abstract
Context Oxidative stress is a common mechanism of liver injury. Carnosine is a dipeptide having strong antioxidant effects.
Objectives We investigated the effects of carnosine on lead-induced hepatotoxicity and oxidative stress in rats.
Materials and methods Animals received an aqueous solution of lead acetate (500 mg Pb/L in the drinking water) and/or daily oral gavage of carnosine (10 mg/kg) for 8 weeks. Rats were then weighed and used for the biochemical (commercial kits), molecular (standard chemical methods) and histological (microscopic) evaluations.
Results Lead-induced oxidative stress in liver tissue was indicated by a significant increase in the level of malondialdehyde (MDA) (8.25 ± 0.15 nmol/mg) as well as decrease in the level of total antioxidant capacity (TAC) (1.72 ± 0.25 μmol/g) and total thiol (SH) groups) 1.9 ± 0.22 μmol/g). Carnosine treatment decreased MDA (4 ± 0.08 nmol/mg), whereas it increased the contents of total thiol (3.25 ± 0.04 μmol/g) and TAC (3.44 ± 0.32 μmol/g) in the lead group. Carnosine also prevented the decreased body weight (p < 0.001), albumin (p < 0.05) and total protein levels (p < 0.001) and increased liver weight (p < 0.05) and activates of hepatic enzymes (p’s < 0.001) (alanine aminotransferase, aspartate aminotransferase, alkaline phosphatase and lactate dehydrogenase) in the lead group. Furthermore, histopathological study showed that carnosine attenuates liver damage by decreasing necrosis and infiltration of inflammatory cells.
Conclusion Carnosine prevented lead-induced hepatotoxicity, indicated by molecular, biochemical and histopathological analyses through inhibiting lipid peroxidation and enhancing antioxidant defence systems. Therefore, carnosine makes a good candidate to protect against the deleterious effect of chronic lead intoxication.
Introduction
Lead is recognised as one of the most toxic metals and its exposure continues to be a widespread biological problem in many countries (Flora et al. Citation2012; El-Mehi & Amin Citation2012). Sources of human exposure include food, drinking water and dust (Barbosa et al. Citation2009). Lead causes a large spectrum of physiological, biochemical and behavioural disorders in the animals, which are investigated in experiments and laboratories and in humans (Barbosa et al. Citation2009; Mansouri & Cauli Citation2009; Eshginia & Marjani Citation2013). Liver is considered to be the target organ in relation to the toxic effect of lead. Several mechanisms have been proposed to explain lead-induced toxicity (Patra et al. Citation2001; Wang et al. Citation2012). Although the precise mechanism of liver toxicity caused by lead is not clear, there is evidence that lead can cause generation of reactive oxygen metabolites and inhibits the activity of antioxidant enzymes in liver tissue (Sivaprasad et al. Citation2004; Liu et al. Citation2012). Efforts have been made to minimise the severity of lead toxicity via enhanced sequestration and elimination using various agents. In this context, antioxidant therapies have been shown to exert protective effects in lead-induced toxicity (Xia et al. Citation2010; Wang et al. Citation2012).
Carnosine (β-alanyl-L-histidine) is a dipeptide that occurs naturally in some species of vertebrates, including humans. According to previous studies, this small molecule has various biological functions including membrane-stabilising, pH-buffering, metal ion-chelating and macrophage regulating activities (Guiotto et al. Citation2005; Fouad et al. Citation2009; Budzeń & Rymaszewska Citation2013). Carnosine has been shown to be an effective agent to decrease prooxidant status in oxidative stress-induced pathologies such as ischemia–reperfusion (Dobrota et al. Citation2005; Fouad et al. Citation2007) and liver damage (Mehmetçik et al. Citation2008; Artun et al. Citation2010). In this context, previous studies report hepatoprotective effects of carnosine against liver injury induced by thioacetamide (Mehmetçik et al. Citation2008), ethanol (Liu et al. Citation2008) and cadmium (Fouad et al. Citation2009) in experimental models.
To our knowledge, no study has been carried out concerning the protective effects of carnosine on lead-induced hepatotoxicity. Taking the above-mentioned facts into account, the present study was carried out to assess the protective effect of carnosine in lead-induced liver toxicity in rats.
Material and methods
Drugs
Carnosine (β-alanyl-L-histidine) and lead acetate (Pb(CH3COO)2) were obtained from Sigma (St. Louis, MO). 2,2′-Dinitro-5,5′-dithiodibenzoic acid (DTNB), 2,4,6-tri (2-pyridyl)-1,3,5-triazine (TPTZ), 2-thiobarbituric acid (TBA), trichloroacetic acid (TCA), n-butanol, tris-hidrochloride acid, ethylenediamine tetraacetic acid (EDTA), sodium acetate, glacial acetic acid, phosphoric acid, potassium chloride, tetramethoxypropane (TMP), ferric chloride (FeCl3ċ6H2O), ferrous sulphate and hydrochloric acid were obtained from Merck (Dramstadt, Germany). All other chemicals were of analytical grade.
Animals
Male adult Wistar rats (8 weeks old, weighing 220–250 g) were used in the present experiments. All animals were maintained at a constant temperature (22 ± 0.5 °C) with a 12 h light/dark cycle. Food and water were available ad libitum in the home cages. Rats were divided randomly to four experimental groups (n = 7). The rats were habituated to the environment for at least 1 week before the start of the experiment. The study protocol was approved by the Institutional Animal Ethical Committee (No. 71B-2081) and all procedures were performed in accordance with the recommendations for the proper care and use of laboratory animals.
Experimental design
A total number of 27 rats were randomly divided into four equal groups: control, lead-treated group, carnosine + lead-treated group and carnosine group. In the control group, the rats received lead-free redistilled water and daily oral gavage administration of physiological saline (0.9% NaCl) during the whole course of the experiment; in the lead-treated group, the rats received an aqueous solution of lead acetate (500 mg Pb/L as the drinking fluid) and physiological saline by oral gavage daily during the course of the experiment; in the carnosine + lead-treated group, animals received an aqueous solution of lead acetate (500 mg Pb/L in drinking water) and received carnosine (10 mg/kg/d) by oral gavage and in the carnosine group, the animals received carnosine (10 mg/kg/d) dissolved in physiological saline (0.9% NaCl) daily by oral gavage. The experiments lasted for 8 weeks. The treatment protocols and the doses used were based on previously published studies (Soliman et al. Citation2007; Fouad et al. Citation2009; Liu et al. Citation2012; Wang et al. Citation2012). For example, Liu et al. (Citation2012) and Wang et al. (Citation2012) reported that administration of an aqueous solution of lead acetate at a concentration of 500 mg Pb/L in drinking water for 8 weeks induces hepatotoxicity in rats. The choice of carnosine dose is also based on the previous findings that showed protective role of carnosine (10 mg/kg/day) in the experimental models of cadmium-induced hepatotxicity (Fouad et al. Citation2009) and gentamicin-induced nephrotoxicity (Soliman et al. Citation2007). At the end of treatments, rats in each group were weighed and then used for the biochemical, histological and oxidant/antioxidant evaluations.
Biochemical and histopathological analyses
Rats were anesthetised with ketamine/xylazine mixture (ketamine 67 mg/kg, xylazine 6 mg/kg, i.p.) and about 5 mL of blood samples were drawn by cardiac puncture with heparin tubes. The plasma was collected after centrifugation at 4000 rpm for 10 min and stored at 70 °C freezer for biochemical analyses. The concentrations of albumin (g/dL), total protein (g/dL) as well as the activities of alanine aminotransferase (ALT), aspartate aminotransferase (AST), lactate dehydrogenase (LDH) and alkaline phosphatase (ALP) were measured using standard techniques and commercial kits (Pars Azmoon Co., Tehran, Iran) with an auto analyzer (Gcsan Chem 2000, Barcelona, Spain).
Immediately after blood collection, the animals were sacrificed by an overdose of sodium pentobarbital (200 mg/kg, i.p.). The liver of each rat was removed, weighed and washed with ice-cold saline at −80 °C, and subsequently homogenised in cold potassium phosphate buffer (0.05 M, pH 7.4). The liver homogenates were centrifuged at 5000 rpm for 10 min at 4 °C. The resulting supernatant was used for the determination of oxidant/antioxidant markers.
Parts of liver tissue obtained from each rat were fixed in 10% formalin solution for 24 h, dehydrated in ascending grades of mixtures of ethyl alcohol–water, cleaned in xylene and embedded in paraffin. Sections of the tissue were prepared using a rotary microtome and stained with haematoxylin and eosin (H&E) dye, which was mounted in a neutral deparaffinated xylene medium for microscopic observations. Five-micron sections were examined under light microscope by a pathologist unaware of the treatment protocol to detect liver lesions including hepatocyte necrosis, inflammatory cells infiltration, disarrangement of hepatic lobules structures, central vein dilation and degenerated hepatocytes with pyknotic nuclei.
Measurement of lipid peroxidation
Hepatic lipid peroxide was estimated by measuring the formed malondialdehyde (MDA). Briefly, the samples were diluted by 1.5 mL TCA (20% w/v) and centrifuged in 3000 g for 10 min. Then, the precipitation was dissolved in sulphuric acid and 1.5 mL of the mixture was added to 1.5 mL of TBA (0.2% w/v). The mixture was then incubated for 1 h in a boiling water bath. Following incubation, 2 mL of n-butanol was added, the was solution centrifuged, cooled and the absorption of the supernatant was recorded in 532 nm. The calibration curve of tetraethoxypropane standard solutions was used to determine the concentrations of TBA + MDA adducts in samples (Moore & Roberts Citation1998).
Measurement of protein thiol (SH) groups
The total thiol content was estimated based on the Ellman method (Ellman, Citation1959). In this method, total SH groups were measured using DTNB as the reagent. This reagent reacts with the SH groups to produce a yellow complex dianion (5-thio-2-nitrobenzoic acid, TNB) which has a peak absorbance at 412 nm.
Ferric reducing/antioxidant power (FRAP) assay
The basis of FRAP assay is reducing the colourless ferric tripyridyltriazine (TPTZ) complex to blue-coloured ferrous tripyridyltriazine by action of electron-donating antioxidants in biological samples (Benzie & Strain Citation1996). This ferrous tripyridyltriazine complex has an intensive blue colour and can be monitored at 593 nm. The FRAP reagent consists of 300 mM acetate buffer (pH = 3.6), 10 mM TPTZ in 40 mM HCl and 20 mM FeCl3.6H2O in the ratio of 10:1:1. Briefly, 50 μL of homogenate was added to 1.5 mL freshly prepared and prewarmed (37 °C) FRAP reagent in a test tube and incubated at 37 °C for 10 min. The absorbance of the blue-coloured complex was read against reagent blank (1.5 mL FRAP reagent + 50 μL distilled water) at 593 nm. Standard solutions of FeII in the range of 100–1000 mM were prepared from ferrous sulphate (FeSO4ċ7H2O) in distilled water. FRAP values were expressed as nmol ferric ions reduced to ferrous form/g protein (Benzie & Strain Citation1996).
Statistical analysis
All data are expressed as mean ± SEM. The analysis was performed using the SPSS statistical software package (version 21.5; SPSS, Chicago, IL). Differences among groups were tested by one-way analysis of variance (ANOVA) with the post hoc Tukey test. Probability values less than 0.05 were considered significant.
Results
Effects of carnosine on the body and liver weight
represents the effects of different treatments on the body and liver weight of the animal groups. Eight-week treatment with lead induced a significant decrease in the final body weight (p < 0.001) and increase in liver weight of the lead group compared with the control group. Carnosine prevented the change in body and liver weight of the lead-treated group; however, there were no significant differences between carnosine-treated lead groups and control animals (p > 0.05). Furthermore, carnosine did not change the body and the liver weight of the control group at the end of the experiments (p > 0.05).
Table 1. Effects of long-term carnosine administration on the final body weight (BW), liver weight (LW), serum ALT, AST, ALP, LDH, albumin and total protein (TP) in different animal groups at the end of experiments (n = 7).
Effects of carnosine on hepatic biomarkers
Lead treatment significantly increased serum ALT, AST, ALP and LDH compared with the control group (p < 0.001, p < 0.01, p <0.001 and p <0.001, respectively) (). Carnosine treatment (10 mg/kg, i.g.) caused significant reductions in the serum levels of hepatic enzymes. Furthermore, lead-induced reductions in the concentrations of albumin and total proteins (p's <0.001) were prevented by 8-week carnosine treatment. However, there were no significant changes in all the biochemical markers tested between animals treated with carnosine alone and control groups (p > 0.05).
Effect of carnosine on lipid peroxidation in liver
The concentration of lipid peroxidative product MDA in different animal groups is shown in . MDA level was significantly enhanced in the lead group (8.25 ± 0.15 nmol/mg protein) compared with the control group (3.91 ± 0.16 nmol/mg protein) (p < 0.001). Carnosine treatment reduced MDA in lead-treated rats compared with the lead group (4 ± 0.08 nmol/mg protein, 8.25 ± 0.15 nmol/mg protein, respectively) (p < 0.001). There were no significant changes in MDA between the carnosine-treated lead group and the control group (p > 0.05). Furthermore, carnosine reduced MDA in control rats (3.14 ± 0.17 nmol/mg protein) compared with control animals (3.91 ± 0.16 nmol/mg protein) (p < 0.01).
Figure 1. Effects of long-term carnosine administration on lipid peroxidation measured as hepatic malondialdehyde (MDA) content in control, lead, carnosine (10 mg/kg) treated control (Carnosine) and carnosine (10 mg/kg) treated lead (lead + carnosine) groups (n = 7) at 8 weeks after treatments. The data are represented as mean ± S.E.M. **p < 0.01 and ***p < 0.001 (as compared with the control group).
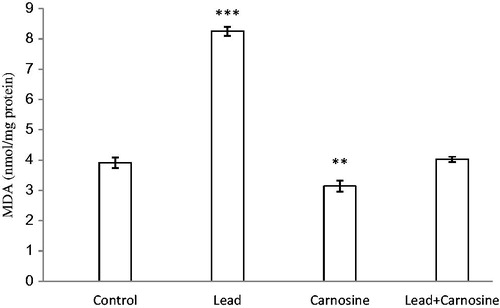
Effect of carnosine on hepatic thiol groups
Chronic lead administration caused a significant reduction in total SH groups (1.9 ± 0.22 μmol/g protein) compared with control rats (3.86 ± 0.15 μmol/g protein) (p < 0.001) (). Carnosine treatment increased total thiol concentration of liver samples in the lead group (3.25 ± 0.04 μmol/g protein) compared with the lead-treated group (p < 0.001). Carnosine also increased total thiol in the control group (4.77 ± 0.15 μmol/g protein) in comparison with control rats (p < 0.01) ().
Figure 2. Effects of long-term carnosine administration on total thiol concentration in liver homogenates samples of control, lead, carnosine (10 mg/kg) treated control (carnosine) and carnosine (10 mg/kg) treated lead (lead + carnosine) groups (n = 7) at 8 weeks after treatments. The data are represented as mean ± S.E.M. *p < 0.05, **p < 0.01 and ***p < 0.001 (as compared with the control group).
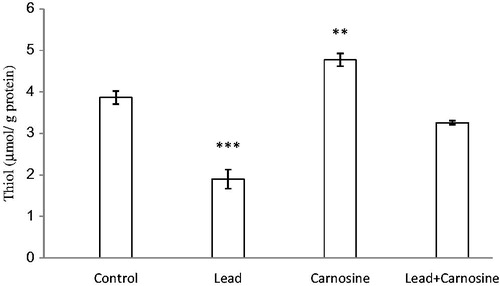
Effect of carnosine on hepatic levels of FRAP
There was a significant reduction in total antioxidant power (FRAP value) of liver homogenate in the lead group (1.72 ± 0.25 μmol/g protein) compared with controls (4 ± 0.17 μmol/g protein) (p < 0.001) (). Carnosine treatment increased FRAP values in lead (3.44 ± 0.32 μmol/g protein) and carnosine (5.18 ± 0.19 μmol/g protein) groups compared with the respective control groups (1.72 ± 0.25, 4 ± 0.17 μmol/g protein, respectively) (p < 0.001, p < 0.05, respectively) ().
Figure 3. Effects of long-term carnosine administration on antioxidant power (FRAP value) in liver homogenates samples of control, lead, carnosine (10 mg/kg) treated control (carnosine) and carnosine (10 mg/kg) treated lead (lead + carnosine) groups (n =7) at 8 weeks after treatments. The data are represented as mean ± SEM. *p<0.05, **p<0.01 and * * *p<0.001 (as compared with the control group).
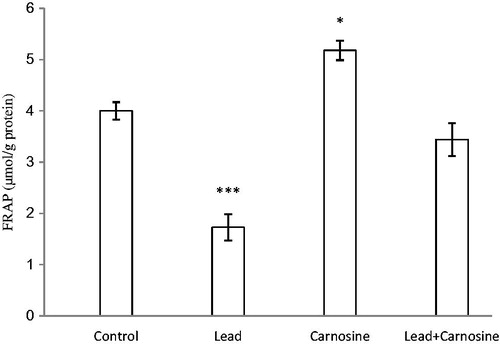
Effects of carnosine on histology changes in liver
shows the results of histopathological evaluation in different animal groups. Liver sections from control rats showed normal liver architecture, as depicted in . Lead exposure caused severe hepatic necrosis and disappearance and disarrangement of hepatic lobules structures. Degenerated hepatocytes with pyknotic nuclei, inflammatory cell infiltrations and distended portal vein in the liver were also observed (). The liver tissues of the carnosine group also showed normal histological structures (). illustrates that chronic carnosine administration showed some regions of recovery and restored the normal architecture of the hepatic lobules and the hepatocytes.
Figure 4. Paraffin sections stained by haematoxylin and eosin (H&E) for histopathological examination of liver tissues of rats as follows: (a) the control rats; (b) rats treated with lead (500 mg Pb/L in the drinking water); (c) rats fed with carnosine (10 mg/kg, i.g.) and (d) rats treated with lead (500 mg Pb/L in the drinking water) and fed with carnosine (10 mg/kg, i.g.). The long arrow indicates infiltrating leukocyte. The short arrow indicates pyknotic nucleus. The dashed arrow indicates hepatic cell necrosis. The asterisk indicates the distended portal vein.
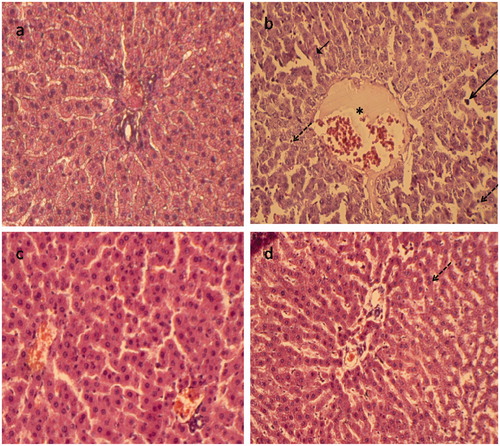
Discussion
The liver is critically damaged by acute or chronic exposure to lead. In the present study, chronic lead administration was found to cause a significant increase in ALT, AST, ALP and LDH, which are the major marker enzymes for liver function and hepatic integrity. Damage to hepatocyte membranes will cause release of these enzymes into circulation (Haleagrahara et al. Citation2010; Soliman et al. Citation2015). Also, releasing of AST, ALT and LDH from the cell cytosol can occur as secondary changes to cellular necrosis (Abdou & Hassan Citation2014). Furthermore, the decreased concentration of serum albumin and total protein could be attributed to changes in protein and free amino acid metabolism and their synthesis in the liver (Gaskill et al. Citation2005). This adverse effect might be caused by the interference of lead with protein synthesis or by the binding of lead to some metal-binding proteins and their removal through detoxification processes (Yousef Citation2004). Generally, these results indicate degenerative changes and hypofunction of liver, which confirm the earlier observations (Nehru & Kaushal Citation1993; Singh et al. Citation1994; El-Nekeety et al. Citation2009). Damage to hepatic structural integrity induced by lead acetate is further supported by our histopathological study, where the liver tissue of the animals treated with lead revealed severe destruction of hepatic architecture, necrosis, infiltration of inflammatory cells typical to those reported in the literature (Rijhsinghani et al. Citation1993; Kubo et al. Citation1996).
Evidence of oxidative stress has been detected in liver injury following lead exposure. Lead is not able to induce the production of free radicals directly, but it influences indirectly the processes of lipid peroxidation, leading to loss of membrane integrity and tissue damage (Sivaprasad et al. Citation2004; Jurczuk et al. Citation2007; Liu et al. Citation2012). In this study, chronic administration of lead resulted in an increase in lipid peroxidation as indicated by the significant increase in MDA. There was also a significant decrease in the SH group level in the liver of lead-treated rats that may be due to high ability of lead to bind with the SH group (Liu et al. Citation2012). Furthermore, TAC levels as a useful marker for demonstrating total changes in antioxidant status were decreased in the lead group.
Previous studies showed that antioxidant therapies have been shown to exert protective effects in lead-induced toxicity (Caylak et al. Citation2008; Xia et al. Citation2010; Wang et al. Citation2012). Carnosine is a natural water-soluble antioxidant which suppresses reactive oxygen species generation and scavenges lipid peroxidation products during free radical reactions (Guiotto et al. Citation2005; Mozdzan et al. Citation2005). In fact, carnosine has SOD-like activity and acts indirectly by preserving GSH which is an antioxidant itself playing an important role in reducing lipid peroxides (Fouad et al. Citation2009). In addition, carnosine also exhibits antioxidant activity by up-regulation of hepatic catalase mRNA expression (Liu et al. Citation2008). In the present study, carnosine treatment markedly decreased the content of MDA and increased SH group level in the liver of lead-treated animals. This suggested that carnosine could, at least partly, attenuate oxidative stress by decreasing MDA and increasing SH group levels. On the contrary, the measurement of TAC may be an important and useful tool in the prevention of lead-induced oxidative toxicity. In this study, carnosine treatment resulted in a significant improvement in TAC.
In the present study, carnosine (10 mg/kg) protected against liver damage induced by lead as indicated from the significant improvement in the biochemical markers of liver function as well as the liver and body weight. The protective effect of carnosine was further confirmed in the liver by the histopathological examinations. Administration of carnosine significantly ameliorated lead-induced liver tissue damage observed by microscopic examinations. The hepatoprotective effect of carnosine was demonstrated in some models of oxidative and inflammatory injuries. For example, carnosine treatment significantly suppressed lipid peroxidation, restored the deficits in the antioxidant defence mechanisms and ameliorated liver damage observed by to ischemia/reperfusion liver injury (Fouad et al. Citation2007). Furthermore, it has been reported that administration of carnosine (10 mg/kg, i.p.) for 3 d ameliorated cadmium-induced liver damage in mice (Fouad et al. Citation2009). There are also studies showing ameliorative effects of carnosine in experimental models of ethanol- (Liu et al. Citation2008), thioacetamide- (Mehmetçik et al. Citation2008) and acetaminophen-induced (Yan et al. Citation2009) hepatotoxicity, which is all in accordance with our study.
Some possible mechanisms that are responsible for the protective effects of carnosine have been reported, such as free radical scavenging ability, antiapoptotic, anti-inflammatory and the divalent metal ion-chelating activities (Gariballa & Sinclair Citation2000). Furthermore, improved microcirculation in the injured tissues by carnosine is another possible factor for its protective effects (Stvolinsky & Dobrota Citation2000).
Taken together, the present study shows that carnosine confers protection against lead-induced oxidative stress and hepatotoxicity in rats. Experimental antioxidant therapy in laboratory animals are exploring to understand the protective effects of these agents in reducing overall toxicity induced by lead, particularly hepatotoxicity. If there are promising results in experimental models, it may deserve further investigation to use them in combination with the current approved clinical interventions.
Declaration of interest
The authors report that they have no conflicts of interest.
References
- Abdou HM, Hassan MA. 2014. Protective role of omega-3 polyunsaturated fatty acid against lead acetate-induced toxicity in liver and kidney of female rats. Biomed Res Int. 435857.
- Artun BC, Küskü-Kiraz Z, Güllüoğlu M, Cevikbaş U, Koçak-Toker N, Uysal M. 2010. The effect of carnosine pretreatment on oxidative stress and hepatotoxicity in binge ethanol administered rats. Hum Exp Toxicol. 29:659–665.
- Barbosa F Jr, Fillion M, Lemire M, Passos CJ, Rodrigues JL, Philibert A, Guimarães JR, Mergler D. 2009. Elevated blood lead levels in a riverside population in the Brazilian Amazon. Environ Res. 109:294–299.
- Benzie IFF, Strain J. 1996. The ferric reducing ability of plasma (FRAP) as a measure of “antioxidant power”: the FRAP assay. Anal Biochem. 239:70–76.
- Budzeń S, Rymaszewska J. 2013. The biological role of carnosine and its possible applications in medicine. Adv Clin Exp Med. 22:739–744.
- Caylak E, Aytekin M, Halifeoglu I. 2008. Antioxidant effects of methionine, alpha-lipoic acid, N-acetylcysteine and homocysteine on lead-induced oxidative stress to erythrocytes in rats. Exp Toxicol Pathol. 60:289–294.
- Dobrota D, Fedorova T, Stvolinsky S, Babusikova E, Likavcanova K, Drgova A, Strapkova A, Boldyrev A. 2005. Carnosine protects the brain of rats and Mongolian gerbils against ischemicinjury: after-stroke-effect. Neurochem Res. 30:1283–1288.
- Ellman G. 1959. Tissue sulfhydryl groups. Arch Biochem Biophys. 82:70–77.
- El-Mehi A, Amin S. 2012. Effect of lead acetate on the thyroid gland of adult male albino rats and the possible protective role of zinc supplementation: a biochemical, histological and morphometric study. J Am Sci. 8:61–71.
- El-Nekeety AA, El-Kady AA, Soliman MS, Hassan NS, Abdel-Wahhab MA. 2009. Protective effect of Aquilegia vulgaris (L.) against lead acetate-induced oxidative stress in rats. Food Chem Toxicol. 47:2209–2215.
- Eshginia S, Marjani A. 2013. The effect of vitamin C on the erythrocyte antioxidant enzymes in intoxicated-lead rat offsprings. J Clin Diagn Res. 7:1078–1081.
- Flora G, Gupta D, Tiwari A. 2012. Toxicity of lead: a review with recent updates. Interdiscip Toxicol. 5:47–58.
- Fouad AA, El-Rehany MA, Maghraby HK. 2007. The hepatoprotective effect of carnosine against ischemia/reperfusion liver injury in rats. Eur J Pharmacol. 572:61–68.
- Fouad AA, Qureshi HA, Yacoubi MT, Al-Melhim WN. 2009. Protective role of carnosine in mice with cadmium-induced acute hepatotoxicity. Food Chem Toxicol. 47:2863–2870.
- Gariballa SE, Sinclair AJ. 2000. Carnosine: physiological properties and therapeutic potential. Age Ageing 29:207–210.
- Gaskill CL, Miller LM, Mattoon JS, Hoffmann WE, Burton SA, Gelens HC, Ihle SL, Miller JB, Shaw DH, Cribb AE. 2005. Liver histopathology and liver and serum alanine aminotransferase and alkaline phosphatase activities in epileptic dogs receiving phenobarbital. Vet Pathol. 42:147–160.
- Guiotto A, Calderan A, Ruzza P, Borin G. 2005. Carnosine and carnosine-related antioxidants: a review. Curr Med Chem. 12:2293–2315.
- Haleagrahara N, Jackie T, Chakravarthi S, Rao M, Kulur A. 2010. Protective effect of Etlingera elatior (torch ginger) extract on lead acetate-induced hepatotoxicity in rats. J Toxicol Sci. 35:663–671.
- Jurczuk M, Moniuszko-Jakoniuk J, Brzóska MM. 2007. Hepatic and renal concentrations of vitamins E and C in lead-and ethanol-exposed rats. An assessment of their involvement in the mechanisms of peroxidative damage. Food Chem Toxicol. 45:1478–1486.
- Kubo Y, Yasunaga M, Masuhara M, Terai S, Nakamura T, Okita K. 1996. Hepatocyte proliferation induced in rats by lead nitrate is suppressed by several tumor necrosis factor alpha inhibitors. Hepatology 23:104–114.
- Liu CM, Ma JQ, Sun YZ. 2012. Protective role of puerarin on lead-induced alterations of the hepatic glutathione antioxidant system and hyperlipidemia in rats. Food Chem Toxicol. 49:3119–3127.
- Liu WH, Liu TC, Yin MC. 2008. Beneficial effects of histidine and carnosine on ethanol-induced chronic liver injury. Food Chem Toxicol. 46:1503–1509.
- Mansouri MT, Cauli O. 2009. Motor alterations induced by chronic lead exposure. Environ Toxicol Pharmacol. 27:307–313.
- Mehmetçik G, Ozdemirler G, Koçak-Toker N, Cevikbaş U, Uysal M. 2008. Role of carnosine in preventing thioacetamide-induced liver injury in the rat. Peptides 29:425–429.
- Moore K, Roberts LJ. 1998. Measurement of lipid peroxidation. Free Radic Res. 28:659–671.
- Mozdzan M, Szemraj J, Rysz J, Nowak D. 2005. Antioxidant properties of carnosine re-evaluated with oxidizing systems involving iron and copper ions. Basic Clin Pharmacol Toxicol. 96:352–360.
- Nehru B, Kaushal S. 1993. Alterations in the hepatic enzymes following experimental lead poisoning. Biol Trace Elem Res. 38:27–34.
- Patra R, Swarup D, Dwivedi S. 2001. Antioxidant effects of alpha tocopherol, ascorbic acid and l-methionine on lead induced oxidative stress to the liver, kidney and brain in rats. Toxicology 162:81–88.
- Rijhsinghani K, Choi HS, Burton LA, Paronetto F, Tavoloni N. 1993. Immunoelectron microscopy identification of early proliferating cells in rat liver tissue during hyperplasia induced by lead nitrate. Hepatology 17:685–692.
- Singh B, Dhawan D, Nehru B, Garg ML, Mangal PC, Chand B, Trehan PN. 1994. Impact of lead pollution on the status of other trace metals in blood and alterations in hepatic functions. Biol Trace Elem Res. 40:21–29.
- Sivaprasad R, Nagaraj M, Varalakshmi P. 2004. Combined efficacies of lipoic acid and 2,3-dimercaptosuccinic acid against lead-induced lipid peroxidation in rat liver. Nutr Biochem. 15:18–23.
- Soliman KM, Abdul-Hamid M, Othman AI. 2007. Effect of carnosine on gentamicin-induced nephrotoxicity. Med Sci Monit. 13:BR73–BR83.
- Soliman MM, Baiomy AA, Yassin MH. 2015. Molecular and histopathological study on the ameliorative effects of curcumin against lead acetate-induced hepatotoxicity and nephrototoxicity in Wistar rats. Biol Trace Elem Res. 167:91–102.
- Stvolinsky SL, Dobrota D. 2000. Anti-ischemic activity of carnosine. Biochem Mosc. 65:849–855.
- Wang J, Yang Z, Lin L, Zhao Z, Liu Z, Liu X. 2012. Protective effect of naringenin against lead-induced oxidative stress in rats. Biol Trace Elem Res. 146:354–359.
- Xia D, Yu X, Liao S, Shao Q, Mou H, Ma W. 2010. Protective effect of Smilax glabra extract against lead-induced oxidative stress in rats. J Ethnopharmacol. 130:414–420.
- Yan SL, Wu ST, Yin MC, Chen HT, Chen HC. 2009. Protective effects from carnosine and histidine on acetaminophen-induced liver injury. J Food Sci. 74:H259–H265.
- Yousef MI. 2004. Aluminium-induced changes in hemato-biochemical parameters, lipid peroxidation and enzyme activities of male rabbits: protective role of ascorbic acid. Toxicology 199:47–57.