abstract
Context 2a,-3a,-24-Trihydroxyurs-12-en-28-oic acid (TEO, a corosolic acid analogue) is a triterpenoid saponin isolated from Actinidia valvata Dunn (Actinidiaceae), a well-known traditional Chinese medicine.
Objective This study investigated the anti-proliferation and inducing apoptosis effects of TEO in three human hepatocellular carcinoma (HCC) cell lines.
Materials and methods Cytotoxic activity of TEO was determined by the MTT assay at various concentrations from 2.5 to 40 μg/mL in BEL-7402, BEL-7404 and SMMC-7721 cell lines. Cell morphology was assessed by acridine orange/ethidium bromide and 4′-6-diamidino-2-phenylindole dihydrochloride staining and fluorescence microscopy. Cell-cycle distribution and DNA damage were determined by flow cytometry and comet assay. Mitochondrial dysfunction was assessed by JC-1 staining and transmission electron microscopy. Apoptosis changes were explored by Western blot, TNF-α and caspase-3, -8, -9 assays.
Results TEO exhibited inhibition effects on BEL-7402, BEL-7404 and SMMC-7721 cells treated for 24 h, the IC50 values were 34.6, 30.8 and 30.5 μg/mL, respectively. TEO (40 μg/mL)-treated three cell lines increased by more than 21% in the G1 phase and presented the morphological change and DNA damage. TEO also declined the mitochondrial membrane potential and altered mitochondrial ultra-structure. Furthermore, caspase-3, caspase-8, caspase-9 and TNF-α were also activated. Mechanism investigation showed that TEO could decrease anti-apoptotic Bcl-2 protein expression, increase proapoptotic Bax and Bid proteins expressions and increase Bax/Bcl-2 ratio.
Conclusion Our results demonstrate for the first time that TEO inhibited growth of HCC cell lines and induced G1 phase arrest. Moreover, proapoptotic effects of TEO were mediated through the activation of TNF-α, caspases and mitochondrial pathway.
Introduction
Hepatocellular carcinoma (HCC) is one of the most prevalent malignancies worldwide. It is currently the second leading cause of cancer-related death, accounting for approximately 800 000 deaths every year (Gomaa & Waked Citation2015). In general, the clinical treatments mainly include surgery, chemotherapy, radiation and liver transplantation. Among them, surgical resection of tumour is still the most effective treatment for HCC. However, owing to poor prognoses and high recurrence rates after resection, a large number of patients present with advanced disease and are not eligible for surgery (Zender et al. Citation2006; Mlynarsky et al. Citation2015). Although chemotherapy is an alternative choice for advanced HCC, the efficacy of chemotherapy is often hampered by a range of adverse side effects and makes patients suffer badly and even to die (Lu et al. Citation2010). Therefore, we need to focus on the development of more effective and alternative therapeutic drugs against this disease.
In recent years, Chinese herbal medicine has been extensively used in various anti-cancer research and a number of them have been identified as owing anti-cancer properties (Zhai et al. Citation2013; Ling et al. Citation2014; Yang et al. Citation2015). Actinidia valvata Dunn (Actinidiaceae) is a shrub mainly growing in eastern China and has a series of applications in traditional Chinese medicine and as a folk herb. The root of this plant, commonly known as Mao renshen in China, exhibited notable anti-inflammatory and anti-tumour activities and has been used for the treatment of hepatoma, lung carcinoma and myeloma for a long time (Li Citation2007; Wang et al. Citation2005). For over a decade, a series of studies were carried out on its pharmacological activities in our laboratory, among the active compounds in Mao renshen. Triterpenoid saponins have been demonstrated as one of the main components responsible for the pharmacological activities including anti-inflammatory, anti-metastasis and anti-tumour effects (Zhang et al. Citation2006; Yi et al. Citation2009; Zheng et al. Citation2012). In order to further study the biological activities of these saponins, a series of compounds have been isolated and identified and some of them showed significant anti-cancer activities (Xin et al. Citation2010; Qu et al. Citation2012). We have demonstrated that corosolic acid had strong potentials in treating human cervix adenocarcinoma and improving cancer chemotherapy (Xu et al. Citation2009). In this study, we focused on a corosolic acid analogue (2α,-3α,-24-trihydroxyurs-12-en-28-oic acid, ), which was isolated from Mao renshen and also has been separated from other medicinal plants, such as Tripterygium wilfordii Hook. f. (Celastraceae) and Actinidia chinensis (Actinidiaceae) (Tian et al. Citation2010; Xu et al. Citation2010). Previous studies have shown that TEO had different degrees of cytotoxicity against six tumour cell lines (Xin et al. Citation2010). In this paper, we investigated the antiproliferation and inducing apoptosis effects of TEO in three HCC cell lines and the possible underlying mechanism.
Materials and methods
Materials
TEO isolated from the root of A. valvata in our laboratory was structurally elucidated by IR, NMR, MS and its purity was determined by conventional methods (HPLC, TLC) was above 97% (Zheng et al. Citation2012).
Tumour necrosis factor radio-immunoassay kit was purchased from Puer Biotechnology Co. Ltd. (Beijing, China). Caspase-3, caspaes-8 and caspase-9 activity assay kit, trizol reagent, cell lysis buffer for Western blot and IP were purchased from Beyotime Institute of Biotechnology (Nantong, China). Antibodies against Bcl-2, Bax, Bid and GAPDH were supplied by Santa Cruz Biotechnology (Santa Cruz, CA). All other chemicals and solvents were from Sigma-Aldrich Corp. (St. Louis, MO).
Cell culture
HCC cell lines BEL-7402, BEL-7404 and SMMC-7721 were obtained from Second Military Medical University and cultured in RPMI-1640 containing 10% (v/v) FCS at 37 °C in an incubator containing 5% CO2. In all experiments, cells were allowed to adhere and grow for 24 h in a culture medium prior to exposure to TEO. To determine the extent of apoptosis in our assays, we collected all fractions, attached and floating cells in culture supernatant.
Cell viability assay
Cell viability was determined by measuring the absorbance of 3-(4,5-dimethylthiazol-2-yl)-2,5-diphenyltetrazolium bromide (MTT) dye staining of living cells. Briefly, cells were incubated in 100 μL of media in 96-well plates at an initial cell density of 1 × 106 cells/mL. After 24 h incubation, different concentrations of TEO were added to cells, and an appropriate volume of drug vehicle was added to untreated cells. After 24 and 48 h of incubation, 10 μL MTT solutions (5 mg/mL in PBS) were added and cells were incubated for a further 4 h. SDS–isobutanol–HCl solutions (100 μL) (10% SDS, 5% isobutanol and 12 μM HCl) were added to each culture and incubated overnight. Relative cell viability was obtained by scanning with a microplate reader (Bio-Rad, San Diego, CA) with a 570 nm filter. Five wells per dose were counted in each experiment.
Cell cycle analysis
Cells were seeded at 1 × 106 cells/mL in a six-well plate, cultured in 10% FCS medium with or without TEO. Cells were then harvested by trypsinisation, washed twice with PBS and centrifuged at 2000g for 5 min. The cells were fixed with 75% ethanol at 4 °C for 24 h. The DNA of the cells was stained with PI staining solution in the dark room for 30 min and analysed using an EPICS XL flow cytometry system with Medical Mcycle software (Beckman Coulter, CA).
Assessment of nuclear morphology
Acridine orange/ethidium bromide (AO/EB) staining was carried out according to the procedure (Zhang et al. Citation2009). The cells were plated at 1 × 106 cells/mL in a six-well plate and incubated for 24 h. Then, the cells were, respectively, treated with TEO (0, 20 and 40 μg/mL) for 24 h. At the end of the treatment, the cells were stained with AO/EB dye mix (100 μg/mL AO and 100 μg/mL EB in PBS) for 5 min followed by fixation with 95% ethanol for 15 min. For 4′-6-diamidino-2-phenylindole dihydrochloride (DAPI) staining, after treatment with TEO, the cells were harvested, washed with PBS and fixed with 10% paraformaldehyde in PBS for 20 min at room temperature. The fixed cells were washed three times with PBS for 5 min each time, and stained with 1.0 μg/mL DAPI solution for 15 min at room temperature. The cells were washed three times with PBS for 5 min each time and analysed via a fluorescence microscope (Olympus, Tokyo, Japan).
Comet assay
HCC cell lines were seeded in a six-well cell culture plate at a density of 1 × 106 cells/mL. Then, cells were exposed to TEO using the same method as described above. The comet assay was performed under alkaline conditions as per the manufacturer’s instructions (Cell Biolabs Inc., San Diego, CA). Images of the cells were obtained using a fluorescence microscope. At least 150 randomly selected cells (50 cells from each of the three replicate slides) were analysed per sample with the Comet assay software project (CASP) 1.2.2 (Biolaunching Technologies Co., Ltd., Beijing, China).
Analysis of changes in mitochondrial membrane potential
Three HCC cell lines were assessed using the 5,5′,6, 6′-tetrachloro-1,1′,3,3′-tetraethylbenzimidazolocarbocyanine iodide (JC-1) kit according to the manufacturer’s instructions (Beyotime Institute of Biotechnology, Nantong, China). This dye accumulates in mitochondria in response to the membrane potential of the organelle. Regions of high membrane potential show up as yellow/orange, whereas areas of lower membrane potential are green. Thus, mitochondrial membrane potential (MMP) was analysed by the fluorescent images obtained using a laser scanning confocal microscope (Leica, TCS SP5, Solms, Germany). Polarised mitochondria are marked by punctate orange-red fluorescent staining.
Transmission electron microscopy
Treated cells were collected by centrifugation and fixed using 2.5% (v/v) glutaraldehyde solution, and washed three times with 0.1 M sodium cacodylate (pH 7.4), and then post-fixed using 1% (v/v) osmium tetraoxide buffered with 0.1 M sodium cacodylate solution for 2 h at 4 °C. The dark brown pellet was embedded in 1% (w/v) agarose and kept overnight at 4 °C and then dehydrated through a series of ethanol/water mixtures for 10 min each (30%, 50% and 70% ethanol) and acetone/water mixtures for 10 min each [80%, 90%, 95% and 100% acetone (v/v)]. After dehydration, the specimens were embedded in low viscosity Spur's epoxy resin and shaken at 30 °C for 4 h. Then, the cells were transferred to silicon moulds and placed in a hot-air oven for 24 h at 35, 45 and 60 °C, respectively. Ultrathin sections (70 nm) were then cut, placed in copper grids, stained with 2% (w/v) uranyl acetate for 20 min and 1% (w/v) lead citrate for 15 min, washed with 1% (w/v) sodium hydroxide and water twice, and then observed with a JEM 2000EX (Jeol, Tokyo, Japan) transmission electron microscope.
Tumour necrosis factor-α assay
The dose of TEO at 5, 10, 20 μg/mL was used to treat BEL-7402, BEL-7404 and SMMC-7721 cell lines at 24 h. The determination of tumour necrosis factor-α (TNF-α) concentration was carried out according to the introduction of the Tumour Necrosis Factor Radio-immunoassay kit. The data were analysed using a ZC-2010 γ-counter (USTC Chuangxin Co., Ltd., Hefei, China).
Caspase-3, caspase-8 and caspase-9 activities assay
Cell supernatants were collected after the treatment of TEO (5, 10, 20 μg/mL) for 24 h. The activities of caspase-3, caspase-8 and caspase-9 were measured strictly according to the instructions of caspase-3, caspase-8 and caspase-9 activity assay kit. Briefly, a mixture of 80 μL detection buffer, 10 μL samples and 10 μL Ac-IETD-pNA was incubated at 37 °C for 60 min, and OD405 was measured. Their activities were calculated based on the standard curve.
Protein extraction and Western blot analysis
Western blot assays were performed as follows: protein (5 mg/mL) was denatured by mixing with an equal volume of two times sample loading buffer and then boiling at 100 °C for 5 min. An aliquot (20 μL, containing 50 μg protein) of the supernatant was loaded onto a SDS gel, separated electrophoresis and transferred to a PVDF membrane. Twelve percent polyacrylamide gel was used for all the electrophoresis. The transmembrane time for Bcl-2, Bax, Bid and GAPDH was 30, 18, 22 and 30 min, respectively. After the PVDF membrane was incubated with 10 mM TBS with 1.0% Tween 20 and 5% dehydrated skim milk to block non-specific protein binding, the membrane was incubated with primary antibodies overnight at 4 °C with primary antibodies including Bcl-2 (1:500), Bax (1:500), Bid (1:500) and GAPDH (1:1000). Blots were then incubated with horseradish peroxidase-conjugated goat anti-rabbit IgG (Santa) or horseradish peroxidase-conjugated goat anti-mouse IgG (Santa) for 2 h at room temperature at a 1:2000 dilution. Detection was performed by an enhanced chemiluminescence method and photographed by Bio Spectrum Gel Imaging System (UVP, Upland, CA). To exclude variations due to protein quantity and quality, the data were adjusted to GAPDH expression (IOD of objective protein versus IOD of GAPDH protein).
Statistical analysis
All data were analysed by statistical software SPSS 11.5 (SPSS Inc., Chicago, IL) and were expressed as mean ± SD. The significance of differences between the means of the treated and untreated groups has been compared by one-way analysis of variance, followed by unpaired Student’s t-test and p-values less than 0.05 were considered significant.
Results
TEO inhibits the viability of HCC cell lines
To investigate inhibitory effect of TEO on cell proliferation, BEL-7402, BEL-7404 and SMMC-7721 cells were treated with TEO (0, 2.5, 5, 10, 20, and 40 μg/mL) and then assessed by MTT assay. As shown in , TEO could significantly inhibit the viability of the HCC cell lines in a dose-dependent manner. When BEL-7402, BEL-7404 and SMMC-7721 cells were treated for 24 h, the IC50 values of TEO were 34.6, 30.8, and 30.5 μg/mL, respectively.
Figure 2. Effect of TEO on proliferation of BEL-7402, BEL-7404 and SMMC-7721 cell lines. The cells were cultured in 10% FCS medium and treated with TEO (1.25, 2.5, 5, 10, 20, 40 μg/mL) for 24 and 48 h. The experiments were conducted in triplicate and each value indicates the mean ± SD of five independent experiments. *p < 0.05 and **p < 0.01 compared with the control group.
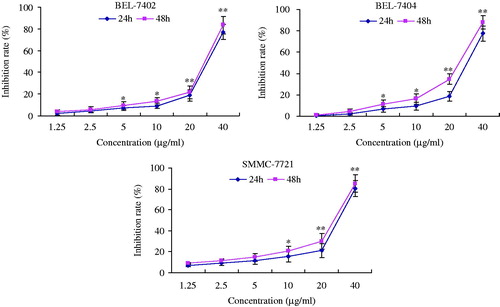
TEO inhibits the proliferation of HCC cell lines
To clarify the inhibition effects of TEO on cells proliferation, we examined the influence of TEO on three HCC cell lines cycle distribution through flow cytometry. Treatment with TEO (40 μg/mL) for 24 h caused an increase in the population of cells in the G1 phase and a decrease in the S phase (). The percentage of BEL-7402, BEL-7404 and SMMC-7721 cells in the G1 phase increased from 60.2% to 73.0%, from 50.3% to 63.3% and from 51.1% to 68.8% after 24 h of incubation with TEO (40 μg/mL), respectively. A significant decrease of BEL-7402, BEL-7404 and SMMC-7721 cells in S phase, from 25.6% to 16.3%, from 32.1% to 23.3% and from 34.2% to 19.0%, respectively, was also observed. These results indicated that TEO inhibited proliferation of three HCC cell lines through G1 phase arrest.
TEO induces the apoptosis of HCC cell lines
Apoptotic morphological changes were assessed by AO/EB and DAPI staining and fluorescence microscopy. As shown in , the cells in untreated groups emitted green fluorescence and nearly no death or apoptosis was observed. In TEO-treated groups, cells emitted yellow or orange fluorescence with different degrees, chromatin condensation and nucleus fragmentation, and apoptotic degrees of three HCC cell lines increased. For DAPI staining (), untreated cells emitted blue fluorescence with consistent nucleus intensity, presenting typical homogeneous distribution of chromatin in nucleus. By contrast, three HCC cell lines treated with 40 μg/mL of TEO for 24 h presented the morphological features of chromatin condensation or fragmentation.
Figure 4. (a) Representative images of AO/EB stained BEL-7402 cells (200×, final magnification), BEL-7404 cells and SMMC-7721 cells (100×, final magnification). After TEO treatment, apoptotic cells (orange fluorescence) exhibited chromatin condensation and apoptotic bodies (arrows). (b) Representative images of DAPI stained three HCC cell lines (200×, final magnification). Arrows indicate nuclear condensation or fragmentation.
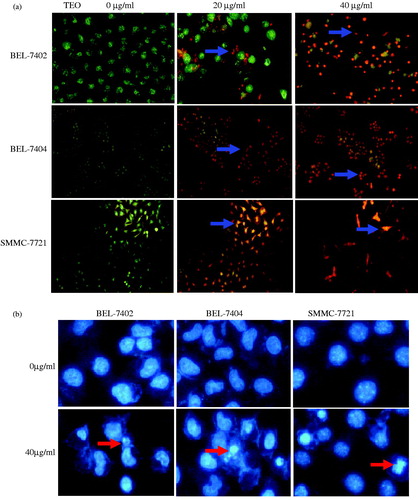
TEO induces the DNA damage of HCC cell lines
Comet assay was performed to determine DNA damage of TEO to three HCC cell lines. As shown in , normal cells showed comets with bright heads and small tails, while TEO-treated (40 μg/mL) cells for 24 h exhibited comets with small heads and long diffuse tails. Head DNA (%) significantly decreased, and the comet length (μm), tail length (μm) and tail DNA (%) of three HCC cell lines significantly increased. The results are listed in .
Figure 5. DNA damage effects of TEO (40 μg/mL) after 24 h treatment on three HCC cell lines were investigated by comet assay (a). These effects were represented as comet tail length (μm), comet length (μm), head DNA (%) and tail DNA (%) of BEL-7402 (b), BEL-7404 (c) and SMMC-7721 (d) cells. Data are expressed as mean ± SD. n=100. **p < 0.01 represents significant difference when compared with control group.
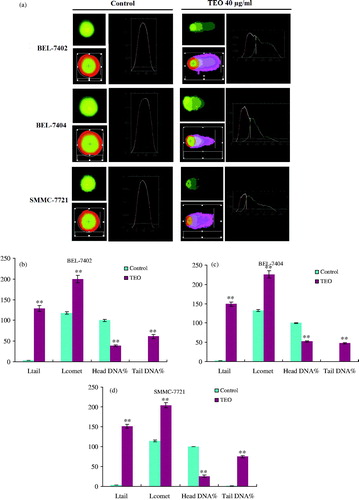
TEO regulates the TNF-α level of HCC cell lines
The TNF-α level in the supernatant of three HCC cell lines was determined, and the content of TNF-α significantly increased, which is shown in . When treated with TEO (20 μg/mL) for 24 h, the content of TNF-α in the supernatant of BEL-7402, BEL-7404 and SMMC-7721 cell lines increased from 1.7 to 7.6 ng/L, from 1.6 to 7.1 ng/mL and from 1.8 to 6.4 ng/mL, respectively.
TEO reduces the MMP of HCC cell lines
Mitochondrial damage, which is readily measured using JC-1 staining, is often associated with MMP (Δψm) loss. Intact mitochondrial membrane allows accumulation of JC-1 in the mitochondria, where it will generate red fluorescent light when a critical concentration is reached. As loss of Δψm occurs, JC-1 cannot accumulate in the mitochondria and will remain as a monomer in the cytosol and generate green fluorescence (Derosier et al. Citation2007). In this study, representative fluorescent images of MMP detected by JC-1 are presented in . The cells in untreated group showed red fluorescence, while TEO (40 μg/mL)-treated cells showed bright green fluorescence, which indicated that TEO obviously decreased MMP.
TEO induces mitochondrial ultra-structural alterations of HCC cell lines
Mitochondrial dysfunction was associated with structural abnormalities. The transmission electron microscopy (TEM) of untreated HCC cells showed that intact mitochondria were clearly visible at the double membrane, and intact cristae structure and a mitochondrial matrix consisting of electron-dense granules were found (). While mitochondrial double membrane was absent and leaky, distorted cristae were depleted in number and the matrix had a reduced number of electron-dense granules in the TEO-treated (40 μg/mL) group. These results demonstrated that TEO induced the mitochondrial injury of three HCC cell lines.
TEO adjusts the apoptosis-related protein expression in HCC cell lines
The involvement of the mitochondrial proteins Bcl-2, Bax and Bid in three HCC cell lines was investigated by Western blot. The levels of them were quantitated in the untreated and treated cells using GAPDH for normalisation (), TEO treatment (20 and 40 μg/mL) of three HCC cell lines for 24 h resulted in significantly increased levels of Bax and Bid proteins and decreased levels of Bcl-2 protein. Increased Bax/Bcl-2 ratios in treated cells suggested a proapoptotic environment within the cells. For 10, 20 and 40 μg/mL TEO treatment, the ratios were 1.1, 4.8 and 8.2 to BEL-7402 cells, were 3.8, 12.8 and 17.1 to BEL-7404 cells, and were 1.6, 2.2 and 6.9 to SMMC-7721 cells, respectively.
Figure 8. Expression levels of Bcl-2, Bax and Bid proteins in TEO-treated BEL-7402 (a), BEL-7404 (b) and SMMC-7721 (c) cell lines. (d–f) Bax/Bcl-2 ratios of three HCC cell lines. Data are representative of means ± SD from three independent experiments. *p < 0.05 and **p < 0.01 compared with control group.
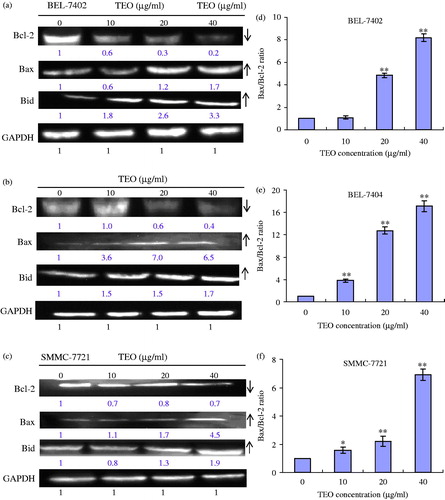
TEO increases the caspases activities of HCC cell lines
Caspases are also known to act as important mediators of apoptosis and to contribute to the overall apoptotic morphology through the cleavage of various cellular substrates. In this study, caspase-3, caspase-8 and caspase-9 activities assay showed a significant increase in lysates of TEO-treated cells. After BEL-7402 cells were treated with TEO (20 μg/mL) for 24 h, the activities of caspase-3, caspase-8 and caspase-9 were about 1.9, 2.2 and 2.2 times, respectively, more than those of the untreated cells. Corresponding values for BEL-7404 cells were about 2.3-, 2.1- and 2.3-fold higher than those untreated cells. In the case of SMMC-7721 cells, the values for activities of caspase-3, caspase-8, and caspase-9 were about 2.5, 2.1 and 2.3 times, respectively, compared with untreated cells (shown in ). These results indicated that TEO induced apoptosis in three HCC cell lines, at least in part through a caspase-dependent pathway.
Discussion
Nowadays, much effort has been focused on researching natural compounds from plants for cancer treatment. As a class of natural products, triterpenoid saponins existed widely in plants and were reported to have a variety of biological activities, including anti-cancer effects (Xavier et al. Citation2013; Lee et al. Citation2015; Olivier et al. Citation2015). In the present study, we demonstrated that a triterpenoid saponin isolated from A. valvata, TEO, showed significant anti-proliferating activities and inducing apoptotic effects on three HCC cell lines.
By MTT assay, we demonstrated that TEO could significantly suppress three HCC cell lines viability in a dose-dependent manner. Inducing cell cycle arrest and apoptosis was considered to be an attractive approach for the treatment of cancer. To determine if the proliferation inhibition of TEO in three HCC cell lines involved cell cycle arrest, we investigated cell cycle phase distribution of the treated cells by flow cytometry. G1 phase arrests were obvious when HCC cell lines were treated with 40 μg/mL of TEO for 24 h. The results suggested that TEO induced G1 phase arrest and thus slowed down cell cycle progression and inhibited cell growth.
Apoptotic morphological changes induced by TEO were assessed by AO/EB and DAPI staining. With AO/EB staining, the apoptotic cells appeared orange and nucleus crimpled. Normal nucleus had an oval shape and emitted green fluorescence, whereas apoptotic nuclei, characterised by orange fluorescence, condensed nuclei and apoptotic bodies, obviously appeared after exposure to TEO for 24 h. Similar effects were also observed by DAPI staining that TEO (40 μg/mL) treatment markedly induced chromatin condensation or fragmentation of the cells. These results suggested that TEO induced apoptosis of three HCC cell lines.
DNA damage is one of the molecular events associated with cell cycle arrest and apoptosis, and many anti-cancer reagents could induce DNA damage (Ding et al. Citation2009; Wu et al. Citation2012; Singh et al. Citation2014). Comet assay is a very simple, highly sensitive, reliable and fairly inexpensive technique and therefore used widely for the evaluation of DNA damage and repair studies (Olive Citation2009; Rao et al. Citation2011). The size and shape of the comet and the distribution of DNA within the comet were correlated with the extent of DNA damage (Fairbairn et al. Citation1995). The comet assay used in this study was conducted to measure DNA damage in three HCC cell lines following TEO treatment. When treated with 40 μg/mL TEO, the DNA damage appeared and comet parameters like comet tail length and tail DNA markedly increased compared with those of untreated cells, among of them, DNA damage in SMMC-7721 cells appeared to be the worst since the comet tail length (μm) and tail DNA (%) were greatest, which were 151.8 ± 4.7 μm and 74.5 ± 5.4%, respectively. The results indicated that cell cycle arrest and apoptosis might be attributed to TEO-induced DNA damage.
Mitochondrion is an important organelle, which regulates the production of cellular energy through metabolism and involves in programmed cell death (Ishikawa et al. Citation2008). The decline in MMP is a marker for early apoptosis. In this study, TEO-treated cells showed green fluorescence, which demonstrated that TEO could obviously induce the collapse of MMP in HCC cell lines. TEM can observe the microanatomy of inner ultra-structure and thus has been widely used to study mitochondria (Modla et al. Citation2010). The TEM images suggested that TEO could directly affect mitochondrial post-protoconal valley membrane leading to fusion and rupture, which indicated that apoptosis of three HCC cell lines induced by TEO was probably through regulating mitochondrial dysfunction pathway in our study.
The mitochondrial function is regulated through Bcl-2 family proteins comprising both anti-apoptotic and proapoptotic proteins (Adams & Cory Citation2007). Bax and Bcl-2 have been identified as major regulators and the Bax/Bcl-2 ratio plays an important role in determining whether cells will undergo apoptosis (Luo et al. Citation1998). A significant increase in Bax protein expression and a decrease in Bcl-2 protein expression were observed in three HCC cell lines after 24 h of TEO treatment. The results of this study presented an increase in Bax/Bcl-2 ratio, which further indicated that TEO activated the mitochondria pathway and then induced the apoptosis of three HCC cell lines.
The consecutive activation of caspase family members is considered to be a pre-requisite of execution of apoptosis (Sinha et al. Citation2006). In particular, activation of caspase-3 plays a central role in the initiation of apoptosis (Nicholson et al. Citation1995; Chodurek et al. Citation2014), which needs the activation of initiator caspases, such as caspase-8 or caspase-9, in response to pro-apoptotic signals (Zhao et al. Citation2011). Caspase-8 is believed to be activated by engaging certain members of the tumour necrosis factor family and trigger the death receptors apoptotic pathway at cell surface; whereas caspase-9 activation involves the mitochondrion-dependent apoptotic pathway (Zhao et al. Citation2011). Our results showed that after 24 h of TEO treatment, TNF-α, caspase-3, caspase-8 and caspase-9 activities considerably increased, which inferred that caspases were activated in the cells and the mitochondrial pathway was involved in TEO-induced apoptosis.
In conclusion, our study demonstrated that TEO from A. valvata had a significant cytotoxic effect and could induce proliferation inhibition, DNA damage and apoptosis in three HCC cell lines. Furthermore, our results showed that HCC cells treated with TEO, Bax/Bcl-2 ratios increased, characteristic morphological changes, with mitochondrial transmembrane potential collapse and the subsequent activation of caspase cascade. Moreover, we provided evidence that apoptosis induced by TEO was related with mitochondrial pathway activation. These findings suggest that TEO is an effective product for inducing apoptosis of three HCC cell lines, which should be developed into a new natural medicine for the treatment of hepatocarcinoma. However, pharmacological and clinical researches require further investigations.
Declaration of interest
The authors declared that there are no conflicts of interest.
This study was financially supported by National Natural Science Foundation of China (No. 81102849), China Post-Doctoral Foundation (No. 20110491850), Special Project of Biological Medicine of Science and Technology Commission of Shanghai Municipality (No. 10431900500) and supported in part by E-institutes of Shanghai Municipal Education Commission (No. E03008).
References
- Adams JM, Cory S. 2007. The Bcl-2 apoptotic switch in cancer development and therapy. Oncogene. 26:1324–1337.
- Chodurek E, Kulczycka A, Orchel A, Aleksander-Konert E, Dzierzewicz Z. 2014. Effect of valproic acid on the proliferation and apoptosis of the human melanoma G-361 cell line. Acta Pol Pharm. 71:917–921.
- DeRosier LC, Buchsbaum DJ, Oliver PG, Huang ZQ, Sellers JC, Grizzle WE, Wang W, Zhou T, Zinn KR, Long JW, et al. 2007. Combination treatment with TRA-8 anti-death receptor 5 antibody and CPT-11 induces tumor regression in an orthotopic model of pancreatic cancer. Clin Cancer Res. 13:5535–5543.
- Ding L, Liu B, Qi LL, Zhou QY, Hou Q, Li J, Zhang Q. 2009. Anti-proliferation, cell cycle arrest and apoptosis induced by a natural xanthone from Gentianopsis paludosa Ma, in human promyelocytic leukemia cell line HL-60 cells. Toxicol In Vitro. 23:408–417.
- Fairbairn DW, Olive PL, O'Neill KL. 1995. The comet assay: a comprehensive review. Mutat Res. 339:37–59.
- Gomaa AI, Waked I. 2015. Recent advances in multidisciplinary management of hepatocellular carcinoma. World J Hepatol. 7:673–687.
- Ishikawa K, Takenaga K, Akimoto M, Koshikawa N, Yamaguchi A, Imanishi H, Nakada K, Honma Y, Hayashi J. 2008. ROS-generating mitochondrial DNA mutations can regulate tumor cell metastasis. Science. 320:661–664.
- Lee KM, Yun JH, Lee DH, Park YG, Son KH, Nho CW, Kim YS. 2015. Chikusetsusaponin IVa methyl ester induces cell cycle arrest by the inhibition of nuclear translocation of β-catenin in HCT116 cells. Biochem Biophys Res Commun. 459:591–596.
- Li Y. 2007. Professor Liu Jiaxiang’s experience of herbal administration treating cancer. Shanghai J Trad Chin Med. 41:9–10.
- Ling CQ, Yue XQ, Ling C. 2014. Three advantages of using traditional Chinese medicine to prevent and treat tumor. J Integr Med. 12:331–335.
- Lu BN, Hu MM, Liu KX, Peng JY. 2010. Cytotoxicity of berberine on human cervical carcinoma Hela cells through mitochondria, death receptor and MAPK pathways, and in-silico drug-target prediction. Toxicol In Vitro. 24:1482–1490.
- Luo X, Budihardjo I, Zou H, Slaughter C, Wang X. 1998. Bid, a Bcl-2 interacting protein, mediates cytochrome c release from mitochondria in response to activation of cell surface death receptors. Cell. 94:481–490.
- Mlynarsky L, Menachem Y, Shibolet O. 2015. Treatment of hepatocellular carcinoma: steps forward but still a long way to go. World J Hepatol. 7:566–574.
- Modla S, Mendonca J, Czymmek KJ, Akins RE. 2010. Identification of neuromuscular junctions by correlative confocal and transmission electron microscopy. J Neurosci Methods. 191:158–165.
- Nicholson DW, Ali A, Thornberry NA, Vaillancourt JP, Ding CK, Gallant M, Gareau Y, Griffin PR, Labelle M, Lazebnik YA. 1995. Identification and inhibition of the ICE/CED-3 protease necessary for mammalian apoptosis. Nature. 376:37–43.
- Olive PL. 2009. Impact of the comet assay in radiobiology. Mutat Res. 681:13–23.
- Noté OP, Jihu D, Antheaume C, Zeniou M, Pegnyemb DE, Guillaume D, Chneiwess H, Kilhoffer MC, Lobstein A. 2015. Triterpenoid saponins from Albizia lebbeck (L.) Benth and their inhibitory effect on the survival of high grade human brain tumor cells. Carbohyd Res. 404:26–33.
- Qu LP, Zheng GY, Su YH, Zhang HQ, Yang YL, Xin HL, Ling CQ. 2012. New triterpenoids with cytotoxic activity from Actinidia valvata. Int J Mol Sci. 13:14865–14870.
- Rao BN, Archana PR, Aithal BK, Rao BS. 2011. Protective effect of zingerone, a dietary compound against radiation induced genetic damage and apoptosis in human lymphocytes. Eur J Pharmacol. 657:59–66.
- Singh V, Azad GK, Mandal P, Reddy MA, Tomar RS. 2014. Anti-cancer drug KP1019 modulates epigenetics and induces DNA damage response in Saccharomyces cerevisiae. FEBS Lett. 588:1044–1052.
- Sinha S, Pal BC, Jagadeesh S, Banerjee PP, Bandyopadhaya A, Bhattacharya S. 2006. Mahanine inhibits growth and induces apoptosis in prostate cancer cells through the deactivation of Akt and activation of caspases. Prostate. 66:1257–1265.
- Tian Y, Hao SJ, Ma YP, Zhang ZX, Wang JH. 2010. Isolation and identification of chemical constituents of roots of Tripterygium wilfordii Hook.f. J Shenyang Pharm Univ. 27:715–718.
- Wang ZZ, Song Y, Hu JH, You BB. 2005. Morphological identification and the clinical application of the roots of Actinidia chinensis and Actinidia valvata. Pharm Care Res. 5:134–137.
- Wu GS, Lu JJ, Guo JJ, Li YB, Tan W, Dang YY, Zhong ZF, Xu ZT, Chen XP, Wang YT. 2012. Ganoderic acid DM, a natural triterpenoid, induces DNA damage, G1 cell cycle arrest and apoptosis in human breast cancer cells. Fitoterapia. 83:408–414.
- Xavier CPR, Lima CF, Pedro DFN, Wilson JM, Kristiansen K, Pereira-Wilson C. 2013. Ursolic acid induces cell death and modulates autophagy through JNK pathway in apoptosis-resistant colorectal cancer cells. J Nutr Biochem. 24:706–712.
- Xin HL, Wu YC, Xu YF, Su YH, Zhang YN, Yuan H. 2010. Four triterpenoids with cytotoxic activity from the leaves of Actinidia valvata. Chin J Nat Med. 8:260–263.
- Xu YF, Ge RL, Du J, Xin H, Yi T, Sheng J, Wang Y, Ling C. 2009. Corosolic acid induces apoptosis through mitochondrial pathway and caspase activation in human cervix adenocarcinoma HeLa cells. Cancer Lett. 284:229–237.
- Xu YX, Xiang ZB, Jin YS, Shen Y, Chen HS. 2010. Two new triterpenoids from the roots of Actinidia chinensis. Fitoterapia. 81:920–924.
- Yang NM, Han F, Cui H, Huang J, Wang T, Zhou Y, Zhou J. 2015. Matrine suppresses proliferation and induces apoptosis in human cholangiocarcinoma cells through suppression of JAK2/STAT3 signaling. Pharmacol Rep. 67:388–393.
- Yi TJ, Xu YF, Zheng GY, Xin HL, Ling CQ. 2009. Inhibitory effects of total saponin from Actinidia valvata on murine transplanted tumor. Chin Arch Trad Chin Med. 27:1642–1643.
- Zender L, Spector MS, Xue W, Flemming P, Cordon-Cardo C, Silke J, Fan ST, Luk JM, Wigler M, Hannon GJ. 2006. Identification and validation of oncogenes in liver cancer using an integrative oncogenomic approach. Cell. 125:1253–1267.
- Zhai XF, Chen Z, Li B, Shen F, Fan J, Zhou WP, Yang YK, Xu J, Qin X, Li LQ. 2013. Traditional herbal medicine in preventing recurrence after resection of small hepatocellular carcinoma: a multicenter randomized controlled trial. J Chin Integr Med. 11:90–100.
- Zhang YN, Liu L, Ling CQ. 2006. Inhibition effect of active fraction from Actinidia valvata on growth of transplanted mouse tumor cells and preliminary study of its mechanism. Chin J Chin Mater Med. 31: 918–920.
- Zhang Z, Wang S, Qiu H, Duan C, Ding K, Wang Z. 2009. Waltonitone induces human hepatocellular carcinoma cells apoptosis in vitro and in vivo. Cancer Lett. 286:223–231.
- Zhao YY, Shen XF, Chao X, Ho CC, Cheng XL, Zhang Y, Lin RC, Du KJ, Luo WJ, Chen JY, et al. 2011. Ergosta-4,6,8(14),22-tetraen-3-one induces G2/M cell cycle arrest and apoptosis in human hepatocellular carcinoma HepG2 cells. Biochim Biophy Acta. 1810:384–390.
- Zheng GY, Xin HL, Xu XF, Li B, Zhai XF, Zhang YH, Ling CQ. (2012). Total saponin from root of Actinidia valvata dunn inhibits hepatoma 22 growth and metastasis in vivo by suppression angiogenesis. Evid-Based Complement Alternat Med. 2012:432814.