aban J, Shariati F, Yepes E, Navrátilová A, Šmejkal K, Žemlička M, Muro A. 2011. Polyphenols as inhibitors of NO production in LPS-stimulated rat macrophages. J Nat Pharm. 2:15–19.
- Nour AM, Khalid SA, Kaiser M, Brun R, Abdalla WE, Schmidt TJ. 2010. The antiprotozoal activity of methylated flavonoids from Ageratum conyzoides L. J Ethnopharmacol. 129:127–130.
- Nový P, Rondevaldová J, Kouřimská L, Kokoška L. 2013. Synergistic interactions of epigallocatechin gallate and oxytetracycline against various drug resistant Staphylococcus aureus strains in vitro. Phytomedicine 20:432–435.
- Radwan MM, El Sohly MA, Slade D, Ahmed SA, Wilson L, El-Alfy AT, Khan IA, Ross SA. 2008a. Non-cannabinoid constituents from a high potency Cannabis sativa variety. Phytochemistry 69:2627–2633.
- Radwan MM, Ross SA, Slade D, Ahmed SA, Zulfiqar F, Elsohly MA. 2008b. Isolation and characterization of new cannabis constituents from a high potency variety. Planta Med. 74:267–272.
- Rocha LG, Almeida JRGS, Macêdo RO, Barbosa-Filho JM. 2005. A review of natural products with antileishmanial activity. Phytomedicine 12:514–535.
- Rodríguez-Rojas A, Rodríguez-Beltrán J, Couce A, Blázquez J. 2013. Antibiotics and antibiotic resistance: a bitter fight against evolution. Int J Med Microbiol. 303: 293–297.
- Sakagami Y, Mimura M, Kajimura K, Yokoyama H, Linuma M, Tanaka T, Ohyama M. 1998. Anti-MRSA activity of sophoraflavanone G and synergism with other antibacterial agents. Lett Appl Microbiol. 27:98–100.
- Salem MM, Capers J, Rito S, Werbovetz KA. 2011. Antiparasitic activity of C-geranyl flavonoids from Mimulus bigelovii. Phytother Res. 25:1246–1249.
- Salem MM, Werbovetz KA. 2006. Natural products from plants as drug candidates and lead compounds against leishmaniasis and trypanosomiasis. Curr Med Chem. 13:2571–2598.
- Sato M, Tanaka H, Yamaguchi R, Kato K, Etoh H. 2004. Synergistic effects of mupirocin and an isoflavanone isolated from Erythrina variegata on growth and recovery of methicillin-resistant Staphylococcus aureus. Int J Antimicrob Agents. 24:241–246.
- Sen N, Das BB, Ganguly A, Banerjee B, Sen T, Majumder HK. 2006. Leishmania donovani: intracellular ATP level regulates apoptosis-like death in luteolin induced dyskinetoplastid cells. Exp Parasitol. 114:204–214.
- Šmejkal K, Chudík S, Klouček P, Marek R, Cvačka J, Urbanová M, Julínek O, Kokoška L, Šlapetová T, Holubová P, et al. 2008. Antibacterial C-geranylflavonoids from Paulownia tomentosa fruits. J Nat Prod. 71:706–709.
- Sudano Roccaro A, Blanco AR, Giuliano F, Rusciano D, Enea V. 2004. Epigallocatechin-gallate enhances the activity of tetracycline in staphylococci by inhibiting its efflux from bacterial cells. Antimicrob Agents Chemother. 48:1968–1973.
- Varela-M RE, Villa-Pulgarin JA, Yepes E, Müller I, Modolell M, Muñoz DL, Robledo SM, Muskus CE, López-Abán J, Muro A, et al. 2012. In vitro and in vivo efficacy of ether lipid edelfosine against Leishmania spp. and SbV-resistant parasites. PLoS Negl Trop Dis. 6:e1612. doi:10.1371/journal.pntd.0001612.
- Wagner H, Ulrich-Merzenich G. 2009. Synergy research: approaching a new generation of phytopharmaceuticals. Phytomedicine 16:97–110.
- Zhao WH, Hu ZQ, Okubo S, Hara Y, Shimamura T. 2001. Mechanism of synergy between epigallocatechin gallate and beta-lactams against methicillin resistant Staphylococcus aureus. Antimicrob Agents Chemother. 45:1737–1742.